Determinants of Glomerular Ultrafiltration and How They are Influenced by the Glomerular Arterioles
The notion that derangements of glomerular dynamics may exert a deleterious effect and play a role in the pathogenesis of chronic kidney disease (CKD) is relatively recent, having been first postulated in a coherent way in the early 1980s. This formulation was made possible after the determinants of glomerular ultrafiltration were directly measured in micropuncture experiments, and theoretically analyzed by Brenner, Deen and co-workers in the late 1960s and early 1970s.
The ultrafiltration process at each glomerulus is governed by four independent physical determinants: the first and most important of them is the glomerular transcapillary hydraulic pressure difference, ΔP, defined by the expression, ΔP=P GC − P BS , where P GC represents the glomerular intracapillary hydraulic pressure and P BS denotes the hydraulic pressure in Bowman’s space. The second determinant is the plasma protein concentration at the afferent arteriole (C A ), which is nearly identical to the systemic plasma protein concentration and determines the oncotic force that opposes filtration. The third determinant is the initial glomerular plasma flow (Q A ); and the fourth determinant is the glomerular hydraulic conductance coefficient (K f ). Variations of ΔP and Q A cause changes in the same direction in SNGFR, whereas the relationship between SNGFR and K f is more complex: although a decrease of K f below normal lowers SNGFR, an increase of K f has limited effect on ultrafiltration. A more detailed description of these determinants, of their individual effects, and of the relationship between them is given in Chapter 21 .
Two of the determinants of ultrafiltration, ΔP and Q A , are directly affected by variations of the afferent (R A ) and efferent (R E ) arteriolar resistances, as well as by changes in systemic blood pressure. The deep influence of these vessels on the dynamics of ultrafiltration derives from the unusual arrangement of the glomerular microcirculation, with the capillary loops interposed between the afferent and efferent arteriolar resistors ( Fig. 86.1 ). If we assume the hydraulic pressure at the end of the efferent arteriole (P E ) to be much lower than the mean arterial pressure (MAP), and neglect the effect of fluid escape from the glomerular capillaries through ultrafiltration, the glomerular intracapillary hydraulic pressure (P GC ) can be approximated by:
P GC = MAP × R E / ( R A + R E )

It becomes clear from this ultra-simplified equation that P GC , and therefore ΔP, varies simultaneously with MAP and with the ratio between R E and the total glomerular arteriolar resistance. An arterial pressure elevation, with R A and R E kept constant, would be accompanied, still in accordance with Equation 86.1 , by a proportional elevation of P GC . Such situation is seldom observed, because autoregulatory mechanisms make R A change in the same direction as the change of MAP or, more specifically, of the renal perfusion pressure ( Chapter 21 , Chapter 24 ). On the other hand, changes in the glomerular arteriolar resistances can deeply influence SNGFR and its determinants. A decrease in R A , with MAP kept constant, will elevate P GC , still as predicted by Equation 86.1 , whereas the opposite will occur if R A increases. The consequences of changing the preglomerular resistance are not limited to the P GC , since in a hydraulic system consisting of series resistors, such as the glomerular microcirculation, variations of one of the resistances will always promote reciprocal variations of flow. Specifically, Q A can be approximated by the expression:
Q A = MAP / ( R A + R E ) × ( 1 − Ht )
where Ht denotes the arterial hematocrit. Thus, a decrease of R A will augment Q A , while the reverse will occur if R A increases. Since as noted above an analogous relationship exists between R A and P GC , and since SNGFR is a direct function of both Q A and ΔP, it follows that variations of R A always promote changes of SNGFR in the opposite direction.
The effect of R E on SNGFR is more complex than that of R A , because of the peculiar location of the efferent resistor. An increase of R E tends to increase P GC (and ΔP), approaching its value to that of MAP, as predicted by Equation 86.1 . Conversely, a decrease of R E tends to bring P GC close to zero, or more precisely, to the low pressure of the postglomerular microcirculation. On the other hand, an increase of R E , as in the case of R A , tends to diminish Q A , as predicted by Equation 86.2 , while the opposite will be seen with a decrease in R E . Thus changes of R E promote opposite effects in P GC (and ΔP) and Q A . If R E is brought to very low values, ΔP may fall to critical levels (close to the plasma oncotic pressure), reducing SNGFR to values that approach zero, regardless of the value of Q A . As R E increases above these critically low values, SNGFR rises very steeply, since the positive effect on P GC (and ΔP) largely compensates its initially small negative effect on Q A . This effect reaches a maximum at R E values that are within the normal range, since further efferent vasoconstriction will promote no additional increase of SNGFR and may even diminish it, because Q A will be reduced in such a way as to offset the elevation of P GC .
In view of the above considerations, it becomes clear that the pre and postglomerular resistors exert quite different functions. The monotonic inverse relationship between R A and the SNGFR renders the afferent resistance ideally suited to function as a regulator of the glomerular microcirculation. Indeed, variations of R A can entirely account for renal autoregulation , which keeps GFR, renal plasma flow and ΔP within narrow ranges in the face of wide variation of systemic blood pressure. Although variations of R E appear unsuited to intervene in the process of regulation of the glomerular microcirculation, the efferent arteriole is essential for the glomerular ultrafiltration process to occur. The mere existence of the efferent arteriole, acting as a “postcapillary sphincter,” allows the glomerular capillaries to maintain much higher hydraulic pressures than in other microcirculatory territories, which is a physical requirement for high-grade ultrafiltration to occur. If R E is decreased to critically low values, which may happen for instance in patients with bilateral renal artery stenosis treated with inhibitors of the renin-angiotensin system or angiotensin II receptor blockers, a catastrophic fall of SNGFR occurs, and acute renal failure can ensue.
The GFR is Fairly Constant Under Physiologic Conditions—But There are Exceptions
Under physiologic conditions, there is little need for the GFR to vary very much, since the balance of water and solutes such as sodium, chloride and potassium is achieved by the action of the tubules, especially at the final portions of the nephron. In fact, GFR is tightly controlled by autoregulatory mechanisms, which involve mostly variations of the afferent arteriolar resistance, more suitable to this task, as pointed out earlier. These variations of R A are thought to be achieved by local myogenic reflexes and by signals generated at the macula densa.
In states of decreased effective arterial volemia such as in dehydration, the GFR can be brought to very low values, even in the absence of kidney injury, as part of the organism’s effort to conserve salt and water. Such an effect is accomplished by arteriolar vasoconstriction and mesangial cell contraction, with consequent reduction of K f .
In contrast with the promptness with which GFR can be reduced whenever necessary, there is usually little need to promote a substantial increase of the GFR. However, there are a few notable exceptions to this rule. Expansion of the extracellular volume, besides promoting tubular rejection of fluid and electrolytes, can increase GFR through the action of atrial natriuretic peptides. Ingestion of a single protein-rich meal can acutely increase the GFR by 30–50% in humans and in laboratory animals, an effect that can be replicated by intravenous infusion of amino acids in rats, and that is thought to represent an adaptation to the need to excrete the protein catabolites more readily. A sustained increase of GFR, of similar magnitude as in the acute experiments, can be obtained by chronic feeding of a high-protein diet. This change in GFR involves approximately proportional dilatation of both the afferent and efferent arterioles and consequent augmentation of Q A , without elevation of P GC and ΔP, as predicted by Equation 86.1 . Normal pregnancy is another physiologic situation characterized by augmented GFR. As with high-protein feeding, the GFR increase observed during pregnancy is due to commensurate decreases of R A an R E , resulting in increased Q A without elevation of ΔP. These changes are entirely reversible, but will recur with every subsequent pregnancy in the same individual, with no cumulative effect.
Single Nephron Hyperfiltration is a Prominent Feature of Chronic Kidney Disease
From the original description of chronic kidney disease (CKD) by Richard Bright in the XIXth century until the 1950s, it was generally believed that the kidneys affected by chronic disease simply ceased to function as a whole, thereafter becoming essentially unable to maintain homeostasis or to adapt to that unfavorable condition. In 1952, Robert Platt proposed that the functional disturbances encountered in renal disease result from progressive reduction in the number of functioning nephrons rather than from the separate impairment of glomerular or tubular functions of all nephrons. He suggested that, as the number of nephrons diminishes, the remaining units undergo functional hypertrophy, in such a way that impairment of renal function is easily overlooked initially, becoming apparent only at relatively advanced stages of the disease. Bricker and colleagues carried this concept a step further by showing that, in an experimental model of CKD, glomerular and tubular functions decreased in parallel, but function per nephron was increased, suggesting that whole nephrons were indeed disappearing as functional units, forcing the surviving nephrons to undergo vicarious hypertrophy. Bricker and coworkers coined the expression intact nephron hypothesis to define this concept, which has survived, with some modifications, up to the present day.
In the 1920s and 1930s, Addis had already postulated that increased “workload” to the kidneys, originating from excess protein intake and/or unilateral kidney removal, led to compensatory functional and structural hypertrophy of the remaining renal tissue. The nephron hypertrophy implied by the intact nephron hypothesis was in keeping with this notion of compensatory whole kidney hypertrophy. However, it was not until the early 1980s that a detailed physiologic basis was constructed to explain the mechanisms by which this nephron adaptation might take place. In 1981, Hostetter, Rennke and Brenner demonstrated that five-sixths renal ablation (by removal of the right kidney and infarction of two thirds of the contralateral one), standardized a few years earlier by Shimamura as a model of progressive glomerulopathy, promoted a striking increase of SNGFR, confirming the earlier predictions of compensatory nephron hyperfiltration upon loss of renal parenchyma. These investigators went on to demonstrate that hyperfiltration was due to the combination of systemic hypertension with a pronounced dilatation of the glomerular microvessels, especially the afferent arteriole. As a consequence of this predominant decrease of R A , and in keeping with Equations 86.1 and 86.2 , there were marked increases of Q A , P GC and ΔP, leading to a corresponding increase of SNGFR, partially offset by a mild reduction of K f . Similar results were later reported by the same group in a different model of glomerular injury, the DOC-salt model, in which uninephrectomy is associated with dietary salt overload and mineralocorticoid excess.
Glomerular Hyperfiltration in Diabetes Mellitus
Renal mass reduction is not the only pathologic instance in which SNGFR is chronically increased. In type 1 diabetic patients not yet afflicted by the long-term complications of the disease, but in which metabolic control is poor, GFR tends to increase by 30 to 40% compared to the normal population, an abnormality that is accompanied by a proportional increase in kidney size. Renal hypertrophy involves mostly the enlargement of glomeruli, whose size may double, although tubular hypertrophy is also prominent in these subjects. Although the mechanisms responsible for the renal hypertrophy in diabetes are presently unknown, a physiological basis for hyperfiltration in this setting is well established. In 1981, Hostetter and coworkers showed that in rats made diabetic with streptozotocin there is vasodilatation of both the afferent and efferent arterioles, with ample predominance of the former, in a similar manner as in compensatory single nephron hyperfiltration. As a consequence, both Q A and ΔP are increased, thus promoting hyperfiltration even in the absence of systemic hypertension. These findings were later confirmed in the same laboratory and elsewhere. In diabetic patients, hyperfiltration and hyperperfusion can be easily demonstrated, but direct determination of P GC is not possible. However, indirect estimates obtained from measurement of filtration fraction suggest that glomerular hypertension does accompany human diabetes as well.
A large body of evidence indicates that glomerular hyperfiltration also occurs in patients with type 2 diabetes mellitus; although its prevalence is lower than among type 1 diabetic patients, and its very existence was questioned in some earlier reports. Hyperfiltration has also been shown in experimental models of type 2 diabetes. It must be noted that analysis of renal hemodynamics in these patients is clouded by the concomitant occurrence of cardiovascular abnormalities, which tend to compromise large arteries, including the renal arteries and their branches, along with the cardiac function. In addition, the exact time of onset of type 2 diabetes is often uncertain, allowing diabetic and/or ischemic nephropathy to develop, and GFR to decline substantially from initially elevated levels.
The “Hyperfiltration Hypothesis” and the Progressive Nature of Chronic Kidney Disease
The consistent finding that nephron hyperfiltration antecedes, and even predicts, the development of renal injury in clinical and experimental CKD prompted the hypothesis, formulated by Brenner and coworkers, that a causal relationship existed between abnormal glomerular hemodynamics and the development of progressive glomerular damage. In the original version of this hyperfiltration hypothesis , it was postulated that the presence of heightened flows and pressures at the glomerular capillaries would promote mechanical aggression to the fragile capillary walls, triggering a process of progressive injury of the glomerular tuft that would eventually result in its complete destruction. In addition, the concept of compensatory nephron hyperfunction implied that glomerular dropout would impose an ever-increasing burden on the remaining units, aggravating the existing mechanical stress and closing a vicious circle that would perpetuate the process of renal parenchymal destruction.
Besides offering a plausible explanation for the well-known progressive behavior of CKD, the hyperfiltration hypothesis formulated in the early 1980s fitted well with a series of findings reported elsewhere. A few years earlier, Steffes and coworkers had demonstrated that the severity of diabetic nephropathy was aggravated in rats also subjected to uninephrectomy, presumably through exacerbation of hyperfiltration in the remaining kidney. In a prior study of the same laboratory, it had been shown that in diabetic rats subjected to unilateral renal artery clipping, diabetic nephropathy was prevented in the clipped side, and aggravated in the contralateral kidney. Accordingly, ipsilateral protection from diabetic nephropathy in patients with unilateral renal artery stenosis has been reported.
The practical implications of the hyperfiltration hypothesis were evident, since it opened the tangible possibility that chronic damage to the renal parenchyma by diabetes or progressive nephron loss could be treated by maneuvers aimed at lessening the associated abnormalities of glomerular dynamics. A few seminal studies developed by Brenner and associates showed that this goal was indeed attainable. Hostetter et al. showed that the early structural abnormalities associated with 5/6 nephrectomy in rats were abrogated by administration of a low-protein diet, a maneuver that normalized SNGFR, Q A and ΔP. Zatz and associates obtained similar amelioration of glomerular dynamics, and equal renoprotection, in diabetic rats that received a low-protein diet for one year. These observations appeared to confirm the hyperfiltration hypothesis and, at the same time, the possibility of changing the natural course of CKD by measures distinct from treatment of hypertension or the metabolic disturbance of diabetes, then the only therapeutic resources available.
Glomerular Hypertension is the Relevant Pathogenic Factor Linking Hyperfiltration to Glomerular Damage
Although the hyperfiltration hypothesis initially seemed to explain convincingly the clinical and experimental findings obtained theretofore, it was soon challenged by a series of apparently inconsistent observations. The predictive value of GFR in relation to diabetic nephropathy was not confirmed in a number of clinical studies. In addition, long-term observations in subjects that had undergone uninephrectomy showed only mild and non-progressive proteinuria and arterial hypertension, or even no abnormality at all, compared with the general population. Likewise, no renal injury was associated even with multiple successive pregnancies in rats, in which GFR is expectedly increased, a finding in keeping with clinical observations in multiparous women. Other studies showed that it was possible to ameliorate glomerular injury in the 5/6 renal ablation model without reducing GFR. Other attempts at preventing the progression of CKD, though successful, were not entirely consistent with the hyperfiltration hypothesis. In rats subjected to five-sixths nephrectomy and treated with the then brand new ACE inhibitor, enalapril, the associated glomerulosclerosis was largely prevented. However, enalapril failed to normalize GFR or Q A , although it did normalize glomerular pressure. Similar results were subsequently reported in the streptozotocin-diabetes model, and confirmed elsewhere. In view of these results, it was no longer possible to bundle together glomerular hyperfiltration, hyperperfusion and hypertension as a pathogenic mechanism of chronic glomerular injury. It became necessary to modify the hyperfiltration hypothesis to reconcile it with the evidence that glomerular hypertension, rather than the hypertension/hyperperfusion/hypertension trio, represented the actual physical mechanism promoting long-term glomerular capillary injury. The designation “Hyperfiltration hypothesis,” though still widely employed, should therefore be replaced by more precise expressions such as “Hemodynamic hypothesis,” or “Hemodynamic theory.”
The Importance of Glomerular Hypertrophy and the Concept of Mechanical Aggression
During the second half of the 1980s, a series of clinical and experimental observations showed that enlargement of the glomerular tuft, a “nonhemodynamic” abnormality, was consistently associated with the development of progressive glomerular injury. Several of these studies suggested that the development of glomerulosclerosis was more closely associated with glomerular hypertrophy than with glomerular hypertension. A study in proteinuric pediatric patients showed that glomerular size predicted the development of focal and segmental glomerulosclerosis, while several experimental observations showed either the development of glomerulosclerosis without glomerular hypertension or the converse. These observations gave rise to a “hypertrophy theory,” which was initially regarded by many as a better alternative to the hemodynamic theory. However, evidence obtained during subsequent years failed to lend support to this “hypertrophy theory.” Some of these studies corroborated the earlier observations of a tight association between glomerular hypertension and glomerular injury. In others, the relationship between glomerular hypertrophy and glomerular injury was rather inconsistent. However, it became clear, in the years that ensued, that the hypertrophy and hemodynamic theories were not at all irreconcilable, and that the two mechanisms must act in concert, rather than separately, to promote glomerular injury. The physical basis for such interaction becomes apparent when we consider the local forces that act on the glomerular filtration surface, shown in simplified form in Fig. 86.2 . The presence of a transcapillary hydraulic pressure difference, ΔP, imposes a continuous mechanical stretch to the glomerular wall. According to Laplace’s law, the wall tension, T, equals the product of the pressure gradient, ΔP, and the capillary radius, R. An elevation of ΔP will promote a proportional increase of T and, therefore, of the mechanical stretch to which the components of the glomerular wall are subjected. On the other hand, enlargement of the glomerular tuft will increase the capillary radius, R, augmenting T in exactly the same way as would an elevation of ΔP. Thus, while glomerular hypertension can conceivably promote capillary damage in the absence of tuft hypertrophy, mechanical injury to the glomerular capillary wall can in principle occur without elevation of ΔP. Accordingly, it is hardly surprising that the association of the two abnormalities can accelerate glomerular injury.

Cellular Mechanisms by Which Mechanical Stretch Can Injure the Glomerular Wall
Stretching of the glomerular capillary wall by intracapillary hypertension or tuft hypertrophy would be expected to promote only localized, non-progressive injury if the resulting physical forces were the only factors at operation. However, the glomerular tuft is not constituted solely by inert elements such as collagen fibers: injury to endothelial cells, mesangial cells and podocytes trigger a series of events that together conspire to extend and amplify the initial mechanical aggression, as will be discussed in the paragraphs that follow.
Endothelial cells are sensitive to mechanical strain, undergoing changes of shape and increasing their rate of proliferation when exposed to axial stretch in vitro . Subjecting these cells to prolonged strain in vivo may result in severe damage, which can even promote disintegration or detachment, leading to local denudation of the glomerular basement membrane, with consequent exposure of extracellular matrix constituents to the circulatory stream. The resulting activation/aggregation of platelets, with fibrin accumulation, may favor the formation of intracapillary microthrombi, leading to luminal obliteration. With subsequent organization of this material, the glomerular tuft can become partially or totally fibrotic. Microaneurysms, which have been reported in several experimental models characterized by severe glomerular hypertension, may undergo a similar process of organization.
The mesangial cells possess a complex cytoskeleton that may be affected by mechanical stretch transmitted from the capillary filtration surface. A number of in vitro experiments have brought evidence that, when subjected to such strain, cultured mesangial cells undergo a series of phenotype changes, increasing their proliferative activity and synthesizing a number of molecules involved in inflammatory phenomena—cytokines, growth factors, adhesion molecules and cyclooxygenase products. In addition, mechanical stretch stimulates the production of collagen fibers, thus favoring the development of fibrosis and contributing to the progressive destruction of the glomerular tuft. Heightened intracapillary pressure can promote additional mesangial injury by driving circulating macromolecules, including immunoglobulins and complement components, into the mesangial region, where they may accumulate, thus contributing to irritate the mesangial cells and to maintain an inflammatory environment at a local level. Such movement is facilitated by the fact that the mesangial region is not covered by glomerular basement membrane, being separated from the circulatory stream only by the fenestrated endothelial layer.
Among the native glomerular cells, the podocytes are perhaps the most likely to suffer phenotypic changes upon exposure to mechanical strain. These cells lie amidst a very complex molecular setup, with a myriad of intricate connections between cytoskeleton constituents, such as actin, myosin and α-actinin, and those of the slit membrane, such as nephrin and p-cadherin. Conceivably, any disturbance of such a delicate arrangement can disrupt this complicated structure, leading to simplification of the foot processes, rupture of the slit membrane, and impairment of the glomerular barrier function, with ensuing proteinuria. Because podocytes are terminally differentiated cells, they possess limited proliferative capacity, being unable to adapt to the increased capillary dimensions imposed by glomerular hypertension and/or hypertrophy. Thus, the inevitable mechanical strain associated with these conditions can promote podocyte rupture, death by apoptosis or necrosis, and detachment from the basement membrane, resulting in denudation of its outermost aspect. The latter is likely to favor the fixation of the glomerular tuft to the parietal layer of Bowman’s capsule, leading to the formation of synechiae, from which a local process of sclerosis may arise and spread to a glomerular segment, to the whole tuft, and/or to the adjacent interstitium. Additional damage can result from leakage to the interstitium, through this adhesion spot, of plasma ultrafiltrate, with limited hindrance to the passage of proteins, given the local absence of foot processes or slit membranes. This “misdirected filtration” ( Fig. 86.3 ) may lead to accumulation of fluid relatively rich in protein at the vicinity of the glomerulus or, if abundant enough, around extensive parts of the respective tubular segments. Inflammation and subsequent organization of this initially amorphous layer may lead to ensheathing of substantial parts of that particular nephron, which can eventually be sectioned by the fibrotic process, and even disintegrate.

As mentioned earlier, mechanical strain of the podocyte layer may lead to disruption of the glomerular filtration barrier, with the passage of large amounts of protein to the urinary space and the appearance of proteinuria, a well-known hallmark of glomerular injury. As a consequence, the protein reabsorption rate of the proximal tubular cells increases markedly, in an attempt at recovering the filtered protein, thus limiting the urinary protein loss. To perform this task, cells must digest the reabsorbed protein, intensifying the formation of endosomes and the synthesis of proteolytic enzymes. Several investigators believe that prolonged exposure of proximal tubular cells to this situation can trigger a process of interstitial fibrosis, which contributes strongly to accelerate the progression of the nephropathy. A number of clinical studies support this hypothesis by showing a direct relationship between the proportion of patients with glomerulopathies that progress to end-stage renal disease and the level of proteinuria at the time of diagnosis.
Although other investigators note that these findings could merely reflect a natural association between the intensity of proteinuria and the severity of the underlying glomerular disease, numerous experimental studies have provided more direct evidence that abnormal passage of proteins to the urinary space can indeed favor interstitial inflammation and fibrosis. When cultured in albumin-rich medium, proximal tubular cells produce significant amounts of cytokines and chemokines. Accordingly, interstitial fibrosis, inflammation, and markers of oxidative stress were found in association with heavy proteinuria in congenital nephrotic syndrome. Together, these findings indicate that glomerular injury with impairment of the filtration barrier can be indirectly propagated to the interstitium, amplifying the resulting renal damage. However, there is evidence that this mechanism may be of limited importance or may become active only at advanced stages of CKD. In two experimental models of proteinuric CKD, Kriz showed that chronic injury was confined to individual nephrons, rather than being disseminated, as would be expected if increased glomerular passage of proteins were a major pathogenic factor in this setting. In addition, Theilig and coworkers studied mutant mice in which proximal reabsorption of protein was severely limited due to deficient expression of megalin, an essential element for protein endocytosis to work properly. Although the presence of inflammatory markers did associate with protein uptake, tubulointerstitial injury correlated with glomerular damage to the parental glomerular tuft, rather than to the local inflammatory activity. Thus, despite evidence favoring the inflammatory effect of filtered protein, the role of impairment of the glomerular barrier in the pathogenesis of interstitial fibrosis in CKD remains open to question.
From Stretch-Induced Injury to Progressive Nephropathy: the Role of Inflammation
As remarked earlier, progressive nephron loss promotes or aggravates mechanical strain in the remaining units, thus contributing to the establishment of a vicious cycle that leads to further nephron destruction. An additional crucial factor for CKD perpetuation is the presence of inflammatory events, a common denominator to all cellular mechanisms discussed in the preceding paragraphs. The participation of inflammatory phenomena in the pathogenesis of nephropathies of immunologic injury has been known for decades. Tissue infiltration by macrophages and fibroblasts has been regarded as a natural follow-up of such events as activation of T-lymphocytes and of the complement cascade. Evidence obtained since the late 1980s in human nephropathies and, especially, in experimental models of CKD, showed that these phenomena are also important as promoters of chronic renal injury not mediated by immune mechanisms, especially those induced by mechanical strain. The presence of inflammatory cells has been described in several models of progressive renal injury, such as streptozotocin-induced diabetes mellitus, aging nephropathy, chronic NO inhibition, experimental nonimmune nephrotic syndrome and protein-overload proteinuria.
Floege and coworkers reported that, in rats with 5/6 renal ablation, by far the most widely utilized CKD model, renal interstitial lymphocyte infiltration, activated by unknown mechanisms, can be observed a few days after renal mass removal, when sclerosing glomerular lesions are still scarce. In the following days, the renal interstitium is progressively invaded by macrophages, while the intensity of the lymphocyte infiltration decreases. These findings were subsequently corroborated in other laboratories. In addition, a surge of cell proliferation, mostly located at the tubulointerstitial compartment, is noted in these rats a few days after renal ablation, decreasing after two weeks, but persisting for at least two months. The identity of these cells is uncertain, although it is conceivable that part of this proliferative activity may be related to the generation of myofibroblasts from either interstitial fibroblasts or tubular cells, the latter through epithelial-to-mesenchymal transdifferentiation.
The recruitment of inflammatory cells into the renal parenchyma, and the proliferation of resident glomerular and tubular cells are crucially dependent on the production of inflammatory mediators. The presence in the renal parenchyma of a number of cytokines, chemokines and growth factors, such as interleukin-1, RANTES, fractalkine, TGF-β, MCP-1, TNF-α, and adhesion molecules, among many others, have been associated with several clinical conditions and experimental models of CKD, along with evidence of activation of the intricate and often redundant intracellular pathways involved in the synthesis of these molecules. An extensive analysis of the participation of these systems in the pathogenesis of CKD would transcend the scope of this chapter. Additional discussion of this subject can be found in Chapter 82 , Chapter 87 , Chapter 90 .
The importance of inflammatory phenomena in the pathogenesis of CKD is further highlighted by the protective effect exerted by anti-inflammatory agents. Fujihara and coworkers reported that concomitant administration of mycophenolate mofetil (MMF), a specific inhibitor of lymphocyte proliferation, strongly attenuated renal inflammation and prevented glomerular and interstitial injury in the remnant kidney model, a finding also reported, almost simultaneously, by Romero and coworkers. Renal protection by MMF monotherapy was also described in streptozotocin-induced diabetic rats and in the chronic NO inhibition model, and was strongly enhanced by association with a depressor of the renin-angiotensin system. Interestingly, administration of nonsteroidal anti-inflammatories exerted a protective effect in the remnant kidney model and in experimental diabetes mellitus. However, this class of drug is contraindicated in CKD due to the risk of precipitating a catastrophic fall of GFR (see below).
The Immense Pathogenic Importance of Angiotensin II in CKD
As detailed in Chapter 15 , Ang II is a potent vasoconstrictor agent, which binds to the AT-1 receptor, abundant in smooth muscle and mesangial cells. By virtue of its vascular effects, Ang II contributes to the maintenance of blood pressure, especially in states of dehydration. This effect is strongly reinforced by the action of Ang II on sodium conservation, as a result of its effects on both the nephron itself, enhancing sodium reabsorption, and the adrenals, stimulating the release of aldosterone. At the glomerular microcirculation, Ang II constricts both the afferent and efferent arterioles. However, this vasoconstrictor effect was shown to be disproportionally intense at the efferent arteriole, a finding confirmed by in vitro studies of isolated afferent and efferent arterioles. The reasons for this asymmetry are unclear, and may be related to the finding that Ang II stimulates vasoconstriction of the afferent arterioles through activation of voltage-gated (L-type) calcium channels, whereas Ang II–mediated vasoconstriction of the efferent arteriole depends mainly on mobilization of calcium from intracellular stores. Ang II also promotes contraction of the mesangial cells, with a consequent reduction of the glomerular K f . The predominant efferent vasoconstriction leads to an elevation of P GC . However, since K f decreases, and since Q A is depressed due to the arteriolar vasoconstriction, the SNGFR remains relatively constant during Ang II infusions, with consequent increase of the filtration fraction (FF). Recent evidence has shown that the renin-angiotensin system is much more complex than originally thought, and that novel components of this system may have physiologic importance. Angiotensin 1–7, a heptapeptide generated by the action of angiotensin I converting enzyme 2 (ACE2), exerts a vasodilatory effect and may modulate the action of Ang II. Likewise, activation of the AT2 receptor by Ang II can initiate a series of events that culminate in vasodilatation and natriuresis, thus antagonizing the vasoconstrictor/antinatriuretic effects elicited by Ang II binding to the AT1 receptor. However, the exact physiologic role of angiotensin 1–7, the AT2 receptor and other, less known components of the renin-angiotensin system is presently unclear.
Chronic nephron loss tends to promote extracellular expansion due to a natural limitation to sodium excretion. In this setting, there is a trend toward renal vasodilatation, while the circulating levels of renin and angiotensin II tend to be depressed, reflecting inhibition of renal renin release to the circulation. On the other hand, abundant evidence has been amassed in the past two decades that a large number of organs and tissues, including the kidneys, possess all the components of the renin-angiotensin system, being able to produce Ang II locally in an independent fashion. The combination of renal arteriolar vasodilatation with local production of Ang II, which as noted above acts preferentially on R E , may result in disproportionate decrease of R A , leading to simultaneous increases of Q A and ΔP and, as a result, to single nephron hyperfiltration.
In diabetes mellitus, the circulating levels of renin and angiotensin II tend to be reduced, which might favor hyperperfusion and hyperfiltration in these individuals. On the other hand, there is evidence that the renin-angiotensin system may be activated at the intrarenal level in early experimental diabetes, which may help to explain the relatively modest fall in R E observed in this condition, which favors, as we have seen, the elevation of P GC , hence glomerular injury. The reasons for the local and systemic derangement of the renin-angiotensin system in diabetes mellitus are yet to be elucidated.
The intrinsic action of Ang II on glomerular hemodynamics in CKD and in early diabetes mellitus is unveiled by the striking effect of treatment with angiotensin-converting enzyme inhibitors (ACEIs) or angiotensin II receptor type 1 blockers (ARBs), which promote dilatation of afferent and efferent arterioles, as well as normalization of glomerular pressure, without significant changes of SNGFR. Thus it is beyond doubt that Ang II exerts a pathogenic effect on the glomerulus, which may favor the installation of tuft injury by the mechanisms discussed above. However, the scope of action of Ang II is now known to largely transcend the hemodynamic sphere, characterizing this octapeptide as a potent proinflammatory cytokine. Chronic systemic Ang II infusion in rats was shown to promote not only arterial hypertension, but also glomerular and interstitial infiltration by mononuclear cells and severe renal fibrosis. Ang II was subsequently shown to influence cell proliferation, and the synthesis of a number of cytokines, chemokines, growth factors and adhesion molecules, all of which exert an obvious pathogenic impact on the progression of CKD, such as RANTES, MCP-1, osteopontin, tumor necrosis factor-α (TNF-α), CTGF and PDGF, among others. These actions are largely mediated by Ang II binding to the AT1 receptor, which stimulates several intracellular pathways linked to inflammation, especially the NF-κB cascade and the MAPK and Stat/Jnk pathways. One of the main proinflammatory effects of Ang II is mediated by its strong stimulation of TGF-β, a well-known promoter of fibrosis in the renal tissue, as well as in other territories. Furthermore, in vitro exposure of mesangial cells to Ang II can increase the production of superoxide anions and induce oxidative stress, a well-known promoter of inflammation. Likewise, in vivo Ang II infusion enhances the production of oxidation markers such as thiobarbituric acid–reactive substances and protein carbonyl, as well as 8-iso prostaglandin F2α. Ang II can further contribute to CKD by one of its classical nonhemodynamic actions, the production of aldosterone by the adrenals. Aldosterone has been shown to increase collagen IV synthesis by mesangial cells, and to facilitate the progression of CKD in the renal and myocardial tissue, independent of Ang II. Plasma aldosterone concentrations are strikingly elevated in partially nephrectomized rats. Conversely, mineralocorticoid receptor blockade, alone or associated with ACE inhibitors or Ang II receptor blockers, reduces glomerulosclerosis and proteinuria in several CKD models, further suggesting a pathogenic role for the stimulation of the mineralocorticoid receptor. Ang II can provide additional contribution to renal damage by increasing glomerular permeability, thus promoting tubulointerstitial damage through the mechanisms discussed earlier. An important evidence of the pathogenic role of Ang II is the demonstration, by immunohistochemistry and/or by immunofluorescence, that Ang II associates with infiltrating cells at inflamed areas of the renal interstitium in several models of CKD, as well as in biopsy material obtained from CKD patients. In these studies, the intensity of tissue infiltration by Ang II-positive cells correlated directly with the extent and severity of interstitial inflammation. A more direct link between Ang II and glomerular mechanical strain was unraveled by the finding that, when subjected to cyclical stretching, mesangial cells upregulate components of the renin-angiotensin system. Thus, the glomerular action of Ang II may lead to a vicious cycle, in that Ang II elevates P GC , which promotes mechanical stress, stimulating further Ang II production and setting in motion its many pro-inflammatory actions.
Given the plethora of hemodynamic and nonhemodynamic actions of Ang II, it is far from surprising that treatment with ACE inhibitors or Ang II receptor blockers prevents or attenuates renal injury in several forms of clinical and experimental CKD. Although several promising novel therapeutic strategies have been proposed in the past two decades, administration of depressors of the renin-angiotensin system remains a mainstay of the therapy of CKD, along with diuretics, other antihypertensives and glycemic control in diabetics. However, CKD patients who receive these drugs still progress to ESRD, albeit at a slower rate, particularly when treatment is initiated at advanced stages of the disease. The reasons why the protection offered by these drugs is incomplete are obscure. It is likely that several of the complex and interrelated intracellular pathways that culminate in the synthesis of inflammatory mediators are activated by physical and chemical events distinct from the Ang II-AT1 interaction, such as oxidative stress and the binding of a number of cytokines and growth factors to specific receptors. Of particular interest is the recent discovery that prorenin, once regarded as an inert precursor of renin, binds to a specific receptor, undergoing a nonproteolytic conformational change that enables prorenin to cleave angiotensinogen to angiotensin I. In addition, binding of prorenin to this receptor activates several intracellular proinflammatory pathways. This receptor can also be activated by renin through the binding of an epitope shared with prorenin. For this reason, this receptor became known as the (pro)renin receptor. Recent evidence suggests that blockade of this receptor with a specific antagonist prevents the progression of experimental diabetic nephropathy, suggesting that this may become a new strategy in the management of CKD.
The Role of Other Vasoactive Compounds in Glomerular Mechanical Strain
Circulating Catecholamines and Renal Sympathetic Innervation
As with Ang II, the glomerular microcirculation is exquisitely sensitive to the vasoconstrictor activity of the sympathetic system and of its circulating mediators. Different from Ang II, however, norepinephrine induces vasoconstriction of both afferent and efferent arterioles, an effect thought to be mediated by α-adrenergic receptors and to depend at least in part on variations of intracellular calcium, especially at the efferent arterioles. Acute catecholamine action results in systemic hypertension and, given the proportionate increases in pre- and postglomerular resistances, a corresponding elevation of glomerular pressure. Because the overall resistance of the glomerular microcirculation increases, glomerular blood flow is diminished. The net result of these changes is, as observed with Ang II, maintenance of GFR at relatively constant levels. In addition to its direct effect on the glomerular vessels, the renal sympathetic inflow has been long known to stimulate renin exocytosis from the juxtaglomerular cells through activation of β1-adrenergic receptors, an effect that enhances the sympathetic action on blood pressure, sodium reabsorption, and the glomerular microcirculation, and can contribute to cause glomerular injury through the mechanisms discussed above. Recent studies indicate that sympathetic activity and, in particular, the sympathetic inflow to the kidneys, are enhanced in CKD. Increased renal sympathetic stimulation has been implicated in the pathogenesis of hypertension in CKD and in the remnant kidney model, while renal denervation has been shown to lower systemic blood pressure in murine hypertension, underlining the important influence exerted by the sympathetic innervation on the renal vasculature and on sodium reabsorption. Additional complexity derives from the finding of two types of sympathetic nerve fibers in the kidney, with differential innervation of the afferent and efferent arterioles.
Nitric Oxide
Nitric oxide (NO) was originally identified about a quarter century ago as a powerful endogenous vasodilator produced from L-arginine by endothelial cells, under the action of NO synthases (NOS), and soon proven to be responsible for the vasodilator effect of compounds such as acetylcholine, histamine and bradykinin. Besides being a potent vasodilator, NO inhibits cell proliferation and platelet aggregation, which helps to preserve the endothelial integrity. Although NO was originally described as a product of the endothelial cells, it became soon clear that it can be synthesized by mesangial cells, smooth muscle cells, tubular cells and leukocytes, among other cell types. Because NO is very diffusible and highly reactive, it quickly combines with other free radicals or is taken away by hemoglobin. In view of these characteristics, which are shared by other reactive species such as superoxide, NO has a short-lived effect, confined to the immediate surroundings of the cells in which it is produced, and must be generated uninterruptedly if it is to play any significant physiologic role.
A number of experimental studies suggest that continuous NO production is fundamental for the circulatory system to function properly. Acute intravenous administration of unselective NOS inhibitors promotes a quick and marked elevation of systemic blood pressure, in addition to a pronounced constriction of renal microvessels, while P GC rises and Q A and K f are decreased. As a result of these opposing changes, the SNGFR is little affected. These findings are strongly reminiscent of the effect of acute Ang II infusions, and suggest that NO may be needed as a modulator of the effect of Ang II and other vasoconstrictors. The individual role of the main isoforms of NOS is unclear. Acute selective inhibition of neuronal NOS (nNOS), which is strongly expressed at the macula densa, lowers GFR without affecting blood pressure or renal blood flow. Parallel results were obtained after perfusion of the macula densa with a NO synthase inhibitor. These findings suggest a regulatory role for nNOS. However, chronic nNOS inhibition was reported to raise blood pressure without changing GFR. Inhibition of the inducible isoform of NO synthase (iNOS) has not been reported to promote consistent changes of renal or systemic hemodynamics under physiologic conditions, being more closely related to the operation of the innate immunity and of inflammatory mechanisms. The current evidence suggests that the endothelial NOS isoform (eNOS) accounts for most of the modulation of renal and systemic hemodynamics ascribed to NO.
Chronic inhibition of NO production promotes abnormalities of glomerular hemodynamics that are qualitatively similar to those elicited by acute NO inhibition, with the development of severe and progressive systemic and glomerular hypertension, besides intense renal vasoconstriction. After a few weeks of treatment, these rats exhibit glomerular ischemic and sclerotic injury, renal interstitial inflammation and vascular damage suggestive of malignant hypertension. Together, these findings indicate that NO exerts an indispensable tonic vasodilatation on renal and systemic microvessels, and that its absence may lead to severe cardiovascular and renal injury.
Evidence obtained in several studies suggests that changes in NO production can participate in the pathogenesis of deranged glomerular dynamics in CKD. Administration of inhibitors of NO synthase limits the hyperfiltration observed in experimental diabetes mellitus, suggesting that excessive renal production NO may be one of the mediators of the associated renal hemodynamic derangement. In subnephrectomized rats, 24-hour urinary excretion of nitrites and nitrates, taken as a surrogate marker of NO production, is significantly decreased. Similarly, in humans with chronic kidney disease (CKD), Schmidt and Baylis showed that urinary nitrites/nitrates excretion is diminished. These observations suggest that CKD is a state of NO deficiency. One of the possible causes of impaired NO synthesis in CKD is the abnormal production in the circulation of a potent endogenous competitive inhibitor of NOS, asymmetric dimethyl-L-arginine (ADMA). The presence of this compound was demonstrated in animal models of CKD, as well as in CKD patients. The finding of elevated ADMA levels at early phases of CKD suggests that subtle degrees of NO deficiency may be one of the primary factors causing glomerular hemodynamic dysfunction and leading to progression of renal injury. On the other hand, inhibition of NO aggravates kidney injury in subnephrectomized rats, in association with glomerular and peritubular endothelial cell loss, with consequent development of microthrombosis and glomerulosclerosis. Conversely, in partially nephrectomized rats, administration of the substrate for NO synthesis, L-arginine, prevented the decrease of GFR and renal blood flow, and reduced the severity of glomerulosclerosis. These observations suggest that endogenous NO still exerts a protective role even after the development of CKD, and point to a potential therapeutic use of NO-releasing drugs, although solid evidence in favor of this concept is lacking.
Prostanoids
Prostanoids are 20-carbon compounds derived from arachidonic acid through the action of two cyclooxygenase (COx) isoforms, COx-1, said to be “constitutive,” and COx-2, initially considered as being “inducible.” Subsequent research showed that both isoforms are active in normal subjects and should be considered “constitutive”. Although acute administration of vasodilator prostanoids such as prostacyclins and PGE2 can induce the synthesis of cyclic AMP and exert a pronounced effect on glomerular circulation and on sodium excretion, acute inhibition of prostaglandin synthesis in normal rats does not affect GFR or glomerular hemodynamics, indicating little tonic activity of these compounds. Likewise, the use of COx inhibitors as anti-inflammatories or painkillers usually promotes little or no change of renal function in most individuals, although adverse cardiovascular and renal effects such as sodium retention, hypertension and vascular injury may occur in a minority of patients treated chronically with these compounds.
Although the effects of COx inhibitors in normal subjects are relatively mild, the vasodilator prostanoids exert an important physiologic role by modulating the action of the renal sympathetic innervation and of circulating vasoconstrictors such as catecholamines and Ang II. For this reason, the use of COx inhibitors in situations in which there is exacerbation of vasoconstrictor activity, such as in states of volume depletion or contraction of the effective arterial volume, may promote exaggerated vasoconstriction and even the development of acute kidney injury.
The thromboxanes, the main representative of which is Thromboxane A2, are potent renal vasoconstrictors, which act through activation of phospholipase C and elevation of the intracellular calcium levels, antagonizing the effects of the vasodilator prostanoids. The thromboxanes also have an important function in hemostasis through platelet activation. Despite the intensity of their vascular effects, the thromboxanes, as in the case of vasodilator prostanoids, exert little effect on renal hemodynamics under physiologic conditions.
A number of observations suggest that vasodilator prostanoids may participate in the pathogenesis of CKD and early diabetic nephropathy by mediating at least part of the attending hemodynamic changes. The renal expression of COx-2 is increased in experimental diabetes mellitus, and may contribute to the renal vasodilatation observed in this condition. Likewise, renal mass ablation increases fractional urinary excretion of both vasodilator and vasoconstrictor prostaglandins in rodents. In partially nephrectomized rats, indomethacin lowers both SNGFR and renal blood flow. Together, these observations suggest that increased activity of cyclooxygenase derivatives may contribute to maintain the glomerular hemodynamic changes encountered in CKD models. However, a different picture emerges when the synthesis of prostanoids is blocked on a chronic basis in rats with renal ablation. Chronic treatment of subnephrectomized rats with either an unselective or a COx-2 selective cyclooxygenase inhibitor, starting on the day following renal mass removal, led to a mild fall in glomerular pressure, without change of GFR. Two months after renal ablation, renal injury was markedly attenuated in rats treated with the COx inhibitors. These observations suggest that prostanoids can act as mediators of progressive kidney injury through nonhemodynamic mechanisms, possibly related to inhibition of renal inflammatory mechanisms. These findings are difficult to translate into clinical practice, however, because, as just noted, increased prostanoid synthesis may be one of the mediators of single nephron hyperfiltration in CKD patients. Therefore, COx inhibition may abrogate this adaptive mechanism, leading to a sudden and dangerous fall of GFR in a substantial proportion of these subjects, a finding that is paralleled in experimental models of CKD. Thus, the use of COx inhibitors is better avoided in CKD.
Endothelin
Endothelin (ET), synthesized mostly, but not exclusively, by endothelial cells, is the most potent known vasoconstrictor. Experimental evidence indicates that ET constricts the afferent and efferent arterioles in proportion, without a clear predominance of one or another. As a result, Q A falls, while P GC remains approximately constant, although higher doses may raise glomerular pressure. Despite its vasoconstrictor potency, the physiologic role of ET in the regulation of the renal microcirculation is uncertain, because acute or chronic administration of ET inhibitors has little effect on glomerular microcirculation and renal function. Equally unclear is the participation of ET in CKD. In rats subjected to partial nephrectomy, Benigni and coworkers reported an increase in the urinary excretion of the best-known ET isoform, ET-1, and in the expression of the ET-1 gene. Moreover, thrombin stimulated ET-1 secretion in glomeruli isolated from remnant kidneys, an effect that was absent in glomeruli isolated from intact kidneys, raising the possibility that endothelins are involved in the alterations of glomerular hemodynamics seen after nephron loss. These investigators also showed that treatment with an ET inhibitor was renoprotective in the remnant kidney model, although a hemodynamic mechanism for this effect was not demonstrated. In streptozotocin diabetic rats, chronic ET receptor blockade caused glomerular vasodilatation and limited hyperfiltration, despite a simultaneous increase of renal plasma flow, suggesting that glomerular pressure was reduced, although direct measurement of this parameter was not performed. In the long run, diabetic nephropathy was largely prevented in treated rats.
Atrial Natriuretic Peptide
Atrial natriuretic peptide (ANP) is released mostly from the atrial wall, usually in response to extracellular volume expansion. As a result, the kidneys excrete a large amount of sodium, which tends to bring the hemodynamic status back to normal. This potent natriuretic effect stems from hemodynamic and nonhemodynamic renal actions. ANP promotes dilatation of the afferent arteriole and constriction of the efferent arteriole, a unique effect among vasoactive substances, with resulting elevation of P GC despite a concomitant fall of arterial pressure, which reflects systemic vasodilatation. Since Q A is also increased due to the predominance of the afferent dilatation, SNGFR increases, augmenting the amount of sodium that reaches the tubules. ANP may also inhibit renal sodium reabsorption by direct action and/or by promoting disproportionate medullary hyperperfusion thus amplifying the natriuretic effect of glomerular hyperfiltration.
The participation of ANP in the pathogenesis of the glomerular hemodynamic changes associated with CKD and diabetes is unclear. The plasma concentration of atrial natriuretic peptide (ANP) is elevated in partially nephrectomized rats on high-salt, but not on low-salt diets, suggesting a potential role of ANP in the adaptive increase of single-nephron sodium excretion in this model of chronic kidney injury. In experimental diabetes, the plasma levels of atrial natriuretic peptide are elevated, and may contribute to the attending renal hemodynamic abnormalities.
The Metabolic Derangement Itself May Promote Hyperfiltration in Diabetes Mellitus
Besides the possible role of prostaglandins and nitric oxide, discussed above, the glomerular hemodynamic abnormalities observed in early diabetes may be influenced by factors more directly related to the attending metabolic derangement. Acute glucose infusions have been reported to increase GFR in normal men, and in diabetic subjects, raising the possibility that hyperglycemia itself may promote hyperfiltration in diabetes. Other contributing factors may be the excessive production of some hormones that deeply influence carbohydrate metabolism, such as growth hormone and glucagon.
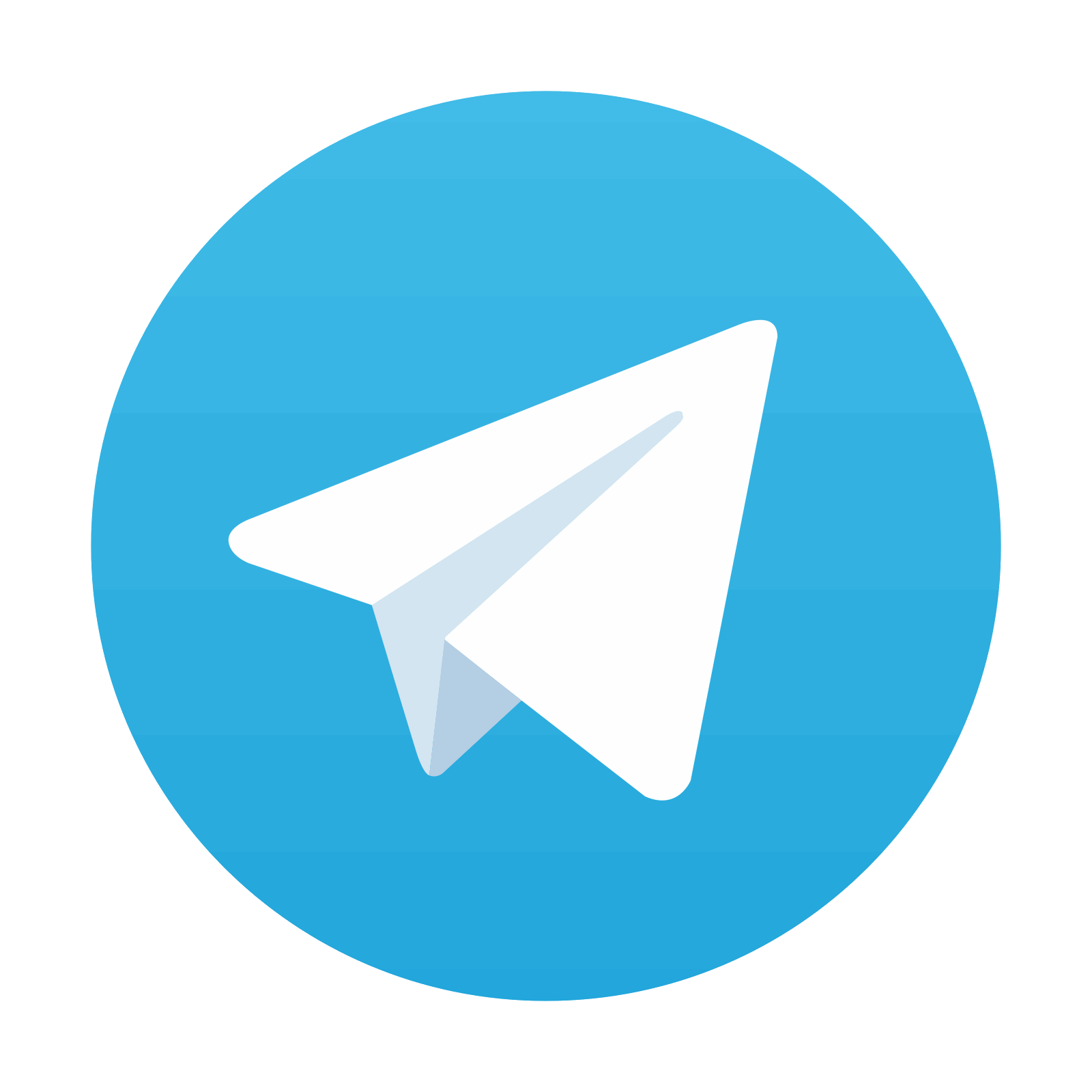
Stay updated, free articles. Join our Telegram channel

Full access? Get Clinical Tree
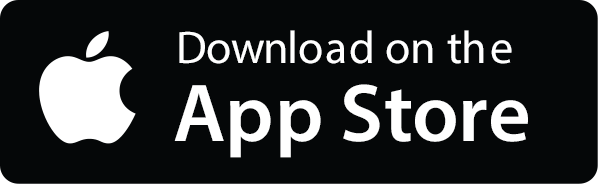
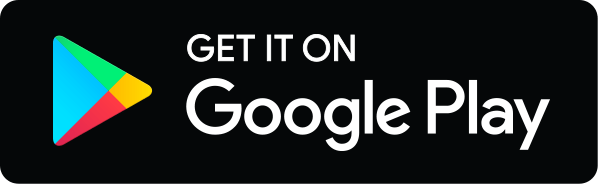