General Considerations
Peritoneal dialysis (PD) is an established form of renal replacement therapy that is used around the world. The concept of continuous ambulatory peritoneal dialysis (CAPD) was first described in 1976. During the 1980s there was rapid growth in the utilization of CAPD in the United States with the development of chronic indwelling PD catheters and the introduction of PD solution in sterile, disposable plastic bags. In the 1990s there was a rapid increase in the number of patients on automated peritoneal dialysis (APD) with the increased interest in dialysis adequacy and the development of simplified, automated cycler machines. In more recent years, however, the growth of PD has decreased in the United States. United States Renal Data System (USRDS) data from 1998 to 2002 indicate that the prevalent PD population decreased by 3.5% per year, with only 8% of prevalent dialysis patients being treated with PD in 2002. In contrast to the experience in the United States, the prevalent number of patients with end-stage renal disease receiving PD has exceeded 60% in other countries, such as in Mexico and Hong Kong. The cause for these differences is likely multifactorial and is related to access to PD, physician expertise, patient mix, and reimbursement.
The selection of dialysis modality is influenced by a number of considerations such as availability and convenience, medical factors, and socioeconomic and dialysis center factors. In general, the one absolute contraindication to chronic PD is an unsuitable peritoneum due to the presence of extensive adhesions, fibrosis, or malignancy. Other relative contraindications do exist (Table 51–1). PD continues to be the preferred dialysis modality for infants and young children, patients with severe hemodynamic instability on hemodialysis, and patients with difficult vascular access. Studies investigating differences in patient mortality between PD and hemodialysis (HD) have been conflicting. Most reports have shown no significant difference in survival between PD and nondiabetic HD patients. Survival by dialysis modality among diabetic patients may vary with age. Some series have found lower survival for older diabetic patients on PD compared to HD. Variable results in these mortality studies have been affected by differences in patient comorbidities, inclusion of prevalent versus incident patients, and the types of analytical methods utilized. Real differences in mortality between PD and HD can be assessed only in prospective, randomly controlled trials that will be very difficult and therefore unlikely to be performed. Technique survival is shorter in PD compared to HD due to peritonitis, peritoneal membrane failure, and patient burnout.
Absolute contraindication |
Extensive abdominal adhesions or documented loss of peritoneal function |
Relative contraindications |
Abdominal hernias |
Presence of colostomy, ileostomy, nephrostomy, or ileal conduit |
Recurrent chronic backache with preexisting disc disease |
Severe psychological and social problems |
Severe diverticular disease of the colon |
Severe neurologic disease, movement disorder, or severe arthritis preventing self care (caregivers can be trained to perform the peritoneal dialysis) |
Severe chronic obstructive pulmonary disease |
Malnutrition |
Clinical Findings
PD involves the transport of solutes and water across the peritoneal membrane, which separates the blood in the peritoneal capillaries and the dialysis solution in the peritoneal space. The dialysis solution in the peritoneal space typically contains sodium, chloride, calcium, and lactate and is made hyperosmolar by the addition of various amounts of glucose. The peritoneal membrane as a dialyzer has a surface area that typically ranges from 1 m2 to 2 m2 in adults. It is a complex membrane composed of the capillary endothelium and associated basement membrane, the intersitium, the mesothelium, and a stagnant fluid film that overlies the peritoneal membrane. The transport of water and solutes across this membrane best fits a model that describes the presence of three different sized pores in the peritoneal membrane. Large pores that are few in number and thought to represent clefts between endothelial cells allow the transport of macromolecules such as proteins. Numerous small pores are responsible for the transport of small solutes such as urea, creatinine, and cations and anions, while ultrasmall, transcellular pores or aquaporins allow the movement of water only.
In PD, solutes are transported by the processes of diffusion and ultrafiltration. During the course of a PD dwell, smaller solutes such as creatinine, urea, and potassium diffuse down a concentration gradient from the peritoneal capillary blood into the peritoneal dialysis solution. With increasing dwell time, the ratio of dialysate to serum levels approaches 1 (Figure 51–1). Thus, for small solutes such as urea, the removal is maximal at the start of the dwell, when the concentration in the dialysis solution is zero, and gradually decreases during the course of the dwell. Diffusion becomes more restricted as molecular weight increases (ie, urea diffuses faster than creatinine). Glucose, lactate, and calcium will diffuse in the opposite direction, from dialysate into the blood.
Ultrafiltration occurs as a result of an osmotic pressure gradient between the dialysis solution and the peritoneal capillary blood. The dialysis solution is made hypertonic usually by the addition of high concentrations of glucose. Ultrafiltration is maximal at the beginning of a dwell when the osmotic pressure gradient is highest. The osmotic pressure gradient will decrease over time due to the dilution of the glucose by ultrafiltrate and by the diffusion of glucose from the peritoneal cavity into the bloodstream (Figure 51–2). Fluid removal is maximized by using more hypertonic dialysis solutions or by doing more frequent exchanges. During ultrafiltration solutes present in body fluids will be swept along by solvent drag, contributing to overall solute clearance. Water and solutes are also constantly being absorbed from the peritoneal cavity into the lymphatic system and this will counteract both solute and fluid removal.
Not all patients’ peritoneal membranes transport solute at the same rate. In clinical practice, a patient’s peritoneal membrane transport characteristics can be determined by measuring the creatinine equilibration curve and the glucose absorption curve during a standardized peritoneal equilibration test (PET). Conventionally, the PET involves a 2-L, 2.5% dextrose dwell with dialysate samples taken at 1, 2, and 4 hours and a plasma sample at 2 hours. Net fluid removal is also measured along with the ratio of dialysate glucose at 4 hours to dialysate glucose at time zero. Patients are classified principally into one of four transport categories: high, high-average, low-average, and low (Figure 51–3).
High transporters will have the fastest equilibration of creatinine, but ultrafiltration will not be as great due to rapid absorption of glucose and dissipation of the osmotic gradient. High transporters also tend to have higher dialysate protein losses. In contrast, low transporters will have less complete equilibration of creatinine, but will have good net ultrafiltration due to the slower absorption of glucose into the bloodstream. Thus, high transporters tend to do better on regimens that have frequent, short duration dwells, such as APD, whereas low transporters tend to do better on regimens with longer duration dwells, such as CAPD. Average transporters are generally able to do well on a variety of PD regimens.
Complications
Peritonitis remains a leading complication of PD. It contributes to technique failure, hospitalization, and patient mortality. Peritonitis is thought to occur most often by touch contamination, but may also occur in the setting of a catheter exit site or tunnel infection, by transmural migration of bacteria through the intestinal wall, or rarely by the hematogenous or transvaginal route. Patients with peritonitis usually present with cloudy peritoneal fluid and abdominal pain. When a patient presents with these complaints the abdomen should be drained and the effluent sent for cell count with differential, Gram stain, and culture. At least two of the following three conditions should be present to make the diagnosis of peritonitis: symptoms and signs of peritoneal inflammation, an effluent white blood cell count of more than 100/mm3 with at least 50% polymorphonuclear neutrophil cells, and a positive culture from the dialysate. Using appropriate culture techniques an organism can be isolated from the peritoneal fluid in over 80% of cases. Infections due to gram-positive cocci (Staphylococcus epidermidis and Staphylococcus aureus) tend to be most common (60–70% episodes) compared to infections with gram-negative bacteria (15–25%) or fungi (2–3%). Infection with mycobacteria can also occur but is rare. Peritonitis with multiple organisms or anaerobes should raise the concern of intrabdominal pathology and lead to abdominal computed tomography (CT) scan and surgical evaluation.
Patients who present with signs and symptoms of peritonitis should always be treated empirically with antibiotics. Various antibiotics can be used to treat peritonitis, but empiric therapy must cover both gram-positive and gram-negative organisms. Guidelines outlining empiric antibiotic regimens are published and have changed over the years in response to concern over the development of vancomycin-resistant organisms and appreciation of the importance of preservation of residual renal function. Gram-positive organisms may be covered by vancomycin or a cephalosporin, and gram-negative organisms by a third-generation cephalosporin or aminoglycoside. Numerous combinations are possible, but the choice of antibiotics should take into account the patient’s infection history and the center’s history of resistant organisms. Antibiotics are usually administered by the intraperitoneal route and can be given by intermittent (once daily) or continuous (in each exchange) dosing (Table 51–2). Most patients will show considerable clinical improvement within 48 hours of starting antibiotic therapy. Final antibiotic therapy should be guided by culture results and sensitivities. Treatment should be continued for a total of 2 weeks, while more severe infections due to S aureus, pseudomonas, or multiple gram-negative organisms should be treated for 3 weeks. If there is no clinical improvement after 48 hours, cell counts and cultures should be repeated. Refractory peritonitis is defined as failure to respond to appropriate antibiotics within 5 days and should be managed by catheter removal. Other indications for catheter removal are fungal peritonitis, relapsing peritonitis, peritonitis in the setting of severe exit site or tunnel infection, and infection due to multiple enteric organisms in the setting of a surgical abdomen. During peritonitis the permeability of the peritoneal membrane increases due to inflammation, and patients often will need a more concentrated dialysis solution in order to maintain fluid removal. Protein losses will also increase during peritonitis. Peritonitis is also often associated with increased fibrin clot production that can occlude the dialysis catheter. Heparin can be added to each bag of dialysis solution (500–1000 units/L) to decrease fibrin clot production.
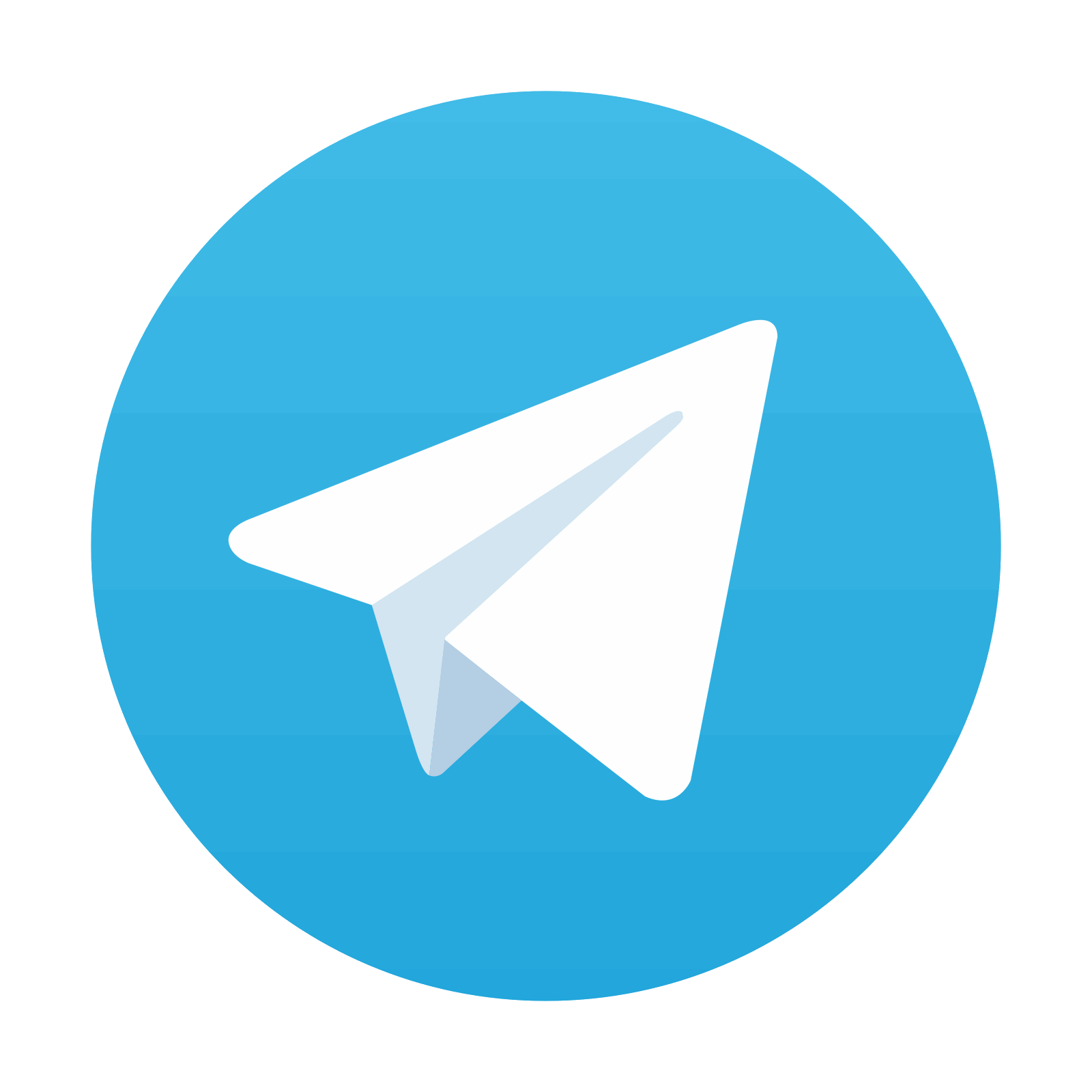
Stay updated, free articles. Join our Telegram channel

Full access? Get Clinical Tree
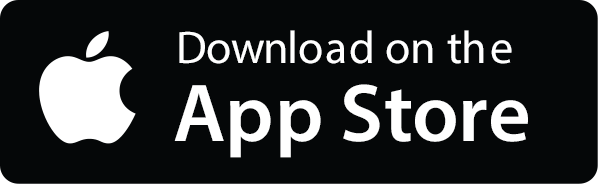
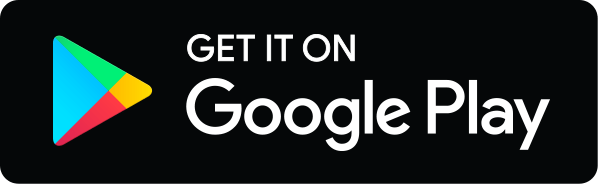