aging can result in endothelial dysfunction and changes in vasoactive mediators resulting in atherosclerosis, hypertension, and glomerulosclerosis.12 Furthermore, age-related changes in cardiovascular hemodynamics, such as reduced cardiac output and systemic hypertension, may contribute to glomerular changes.13 Moreover, dysautoregulation of the afferent and efferent arterioles may increase glomerular plasma flow, glomerular capillary pressure, and “hyperfiltration,” leading to mesangial matrix accumulation.14 A morphometric study showed dilatation of the afferent arterioles, increased glomerular capillary lumens (especially hilar), and enlarged glomeruli, which suggested a discordance between the afferent and efferent arterioles.15 The vascular adaptations to functional or structural nephron loss may help preserve glomerular filtration rate (GFR) by producing hyperperfusion and hyperfiltration in the surviving nephrons. This local glomerular hypertension and hypertrophy may lead to cytokine-mediated mesangial matrix expansion and, eventually, glomerulosclerosis. Such hyperperfusion-associated glomerular injury is seen with oligomeganephronia, diabetic nephropathy, morbid obesity, sickle cell anemia, and reflux nephropathy. It has been suggested that the vascular/ischemic changes seen in aging kidneys first cause cortical glomerulosclerosis and consequent juxtamedullary glomerular hypertrophy, followed by juxtamedullary glomerulosclerosis.16
TABLE 65.1 Morphological Changes of the Aging Kidney | ||||||||||||||||||||||||||||||||||||
---|---|---|---|---|---|---|---|---|---|---|---|---|---|---|---|---|---|---|---|---|---|---|---|---|---|---|---|---|---|---|---|---|---|---|---|---|
|
wall and narrowing of the arterial lumen produced by thickening of the medial smooth muscle layer, fibrosis of the media, and/or intimal thickening. These changes may be seen with hypertension, diabetes, and aging, with the prevalence of arterial sclerosis increasing with advancing age.7,19,20 Intimal fibroplasia or collagenous fibrosis of the arterial intima may be associated with thinning of the media and is found uniformly in older kidneys with or without underlying cardiovascular disease. Intimal fibroplasia is seen primarily in arteries that are 80 to 300 µm in diameter, such as the interlobular arteries. The regional heterogeneity of intimal hyperplasia may account for the heterogeneity of ischemic nephrons. Although the etiology of aging-associated intimal fibroplasia is not entirely clear, it starts early in life and is accelerated by hypertension. Intimal hyperplasia in the interlobular arteries may allow the transmission of the pulse wave into the smaller distal branches leading to arteriolar hyaline changes, which may in turn accelerate the proximal intimal fibrosis. Global glomerulosclerosis appears to be associated with arterial intimal fibrosis rather than with arteriolar hyaline change.21
![]() FIGURE 65.1 Morphological changes in the aging kidney. A: There are two glomeruli displaying solidified global glomerulosclerosis in which the sclerotic tufts fill the entirety of the Bowman space, often representing the sclerosis caused by focal segmental glomerulosclerosis. The nonsclerotic glomerulus shows ischemic changes with a segmental adhesion to the Bowman capsule (arrow). Significant tubular atrophy and interstitial fibrosis are also noted. A few arterioles demonstrate significant hyalinosis (double arrows, PAS, periodic acid-Schiff; × 200). B: The two glomeruli show ischemic obsolescence characterized by shrunken and globally wrinkled and thickened capillary tufts with the loss of most cells. The Bowman space is filled with collagenous material that stains less intensely than the capillary tuft (PAS; × 400). This type of global glomerulosclerosis is often secondary to ischemic vascular disease. C: An interlobular artery shows intimal fibrosis (arteriosclerosis) characterized by fibrous thickening and migration of medial muscle cells into the intima with an atrophic muscle layer (Trichrome; × 400). D: Classic type tubular atrophy: There is severe tubular atrophy with thickening and lamellation of the tubular basement membranes (PAS; × 400). E: A thyroidization type tubular atrophy: The atrophic tubules have a thin epithelium and contain homogeneous casts resembling thyroid tissue (H&E, hematoxylin and easin; × 400). F: An endocrine type tubular atrophy: The small tubules reveal cuboidal cells with pale-staining cytoplasma (containing abundant mitochondria) and virtually no lumens, reminiscent of endocrine glands (H&E; × 400). (See Color Plate.) |
unit kidney mass with advancing age, suggesting that the decrease in RBF does not simply reflect the decline in the renal mass with aging. In addition, they demonstrated that the fall in renal perfusion with aging is most profound in the cortex, with relative sparing of flow to the medulla. This redistribution of blood flow from the cortex to the medulla may explain the slight increase in filtration fraction observed in the elderly population. Studies on the morphology and histology of the renal vasculature by postmortem angiograms and histologic sections demonstrate increased irregularity and tortuosity of the preglomerular vessels and tapering of afferent arterioles. However, no characteristic histologic lesion of aging has yet been identified in the renal vasculature.
TABLE 65.2 Altered Glomerular, Tubular, and Vascular Functions in the Aging Kidney | ||||||||||||||||||||||||||||||
---|---|---|---|---|---|---|---|---|---|---|---|---|---|---|---|---|---|---|---|---|---|---|---|---|---|---|---|---|---|---|
|
(ACEI), angiotensin receptor blockers (ARB), calcium channel blockers, and nitrates, which relax smooth muscle in large and small conduit arteries throughout the body, thus resulting in arterial dilation.37,41
TABLE 65.3 Formulas to Estimate Glomerular Filtration Rate | ||||||||||||||||||
---|---|---|---|---|---|---|---|---|---|---|---|---|---|---|---|---|---|---|
|
wards in Italy, the relative risk of mortality in patients with eGFR = 30 to 59.9 or < 30 mL/min/1.73 m2 was compared to subjects with eGFR ≥ 60 mL/min/1.73 m2 using the body surface area-adjusted CG (CG-BSA), the MDRD, and the CKD-EPI formulas. Participants with reduced GFR showed an increased mortality regardless of the equation used, and CKD-EPI-derived GFR outperformed to some extent MDRD-and CG-BSA-derived GFR in a multivariable predictive model, suggesting its usefulness in the elderly population.50 However, the performance of the serum creatinine-based estimating equations still remains insufficiently evaluated in older patients, in whom there may be a high prevalence of chronic disease associated with alterations in muscle mass and diet, resulting in an overestimation of the measured GFR and an underestimation of the severity of CKD. Lately, cystatin C has emerged as an alternative marker for the measurement of kidney function.51 Cystatin C, a filtration marker that is less related to muscle mass than creatinine, may have a particular advantage in the estimation of GFR in the elderly population. However, the clinical role of cystatin C measurement remains unclear. In a recent study involving 11,909 patients, the risk of death, cardiovascular events, and kidney failure was compared in patients with GFR < 60 mL/min/1.73 m2 (CKD) to those with GFR > 60 mL/min/1.73 m2, as estimated by creatinine and cystatin C measurements. The survey showed that cystatin-based estimates were better predictors of adverse outcomes among adults with CKD, suggesting that cystatin C may be useful in identifying patients with CKD who have high risks of complications.52
situations, prompt treatment with free water restriction alone or with the concurrent administration of hypertonic solutions or vasopressin V2-receptor antagonists is warranted.60,61
believed that telomeres act as a mitotic clock, initiating replicative senescence when telomeres become critically short after a certain number of cell divisions. Melk et al.104 have demonstrated that telomere shortening progresses with advancing age in the human kidney, and this phenomenon is more important in the cortical than the medullary area.104
premature aging phenotype with soft tissue and vascular calcification, hyperphosphatemia, muscle and skin atrophy, and early death. In contrast, overexpression of the Klotho gene extended the life span in the mouse.110 In addition, several single-nucleotide polymorphisms in the human Klotho gene are associated with a shortened life span, osteoporosis, stroke, and coronary artery diseases, suggesting that Klotho may be involved in the regulation of human aging and age-related diseases.111
an elevation of the advanced glycosylation end products and their receptors (AGE and RAGE) that can cross-link adjacent proteins. This, along with the ROS that can activate ubiquitin-proteasome, may degrade hypoxia-inducible factor-1alpha (HIF-1α) and limit the capacity of the aging cells to form HIF-1-DNA hypoxia-responsive recognition element (HRE) complexes (HIF-1-HRE complexes).130,131 In the kidney, the subsequent decrease in the ability of the cells to respond to hypoxia could explain the attenuated anemiainduced secretion of erythropoietin as well as the decreased hypoxia-induced production of vascular endothelial growth factor leading, respectively, to reduced erythropoiesis and angiogenesis.130 In a rat model, renal aging was associated with a 60% decline in GFR, a threefold increase in renal F2 isoprostanes (a marker of oxidative stress), an increase in oxidant-sensitive heme oxygenase, as well as increased AGEs and RAGE. Furthermore, a diet rich in vitamin E attenuated the age-related upregulation of heme oxygenase and RAGE, suppressed the production of F2 isoprostanes, lessened measured markers of oxidative stress, reduced glomerulosclerosis, and improved renal plasma flow and GFR by 50%.132 In cultured rabbit proximal tubular epithelial cells, AGEs and even the early glycosylation end products (Amadori products) directly inhibited NOS activity and AGEs quenched the released NO.133 Immunohistochemical studies of the aging rat kidneys have revealed a reduction of endothelial NOS (eNOS) in the peritubular capillaries and the presence of eNOS immunoreactivity in renal tubular epithelial cells, infiltrating mononuclear cells and foci of tubulointerstitial injury, suggesting that the aging-related renal tubulointerstitial fibrosis may be secondary to the ischemia caused by peritubular capillary injury and impaired eNOS expression.134 Via activation of the angiotensin receptor AT1, the tissue RAS may promote the production of ROS and TGF-β1, events that can promote fibrosis. Indeed, the administration of ACEI and ARB in rats ameliorated the aging-related renal damage and attenuated glomerular sclerosis, mesangial expansion, tubular atrophy, interstitial fibrosis, and mononuclear cell infiltration.135 The salutary effect of RAS blockade may be, in part, mediated by the ability to limit the impact of aging on the structure and function of mitochondria and other cellular organelles involved in energy metabolism and ROS production.136
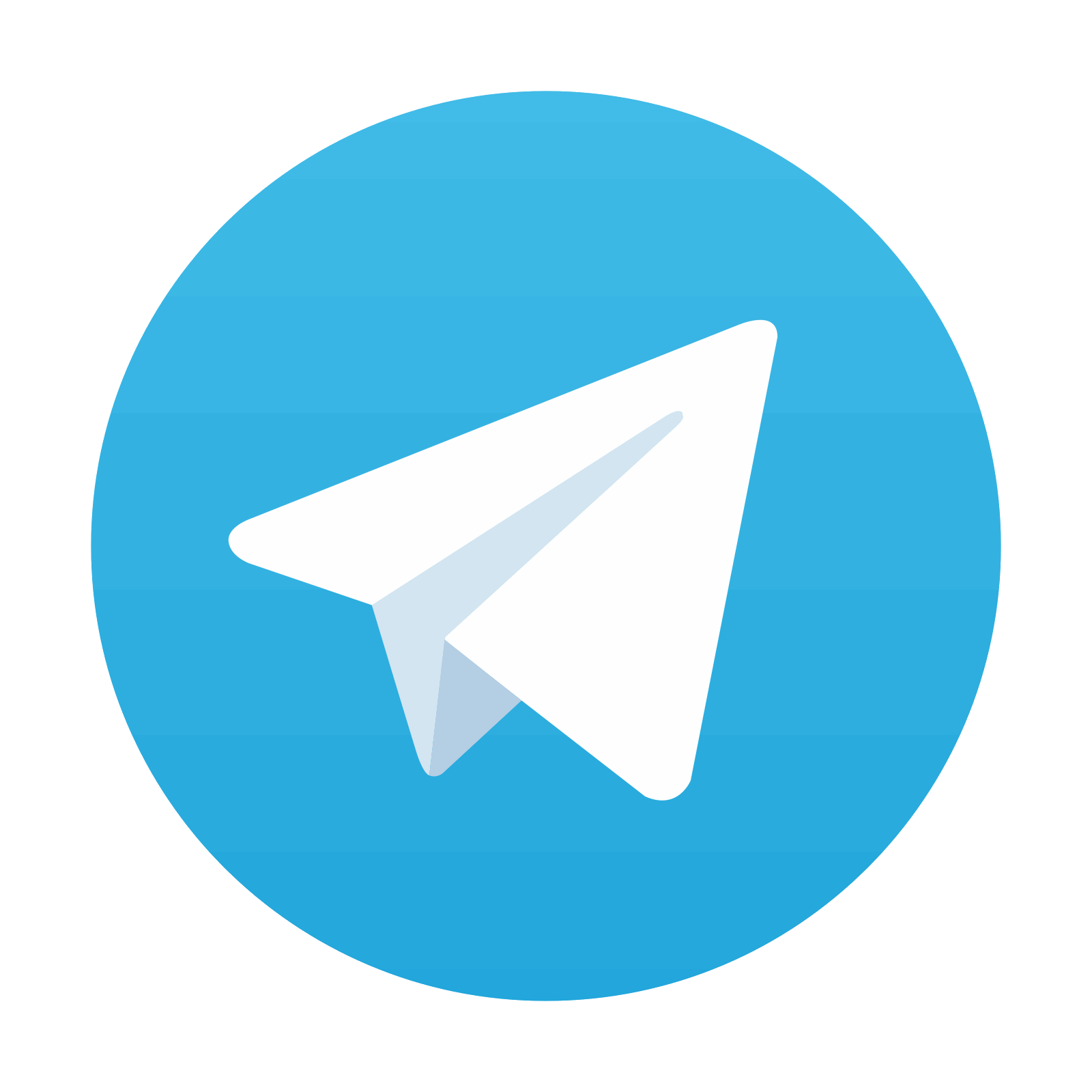
Stay updated, free articles. Join our Telegram channel

Full access? Get Clinical Tree
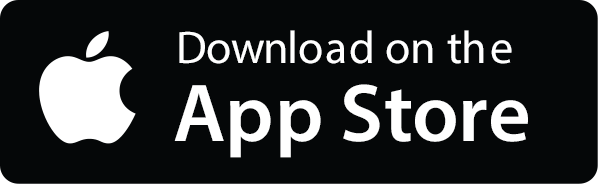
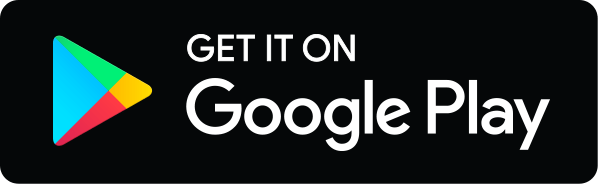