Renal proximal tubules efficiently secrete anionic and cationic drugs and toxins. Many of the involved transporters are members of the solute carrier family 22 ( SLC22 ) and exhibit a broad substrate specificity. Uptake of organic anions from blood into proximal tubule cells occurs through organic anion transporters 1 and 3 (OAT1/ SLC22A6 and OAT3/ SLC22A8 ), and the uptake of organic cations involves organic cation transporters 2 and 3 (OCT2/ SLC22A2 and OCT3/ SLC22A3 ). In humans, the release of organic anions from cells into the tubule lumen can occur by anion exchange (OAT4/ SLC22A9 ) and can be voltage- (NPT4/ SLC17A3 ) or ATP-driven (MRP2/ ABCC2 and MRP4/ ABCC4 ). Organic cations are released by exchange against protons through the multidrug and toxin extrusion 1 and 2-K (MATE1/ SLC47A1 and MATE2-K/ SLC47A2 ) and OCTN1/ SLC22A4 , by exchange against organic cations by OCT1 and OCTN2/ SLC22A5 , and by the ATP-driven P-glycoprotein (MDR1/ ABCB1 ). We summarize the molecular properties of the organic anion and cation transporters, their short and long term regulation and altered function as a consequence of single nucleotide polymorphisms, and their interaction with drugs.
Keywords
proximal tubule, secretion, organic anion transporters, organic cation transporters, gender differences, molecular modeling, drug transport, drug-drug interaction, single nucleotide polymorphisms
Introduction
The kidneys efficiently excrete endogenous and exogenous organic anions and cations, including widely used drugs and potentially toxic compounds. At pH 7.4, organic anions carry one or more negative charges, and organic cations one or more positive charges, respectively. Many exogenous organic anions and cations undergo secretion in renal proximal tubules, involving uptake across the basolateral membrane into tubule cells and release across the apical (lumenal, brush-border) membrane into the lumen. For organic anions, uptake from the blood takes place against an opposing inside negative membrane potential whereas, for organic cations, the inside negative electrical potential difference has to be overcome during their release into the lumen. Thus, secretion requires the input of cellular energy. In this chapter, we shall focus on proximal tubular organic anion and organic cation transporters being involved in drug secretion.
Organic Anion Transport Systems
Characteristics of Organic Anion Secretion in Proximal Tubules
Initial experiments revealed proximal tubules as the principle site of organic anion secretion in various species (for earlier literature see ). In most of these studies, p -aminohippurate (PAH) served as a model organic anion, and the substrate specificity of the so-called PAH transport system in the basolateral membrane was extensively tested by stop-flow competition experiments with rat kidneys in situ . This system preferred amphiphilic compounds carrying one negative charge or two negative charges separated 6–7 Å by a hydrophobic moiety and being able to form multiple hydrogen bridges. The “PAH transport system” turned out to interact with a multitude of chemically unrelated molecules including many drugs.
PAH uptake across the basolateral membrane occurred by an exchange against intracellular α-ketoglutarate. Thereby, PAH uptake is a tertiary active process, with the Na + ,K + -ATPase establishing the extracellular-to-intracellular Na + gradient (primary active process), the sodium-dicarboxylate cotransporter utilizing this Na + gradient for intracellular α-ketoglutarate enrichment (secondary active process), and the PAH/α-ketoglutarate exchanger using the intracellular-to-extracellular α-ketoglutarate gradient for PAH uptake against the inside negative membrane potential (tertiary active process; see also Figure 72.1 ). In addition, α-ketoglutarate absorbed from the primary urine or derived from metabolism can drive PAH uptake. The arrangement of three co-operating transporters is conserved among species including man. As opposed, earlier experiments on PAH release across the apical membrane revealed species differences.

With the advent of molecular cloning and heterologous expression techniques, several proximal tubular transporters for organic anions have been characterized. Figure 72.1A shows transporters that are involved in the secretion of anionic drugs and toxins, or are targets of drugs, in the human proximal tubule. These include OAT1, OAT2, OAT3, OATP4C1, and MRP6 in the basolateral cell membrane, and OAT4, OAT10, URAT1, NPT4, MRP2, and MRP4 in the apical membrane. In rodent proximal tubules, Oat1, Oat3, Oatp4c1, and Mrp3 were found in the basolateral membrane, and Oat2, Oat5, Urat1, Oat-K1/2, Oatp1a1, Npt1/Oat v 1, Mrp2, and Mrp4 in the apical membrane ( Figure 72.1B ). Thereby, both cell sides contain antiporters (OATs/Oats, URAT1/Urat1, OATP4C1/Oatp4c1), ATP-driven systems (MRPs/Mrps) and a voltage-driven system (NPTs/Npts). Taken together, the picture of organic anion transport became more complicated than it was anticipated from earlier in vivo and in vitro functional studies.
Drug Transporters in the Basolateral Membrane of Proximal Tubule Cells
The Organic Anion Transporter 1 (OAT1/Oat1; SLC22A6/Slc22a6 )
The Organic Anion Transporter 1 (humans, OAT1; other species, Oat1) was cloned from man, monkey, pig (p), rabbit (rb), rat (r), and mouse (m). OAT1/Oat1 is the member A6/a6 of family SLC22/Slc22 , that consist of at least 25 different genes. The gene coding for OAT1 was located to chromosome 11q12.3 paired with that of OAT3. OAT1 mRNA is expressed in kidneys, and to a small extent also in brain and other tissues. In rats, mRNA expression rose from day 10 after birth and reached a maximum in adult animals whereas, in mice, Oat1 mRNA did not rise before day 25 after birth. Importantly, gender differences were observed in adult rats and mice with higher Oat1 mRNA expression in male animals.
Immunohistochemical studies localized OAT1/Oat1 protein to the basolateral membrane of proximal tubules of human, monkey, rat and mouse kidneys. In humans, OAT1 was found along the complete proximal tubule whereas rOat1 was highest in the S2 segment. Immunohistochemistry in adult rats revealed more Oat1 protein in male than in female animals. Whether gender differences are present in humans is not known (for reviews on gender differences see ).
The expression of human OAT1 was under positive control of hepatocyte nuclear factors 1α (HNF-1α) and 4α (HNF-4α). An increased expression of rOat1 was found after treatment of kidney slices or proximal tubules with insulin and epidermal growth factor as well as after bile duct ligation in rats whereas, in several other circumstances such as fever, endotoxinemia, renal insufficiency, ischemia-reperfusion, and ureter obstruction, the abundance of rOat1 was—at least transiently—decreased (reviewed in ). A down-regulation of Oat1 expression most probably decreases the renal excretion of anionic drugs. In this regard, it is interesting that inhibition of COX-2 attenuated the endotoxin-induced down-regulation of rOat1.
Short-term down-regulation involves a protein kinase C (PKC) activity-dependent endocytosis of OAT protein. Thereby, the protein itself is not phosphorylated, but possibly ancillary proteins such as caveolin 2. The appearance of immunoreactive rOat1 in vesicles beneath the basolateral membrane after mercury treatment of rats suggests that endocytosis of Oat1 may occur also in vivo .
Several non-synonymous single nucleotide polymorphisms have been described for human OAT1 (summarized in ). Except for the amino acid change R50H which led to changes in the K m for adefovir, cidofovir and tenofovir, other amino acid exchanges did not result in altered transport function, at least for the tested substrates.
Following heterologous expression, OAT1/Oat1 proved to be a p -aminohippurate (PAH)/α-ketoglutarate antiporter with in vitro affinities for PAH between 5 and 50 µM (range, 3.1 to 430 µM ). A prototypical, though not OAT1-specific inhibitor is probenecid with K i or IC 50 values between 1.4 and 18.6 µM. Numerous compounds were tested as possible substrates of OAT1/Oat1 either by checking their inhibition of the uptake of labeled PAH (or other labeled or fluorescent substrates), or by direct demonstration of transport of labeled test compounds (results compiled in ). Among endogenous substrates of OAT1/Oat1 are, e.g., medium-chain fatty acids, α-ketoglutarate, cAMP and cGMP, prostaglandins E 2 and F 2α , urate, and acidic neurotransmitter metabolites. Drugs interacting with OAT1/Oat1 included β-lactam antibiotics, antiviral drugs, non-steroidal anti-inflammatory drugs, diuretics, ACE inhibitors, angiontensin II receptor 1-antagonists, and methotrexate. Furthermore, OAT1/Oat1 interacted with uremic toxins, environmental toxins, and carcinogens. Thus, OAT1/Oat1 covers a wide substrate spectrum, is involved in renal drug handling, and contributes to nephrotoxicity. The interaction of several compounds with the same transporter, OAT1/Oat1, can lead to drug-drug interaction during renal excretion, alter pharmacokinetics and cause potentially serious side effects (for examples see ).
For mouse Oat1, a quantitative structure-activity relationship was determined. For mono-anions, interaction with mOat1 increased (or K i decreased) with increasing mass and hydrophobicity, e.g., with the chain length of fatty acids. For di-anions, steric and electrostatic factors became the main determinants of affinity. For transport velocity, other factors seemed important because no correlation was found between V max and K m or K i . In general, QSAR on mOat1 was in agreement with earlier data obtained for PAH transport in rat kidney in situ . Mutational studies on OAT1/Oat1 were performed to find out residues important for transport function: amino acid residues surrounding the putative substrate binding site of human OAT1 include R466 (transmembrane helix TMH11), K382 (TMH8), Y353, Y354 (both in TMH7), and F374 (TMH8) (summarized in ). R466 is also important for the interaction of OAT1 with chloride that increases the V max of the transporter, but has no effect on its affinity towards PAH.
An Oat1 knockout mouse was generated which did not show any gross abnormalities. The clearance of PAH, furosemide, bendroflumethiazide, and a number of endogenous hydroxyl-substituted short chain fatty acids was decreased. Thus, mOat1 contributes to the renal excretion of diuretics and of hitherto unknown endogenous metabolites.
The Organic Anion Transporter 2 (OAT2/Oat2; SLC22A7/Slc22a7 )
OAT2/Oat2 was cloned from man, rat, and mouse. The gene for human OAT2 is located on chromosome 6p21.1. In humans and male rats, the expression of OAT2/Oat2 mRNA was high in liver and low in kidneys. In female rats, Oat2 expression in kidneys exceeded its expression in liver. In male mice, message for Oat2 was expressed in kidneys, but hardly in liver whereas, in female mice, message was found both in liver and kidneys. Immunolocalization studies revealed human OAT2 at the basolateral membrane of proximal tubule cells. In rat and mouse kidneys, Oat2 appeared to be present in the apical membrane of proximal tubule cells and exhibited a higher expression in female animals. Thus there are gender and species differences in Oat2 expression.
In liver and kidneys, OAT2/Oat2 expression was under positive control of HNF-1α and HNF-4α. In diabetic rats and in cisplatin-treated mice, a decreased renal Oat2 expression was found. As regards single nucleotide polymorphisms, three non-synonymous SNPs were reported the functional consequence of which is unknown (see ).
Due to conflicting results in the literature, it is unclear whether OAT2/Oat2 interacts with and is driven by dicarboxylates. High affinities of human and rat OAT2/Oat2 were reported for prostaglandin E 2 and prostaglandin F 2α . Other endogenous substrates of OAT2 are nucleobases, nucleosides and nucleotides such as adenine, adenosine, GMP, GDP, GTP, cGMP, and cAMP. Urate was transported by OAT2 with an apparent K m of 1.17 mM, suggesting that OAT2 contributes to proximal tubular urate secretion. Among drugs, some diuretics (hydrochlorothiazide, furosemide), pravastatin, sartanes (losartan and telmisartan), cephalosporins, erythromycin, histamine receptor-2 blockers, NSAIDs, and uricosurics (probenecid, benzbromarone) interacted with OAT2. High affinities were reported for antineoplastic drugs (methotrexate, fluorouracil, taxol).
In humans, the basolateral localization of OAT2 would favour a role in organic anion (urate) and drug excretion whereas, in rodents, the apical location of Oat2 is hard to reconcile with secretion. Thus, more information is needed to fully appreciate the function of OAT2/Oat2.
The Organic Anion Transporter 3 (OAT3/Oat3; SLC22A8/Slc22a8 )
As reviewed in, OAT3/Oat3 was cloned from man, monkey, pig, rabbit, rat, and mouse. The gene SLC22A8 is located on chromosome 11q12.3, in direct neighbourhood to the gene of OAT1. In all species, the mRNA for OAT3/Oat3 was highest in the kidneys; additional expression was found in brain, liver, skeletal muscle, and adrenals. The mRNA levels increased shortly after birth and reached the same levels in mature male and female rats. Based on quantitative mRNA analysis, the expression of OAT3 was threefold higher than that of OAT1, and more than tenfold higher than the expression levels of OAT2 and OAT4. The mRNA levels corresponded to protein expression as tested by Western blotting, indicating that OAT3 is the most abundant OAT isoform in the human kidney.
In immunohistochemistry, OAT3/Oat3 was found at the basolateral membrane of proximal tubules as well as in thick ascending limbs of Henle’s loops, connecting tubules and collecting ducts; gender differences were only apparent for rOat3 in proximal tubules with lower expression in female rats (reviewed in ). OAT3/Oat3 was also found in human, rat, and mouse choroid plexus. At this location, OAT3/Oat3 is likely involved in the uptake of organic anions including neurotransmitter metabolites and drugs from the cerebrospinal fluid and their transport into the blood. Indeed, Oat3 knockout mice showed an impaired cerebrospinal fluid-to-blood transport of fluorescent organic anions.
Hepatocyte nuclear factors HNF-1α and HNF-1β induced OAT3 expression and HNF-1α knockouts showed a diminished renal expression of Oat3 (reviewed in ). Inhibition of promoter methylation also increased OAT3 expression. As summarized in, OAT3/Oat3 expression is subject to regulation by various factors. Insulin, epidermal growth factor and short term exposure to prostaglandin E 2 increased whereas long term PGE 2 elevation as observed in fever and inflammatory states decreased rOat3. Intracellular cAMP served to increase the OAT3 promoter activity. Several kidney disorders such as ischemia-reperfusion, ureteral obstruction as well as methotrexate and cisplatin treatment decreased rOat3 abundance. Biliary obstruction and 5/6 nephrectomy increased rOat3 protein expression (see ).
The existence of several single nucleotide polymorphisms in the promoter and the coding region of human OAT3 have been described (compiled in ), including 9 non-synonymous mutations, leading to changes in the amino acid sequence or premature truncation. Three loss-of-function mutations (R149S, Q239X, I260R) were found in Asian-Americans. The impact of these mutations is unknown.
A widely used test substrate for heterologously expressed OAT3/Oat3 is estrone-3-sulfate with half-maximal transport rates ( K t or K m ) between 2.2 and 21.2 µM. PAH was translocated by OAT3/Oat3, but the affinity was smaller than that of OAT1/Oat1. OAT3/Oat3 is driven by the outwardly directed concentration gradient of α-ketoglutarate. Thus, OAT3/Oat3 is involved in uptake of organic anions from the blood, which fits to its location at the basolateral membrane.
Numerous compounds have been tested as putative substrates of OAT3/Oat3 (results compiled in ). An interaction was demonstrated for endogenous substances such as the second messengers cAMP, cGMP; the vitamin folate; the bile salts cholate and taurocholate; the (sulfated) hormones cortisol, dehydroepiandrosterone sulfate, estrone sulfate; the local hormones prostaglandin E 2 and F 2α ; the purine metabolite urate; and some neurotransmitter metabolites. Highly interesting is the observation that Oat3 knockout mice have a decreased blood pressure. Obviously, intact mOat3 excretes one or more endogenous substances that lower blood pressure. Thymidine could play such a role, because knockout mice had an increased thymidine concentration in blood, and thymidine infusion lowered blood pressure.
Drugs interacting with OAT3/Oat3 comprised ACE inhibitors, angiotensin II receptor blockers, diuretics, statins, antibiotics, antineoplastics, immune suppressants, histamine receptor 2 blockers, and non-steroidal anti-inflammatory drugs. As compared to OAT1, OAT3/Oat3 has higher affinities for urate, benzylpenicillin, and loop diuretics, suggesting a dominant role in proximal tubular secretion of these compounds. Indeed, Oat3 knockout mice showed a decreased renal excretion of diuretics, benzylpenicillin, quinolones and methotrexate.
Chimeras between rat Oat3 and Oat1 revealed that substrate recognition is located in transmembrane helices TMH 6-12, i.e. in the C-terminal part of rOat3. Replacement of the positively charged arginine 454 that is conserved in all OATs, by the acidic amino acid aspartate (R454D) abolished PAH uptake, but did not change the handling of cimetidine. The mutant lysine-370 to alanine (K370A) also was unable to translocate PAH, but still transported cimetidine, indicating that cimetidine interacts with amino acid residues different from those involved in binding and translocation of PAH, and that translocation of PAH requires the presence of cationic amino acid residues in TMH 8 and 11. Aromatic residues in TMH 7 (W334, F335, Y341) and F362 in TMH 8 were found to be important for the translocation of PAH and cimetidine, but not for the transport of estrone sulfate. Whether rOat3 has, as suggested, three parallel transportation paths, one for PAH, one for cimetidine, and one for estrone sulfate, must be clarified in future experiments.
In summary, OAT3/Oat3 has a wider distribution than OAT1/Oat1 along the nephron, operates as an organic anion/α-ketoglutarate exchanger, and handles small and, unlike OAT1/Oat1, also larger and more hydrophobic substrates.
The Organic Anion Transporting Polypeptide 4C1 (OATP4C1/Oatp4c1; SLCO4C1/Slco4c1 )
OATP4C1 was cloned from human and rat kidneys and in both species, OATP4C1/Oatp4c1 proteins were found in the basolateral membrane of proximal tubule cells. The gene for human OATP4C1 was located on chromosome 5q21.2. In humans, the expression was found in kidneys, liver, and weakly in lungs. In rats, predominant expression was found in the kidneys and a weaker one in the lungs. Gender differences were not apparent. Thus, OATP4C1 can be regarded as a kidney-specific OATP. Functional tests revealed the transport of the endogenous compounds cAMP, triiodothyronine, thyroxine, but not of cGMP, taurocholate, prostaglandin E 2 , estradiol-17β-D-glucuronide, and PAH. Among drugs, digoxin, ouabain, and methotrexate were taken up by OATP4C1. It was assumed that the physiological role of OATP4C1/Oatp4c1 is to present thyroid hormones to proximal tubule cells. The extent to which this transporter contributes to the proximal tubular secretion of drugs remains to be determined.
The Multidrug Resistance-Associated Protein 6 (MRP6/Mrp6; ABCC6/Abcc6)
MRP6/Mrp6 is a member of the ATP binding cassette (ABC) proteins that is mainly expressed in liver and kidneys. The murine homologue, mMrp6, showed highest expression in the liver, but was also found in other organs including the skin. Within human and mouse kidneys, MRP6/Mrp6 was localized to the basolateral membrane of proximal tubule cells (see ). This ATP-driven transporter handled glutathione-conjugates, the endothelin receptor antagonist BQ-123, and conferred low level resistance to epipodophyllotoxins and anthracyclines; probenecid and indomethacin inhibited MRP6. Mutations in the second nucleotide binding domain were associated with the connective tissue disease, Pseudoxanthoma elasticum , but the exact causal relationship between MRP6 and this disorder is unknown. Given the localization in the basolateral membrane of proximal tubule cells and the function of an ATP-driven efflux transporter it is unlikely that MRP6 contributes to organic anion secretion.
MRP1 (ABCC1) was found in the basolateral membrane of Henle’s loops and collecting ducts, and MRP3 (ABCC3) in distal tubules. In rats, Mrp3 was localized also to the basolateral membrane of proximal tubules. Also these transporters are not likely involved in organic anion secretion but may protect renal tubule cells from cytotoxic compounds.
Drug Transporters in the Apical Membrane of Proximal Tubule Cells
The Organic Anion Transporter 4 (OAT4, SLC22A11 )
This transporter (reviewed in ) occurs only in humans. The gene for OAT4 is located on chromosome 11q13, in close neighbourhood to that of URAT1. OAT4 expression was highest in the kidneys, lower in placenta, and virtually absent from all other tested organs. Immunohistochemical studies revealed the presence of OAT4 in the proximal tubules only where it is located at the apical membrane. Thereby, OAT4 interacts with the scaffolding proteins PDZK1 and NHERF1 through its three C-terminal amino acids. Factors influencing the expression of OAT4 are presently unknown.
Mutational analysis revealed that evolutionary conserved glycine residues at positions 241 and 400 (transmembrane helices 5 and 8, respectively) are functionally important. Replacement of these residues by long aliphatic amino acids abolished transport activity, whereas the glycine-to-alanine mutation (G241A, G400A) reduced transport rates and decreased affinities were observed. Glycine is thought to participate in helix-helix interactions that seem to be important for transport activity of OAT4.
Eight non-synonymous single nucleotide polymorphisms were found, one of which (R48X) is deleterious (reviewed in ). The impact of the other amino acid changes on the function of OAT4 is unknown.
Following heterologous expression (for results see ), OAT4 transported estrone-3-sulfate with high affinity ( K t of 1 µM). Other endogenous compounds translocated by OAT4 were urate, the prostaglandins E 2 and F 2α , and dehydroepiandrosterone sulfate; no inhibition was found with glucuronidated steroids, suggesting that OAT4 only handles sulfated compounds. Cholate, taurocholate, octanoate, and corticosterone inhibited OAT4. Intracellular glutarate trans -stimulated estrone-3-sulfate uptake, but did not cis- inhibit it when present in the extracellular medium, suggesting different affinities for this dicarboxylate at the inner and outer binding sites. Similar findings for PAH indicate that the internal binding site has a higher affinity to this organic anion than the external binding site. Labeled PAH was not taken up by OAT4, but its efflux was accelerated by estrone-3-sulfate in the medium. It was concluded that OAT may work asymmetrically, taking up estrone-3-sulfate or urate in exchange for intracellular (α-keto)glutarate (“influx mode”). Intracellular organic anions such as PAH are effluxed through OAT4, probably in exchange for external chloride (“efflux mode”).
As reviewed in, OAT4 interacted with β-lactam antibiotics, angiotensin II receptor-1 blockers, methotrexate, diuretics, NSAIDs, and was inhibited by probenecid. The secretion of diuretics such as torasemide can lead to an increased reabsorption of urate due to diuretic/urate antiport at OAT4, explaining the observation that therapy with diuretics can cause hyperuricemia. NPT4 is yet another site for an interaction between diuretics and urate (see later).
Taking together, OAT4 in the apical membrane of proximal tubule cells can contribute to the absorption of estrone sulfate and urate from the primary urine, and to the secretion of anionic drugs. Since OAT4 is not present in rodents it is not possible to learn more about its function from knockout animals.
The Organic Anion Transporter 5 (Oat5, Slc22a19 )
Oat5 was cloned from rat and mouse in which it was restricted to the kidney and was equally expressed in male and female animals. There is no human homologue to Slc22a19. The Oat5 protein was localized to the apical membrane in the late proximal tubule segments. The interaction of Oat5 with dicarboxylates is not yet settled, because conflicting results were reported on the ability of succinate to trans -stimulate uptake of estrone-3-sulfate. Endogenous substrates were dehydroepiandrosterone sulfate and estradiol sulfate, but not prostaglandins and urate. A few drugs were tested: furosemide, benzylpenicillin, diclofenac, and ibuprofen inhibited Oat5. As long as the driving force for Oat5 is not defined it is not possible to speculate on the role of this transporter in drug secretion or absorption.
The Organic Anion Transporter 10 (OAT10/Oat10; SLC22A13/Slc22a13 )
OAT10 (previously cloned as orphan transporter ORCTL3) is predominantly expressed in human kidneys and weakly in brain, heart and colon. The gene is located on chromosome 3p22.2. Western blots showed the rOat10 protein in the apical, but not in the basolateral membrane, and female animals showed a stronger expression of rOat10 than did males. Endogenous substrates of OAT10 were nicotinate, lactate, urate, succinate, and glutathione. Succinate served as counter anion for nicotinate and urate uptake from the lumen. Among the few drugs tested, furosemide, hydrochlorothiazide, sulfinpyrazone, and cyclosporine A inhibited OAT10. It needs to be established whether OAT10 is involved in cyclosporine A-induced nephropathy. The physiological role of OAT10 is probably the uptake of the vitamin nicotinate in small intestine and renal proximal tubule cells.
The Urate Anion Transporter 1 (URAT1/Urat1/Rst; SLC22A12/Slc22a12 )
URAT1/Urat1/Rst was cloned from human and mouse kidneys (for reviews see ). The gene for human URAT1 is located on chromosome 11q13.1 adjacent to that of OAT4. The highest though not exclusive expression was found in kidneys. Human and mouse URAT1/Urat1 were immunolocalized to the apical membrane of proximal tubule cells where they interact with the scaffolding protein PDZK1. Male mice showed a higher expression than female animals, indicating sex differences at least in this species.
Hepatocyte nuclear factor-1α and -1β induced the expression of human and mouse URAT1/Urat1 promoter, and mUrat1 expression was diminished in a HNF-1α knockout mouse. Furthermore, the mUrat1 promoter was found to be hypomethylated, suggesting a tissue-specific epigenetic control (reviewed in ).
URAT1 mutations have been linked to familial hypouricemia. Most of the hypouricemic patients in Japan and Korea carry a truncation mutation, W258X. Meanwhile, several more amino acid exchanges have been described (see for a compilation). Some mutations occur also with hyperuricemia and gout, but the molecular mechanism is unknown.
URAT1 expressed in oocytes displayed saturable uptake of urate. Extracellular chloride inhibited, and intracellular chloride trans -stimulated urate uptake, indicating that URAT1 can operate as urate/chloride exchanger. Preloading of oocytes with lactate trans -stimulated urate uptake, indicating urate/lactate exchange as another transport mode of URAT1. The endogenous compounds L- and D-lactate, acetoacetate, β-hdyroxybutyrate, succinate (but not α-ketoglutarate), and orotate inhibited urate uptake. Exogenous compounds inhibiting URAT1 were the uricosuric drugs probenecid, benzbromarone, losartan; the antiuricosuric drugs pyrazine carboxylate and pyrazine dicarboxylate; the loop diuretics furosemide and bumetanide; the NSAIDs phenylbutazone, salicylate and indomethacin; and the vitamin nicotinate. PAH and estrone sulfate did not affect URAT1.
Also mouse Urat1/Rst transported urate ( K m 1.2 mM) and performed urate/chloride, urate/lactate and urate/pyrazinoate exchange. Uptake of labeled urate was inhibited, when the uricosurics probenecid and benzbromarone, the uremic toxins indoxyl sulfate, indole acetate, hippurate, and a number of acidic neurotransmitter metabolites were present in the medium.
In summary, URAT1/Urat1 is involved in urate absorption from the primary urine. Thereby, urate uptake is driven by exchange against lactate that is transported into the cells by a sodium-lactate symporter in the apical membrane, as has been convincingly demonstrated in a c/ebpδ knockout mouse. This mouse does not express the sodium-coupled lactate transporters Slc5a8 and Slc5a12 in the apical membrane of proximal tubules and excretes lactate and—at unchanged Urat1 expression—urate with the urine. In man, uricosurics inhibit URAT1, and loss-of-function mutations cause an increased urate clearance and a reduction in serum level of urate.
The Voltage-Sensitive Organic Anion Transporter 1 (Oat v 1/Npt1; Slc17a1 )
Searching for the voltage-driven transporter for PAH and urate in urate-secreting species (rabbits, pigs), Oat v 1 was identified by expression cloning from pig kidneys. The message for pOat v 1 was restricted to liver and kidneys. pOat v 1 is not a member of the SLC22 family, but showed highest identity to human and rodent NPT1/Npt1 ( SLC17A1/Slc17a1 ), previously cloned as sodium-dependent phosphate transporters from human, rabbit, and mouse kidneys and located to the apical membrane of the proximal tubule segments S1–S3 (for review see ). The human gene for NPT1 was found on chromosome 6p21.3-p23. The expression of mNpt1 was under control of heptatocyte nuclear factor-1α, and HNF-1α knockout mice had greatly diminished proximal tubular Npt1 expression. Fasting decreased and streptozotocin-induced diabetes increased renal and hepatic expression of NaPi-1/Npt1 in rats, respectively. Whether gender differences exist is not known.
Human NPT and mouse Npt1/NaPi-1 performed the uptake of radiolabeled organic anions including PAH, urate, estradiol-17β-D-glucuronide, benzylpenicillin, faropenem, and mevalonate. Benzylpenicillin, ampicillin, cephalexin, cefazolin, indomethacin, furosemide, benzoate, lactate, and probenecid inhibited uptake of labeled faropenem by mNpt1. Uptake of labeled PAH by porcine Oat v 1 was inhibited by, e.g., estrone sulfate, penicillin G, salicylate, indomethacin, ibuprofen, diclofenac, furosemide, bumetanide, and probenecid. Organic anion transport was independent of sodium, but was inhibited by increasing chloride concentrations as well as by DIDS and NPPB.
It is likely that organic anion transport through NPT1/Npt1 is electrogenic. Busch et al. observed outward currents upon addition of benzylpenicillin, phenol red and probenecid in Xenopus laevis oocytes expressing rbNaPi-1 (Npt1 ). PAH uptake ( K m 4.4 mM) by pOat v 1/pNpt1 was increased at inside positive membrane potentials. More recently, mNpt1 was purified and inserted into proteoliposomes for functional characterization. Both, sodium-dependent phosphate transport and sodium-independent PAH and urate transport were observed, indicating that at least mNpt1 is able to carry out both functions. The affinity of mNpt1 for urate and PAH was similar ( K m 1.1 mM) and uptake was accelerated by a proteoliposome-inside positive membrane potential. Chloride stimulated (whereas it inhibited in other studies) PAH uptake, but was not transported itself. Salicylate, aspirin and acetaminophen, but not other NSAIDs, inhibited PAH and urate transport; radiolabeled aspirin was translocated by reconstituted mNpt1.
The mutation of an arginine residue (R138A) conserved within the SLC17 family abolished transport. A lowered V max was found with the T269I mutation that corresponds to a SNP in human NPT1 gene associated with gout. Taken these data together it is likely that NPT1/Npt1/Oat v 1 is involved in the voltage-driven exit of organic anions including urate, PAH, β-lactam antibiotics, diuretics and some non-steroidal anti-inflammatory drugs.
The Sodium-Phosphate Transporter 4 (NPT4/Npt4; SLC17A3/Slc17a3 )
Exploring the locus for hereditary hemochromatosis in humans, two genes closely related and juxtapositioned to SLC17A1 were found on chromosome 6p21.3 and sequenced. One of these genes, SLC17A3 , was expressed only in liver and kidneys and the gene product was named NPT4. In mice deficient in HNF-1α, the expression of Npt4 in kidneys was reduced, indicating that, like Npt1 (see previous chapter), Npt4 is under control of this hepatic nuclear transcription factor. Npt4 was also isolated from rat kidneys where it was immunolocalized to the apical membrane of proximal tubule cells of the segments S2 and S3; segment S1 following directly the glomerulus did not show any labelling. Human NPT4 was also localized to the apical membrane of proximal tubule cells.
When human NPT4 was expressed in COS cells, a perinuclear localisation was found; the authors speculated that NPT4 is involved in the release of phosphate from the endoplasmic reticulum, and loss of its function should give rise to the glycogen storage disease type 1c. Indeed, a patient with this disease showed a non-synonymous SNP, resulting in a Gly201Arg mutation. The functional consequences of this mutation were, however, not tested.
Single nucleotide polymorphisms of the human SCL17A3 gene correlated with hyperuricemia. The linkage was not as strong as with SNPs affecting the urate transporter GLUT9 and the breast cancer resistance protein ABCG2 and, in later studies, failed to reach statistical significance or were associated with an increase in serum uric acid only in women.
When rat Npt4 was expressed in Xenopus laevis oocytes it showed a sodium-dependent phosphate transport. Expression of human NPT4 also in oocytes resulted in the uptake of labelled estrone-3-sulfate, estrone-17β-D-glucuronide, prostaglandin E 2 , taurocholate, PAH, bumetanide, and ochratoxin A. Uptake was stimulated by depolarization, and PAH and bumetanide application caused an outward current in voltage-clamped oocytes. PAH uptake was inhibited by the hormones DHEAS, β-estradiol sulfate, estriol, estrone-3-sulfate; the diuretics hydrochlorothiazide, chlorothiazide, trichlormethiazide, bumetanide, furosemide, spironolacton; the NSAIDs diclofenac, ibuprofen and indomethacin; and the common inhibitor probenecid. No interaction with NPT4 was seen for succinate, α-ketoglutarate, lactate, nicotinate, xanthine, and hypoxanthine.
Importantly, labelled urate was transported by NPT4. In Japanese hyperuricemic patients, two mutations (N68H and F304S) were found in NPT4. Expression of these mutants in Xenopus laevis oocytes resulted in a decreased urate uptake as compared to oocytes expressing the wildtype NPT4. Thus, it is likely that NPT4 constitutes the voltage-driven exit step for urate secreted into proximal tubules. A decreased function of NPT4 will decrease urate secretion and lead to hyperuricemia.
The Organic Anion Transporting Polypeptides, Subfamily 1A (OATP1A/Oatp1a; SLCO1A/Slco1a )
The organic anion transporting polypeptides were grouped into 6 families, OATP1-6. The genes (formerly SLC21) have been systematically renamed to SLCO followed by the number of OATP/Oatp. Human OATP1A2 (SLCO1A2; formerly OATP-A; SLC21A3) was localized to the apical membranes of distal tubules and, hence, is not involved in proximal tubular drug secretion. Of the rat homologues, rOatp1a1 (Slco1a1; formerly Oatp1) and rOatp1a3 (Slco1a3; formerly OAT-K1/K2) have been localized to the apical membrane of proximal tubule cells. Oatp1a1 expression in rats and mice was higher in males than in females and, in mice, mRNA appeared late in adult animals. Rat Oatp1 transported bile salts, hormones and their conjugates, peptides, drugs, toxins, and even organic cations (reviewed in ). Since it appears that Oatp1 is rather involved in drug uptake across the apical membrane rather than in drug secretion, we do not further consider this transporter in this review.
Oat-K1 and its N-truncated variant Oat-K2 were isolated as rat renal homologues of Oatp1a1 (reviewed in ). In the new nomenclature, Oat-K1 was classified as Oatp1a3 (gene Slco1a3; formerly Slc21a4). The message for rOat-K1 was detected exclusively in kidneys and was located to superficial and juxtamedullary proximal straight tubules (S3 segment ). Antibodies reacted with a 40 kDa band in rat renal brush-border membranes, indicating the expression of the rOat-K1 protein in the apical membrane. The mRNA for the splice variant rOat-K2 was detected mainly in the S3 segment, but also in proximal convoluted tubules as well as in collecting ducts. When expressed in MDCK cells, rOat-K2 appeared in the apical membrane, suggesting that both transporters are located in the apical membrane.
Rat Oatp1a3/Oat-K1 mediated the sodium-and chloride-independent, bidirectional transport of methotrexate (K m 2.1 µM) and folate (substrates reviewed in ). Further transported substrates were the hormone conjugates dehydroepiandrosterone sulfate, estrone-3-sulfate, estradiol-17β-D-glucuronide; the thyroid hormones triiodothyronine and thyroxin; the bile salt taurocholate; and the mycotoxin ochratoxin A. PAH inhibited methotrexate transport but was not translocated. A series of non-steroidal anti-inflammatory drugs inhibited methotrexate uptake, but were not translocated. Although truncated, rOat-K2 was functional and translocated methotrexate, folate, taurocholate, and prostaglandin E 2 . In addition transport of dehydroepiandrosterone sulfate, estrone sulfate, estradiol-17β-D-glucuronide, triiodothyronin, thyroxine, ochratoxin A, and the antiviral drug zidovudine was shown.
Taken together, rat Oatp1a3/Oat-K1 and Oat-K2 interact with endogenous compounds and selected drugs, methotrexate and zidovudine. Oatp3a1/Oat-K1 and K2 were considered important for renal methotrexate secretion. The inhibition by NSAIDs could provide an explanation for the toxic accumulation of methotrexate in the body, when co-administered with analgesics.
The Multidrug Resistance Protein 2 (MRP2/Mrp2; ABCC2/Abcc2 )
The ATP binding cassette (ABC) transporter MRP2/Mrp2 is mainly expressed kidneys, liver, placenta and small intestine (for reviews see ). Highest expression was found in human liver and jejunum; in human kidneys, mRNA for MRP2 was moderately expressed as compared to the highly expressed OAT1 and OAT3. The human gene, ABCC2, is located on chromosome 10q24. In mice, mRNA for Mrp2 was low until day 10 after birth; from day 15 on, the mRNA was higher and did not show statistically significant sex differences in adulthood.
MRP2/Mrp2 is a large protein consisting of 1,545 amino acids arranged in three membrane spanning domains, MSD0, MSD1 and MSD2, with 5, 6, and 6 transmembrane helices (TMH) each, respectively. Between MSD1 and MSD2 and carboxyterminal from MSD2 there are two intracellularly located nucleotide binding domains (NBDs). In human MRP2, TMH 6, 9, 16, and 17, and in rMrp2 TMH 11, 14, and 15 are important for function (for literature see ). In all organs, MRP2 protein was found at the apical membrane. Within human and rat kidneys, MRP2/Mrp2 is located in the proximal tubules in segments S1 to S3 and thus prone to ATP-driven extrusion of its substrates.
Several loss-of-function mutations of MRP2 are the cause of the Dubin-Johnson syndrome in humans, a rare autosomal-recessive disease with hyperbilirubinemia and deposition of a dark pigment in the liver (for a review of mutations see ). Mrp2 is absent in two rat strains, the Eisai hyperbilirubinemic rat and the TR – rat that served as models for the Dubin-Johnson syndrome and offered valuable insights into the physiological function of MRP2/Mrp2. More recently, Mrp2 knockout mice were generated which were also hyperbilirubinemic (summarized in ). The increased serum levels in bilirubin diglucuronide in MRP2/Mrp2 deficient humans, rats, and mice is due both to an impaired secretion of hepatic bilirubin diglucuronide across the canalicular membrane into the bile and to an increased release of this metabolite through the upregulated Mrp3 and Mrp4 across the sinusoidal membrane into the blood. The functional significance of several other single nucleotide polymorphisms observed in human MRP2 is not yet clear. The SNP 1249G>A that leads to the amino acid exchange Val417Ile is frequent in Caucasians (22%) and Japanese (13%) and possibly associated with a renal tubulopathy induced by the antiviral drug tenofovir. The SNP -24C>T in the promoter region was found to cause a lower renal MRP2 expression and a decreased excretion of methotrexate.
MRP2/Mrp2 is an ATP-driven pump for the endogenous compounds leukotrienes C 4 , D 4 and E 4 , mono- and bis-glucuronidated bilirubin, estradiol-17β- D -glucuronide, glutathione, glutathione-conjugates, and conjugated bile salts. Exogenous compounds being transported by or interfering with MRP2/Mrp2 include β-lactam antibiotics, statins, NSAIDs, HIV drugs, and a number of anticancer drugs (reviewed in ). The clinical importance of MRP2 is based on its ability to confer resistance to a number of cytostatic drugs. ln proximal tubules, MRP2/Mrp2 is expected to pump glutathione, glucuronide and sulfate conjugates into the urine, utilizing ATP hydrolysis as energy source. When human MRP2 was expressed in HEK293 cells and inside-out vesicles were prepared from these cells, an ATP-driven uptake of radiolabeled PAH with low affinity was found.
The Multidrug Resistance Protein 4 (MRP4/Mrp4; ABCC4/Abcc4 )
The mRNA for MRP4/Mrp4 was low in several organs and showed the highest levels in kidneys and prostate. The human gene for this ABC transporter, ABCC4 , is located on chromosome 13q32. In mice, the mRNA was present at their birth; at day 15 after birth, a small increase in mRNA levels took place and from day 30 on, expression in female mice exceeded that in male mice considerably, indicating sex differences in adult animals. The female-dominant mMrp4 expression was verified in Western blots. In gonadectomized mice, 5-dehydrotestosterone application decreased mRNA, suggesting that androgens repress the expression of Mrp4. In contrast to mice, rMrp4 showed a male-dominant expression in adult rats. It is unknown whether sex differences exist in human MRP4 expression. In human kidneys, MRP4 was localized to the apical membrane of proximal tubule cells.
Hepatic mMrp4 mRNA expression was regulated through the constitutive androstane receptor (CAR) and the peroxisome proliferator-activated receptor α (PPARα), leading to an increased expression during cholestasis. In mouse kidneys, ischemia-reperfusion injury increased the mRNA, but decreased the Mrp4 protein level, and in rat kidneys, endotoxemia did not change mRNA, but again decreased protein levels, suggesting that a post-translational regulation takes place. Conflicting results have been obtained with Mrp2-deficient TR¯ rats with either no change or an increased rMrp4 expresssion; in Mrp2 knockout mice, mMrp4 was upregulated (summarized in ).
Several single nucleotide polymorphisms have been reported for the MRP4 gene in Caucasians and Japanese (collected in ). Although a number of non-synonymous SNPs lead to amino acid exchanges in conserved regions, no influence on expression and localization of MRP4 was found in liver; functional consequences of these mutations were not studied so far.
MRP4/Mrp4 has only two membrane-spanning domains (MSD1 and MSD2) and lacks MSD0. Thereby, MRP4/Mrp4 is with 1325 amino acids the shortest member of the ABCC family. Morever, MRP4/Mrp4 differs from MRP2/Mrp2 in its ability to transport cyclic nucleotides and nucleoside analogues. Cells overexpressing human MRP4 showed a unique resistance to 9-(2-phosphonylmethoxyethyl)adenine (PMEA), 9-(2-phosphonylmethoxyethyl)guanine (PMEG), and azidothymidine (AZT), suggesting that this ATP-driven transporter translocates nucleoside-based antiviral drugs across the cell membrane. Numerous endogenous compounds are substrates of MRP4/Mrp4: cAMP, cGMP, prostaglandins (PGE 1 , PGE 2 , PGF 2α ), leukotrienes (LTB 4 , LTC 4 ), unconjugated (cholate) and conjugated bile acids, (e.g. taurocholate, glycocholate), conjugated steroids (dehydroepiandrosterone sulfate, estradiol-17βD-glucuronide), folic acid, and glutathione. Thereby, cholate, glycocholate, taurocholate, LTB 4 are cotransported with glutathione. In addition, MRP4 turned out as an ATP-driven urate transporter. Since urate transport showed a Hill coefficient of 1.7 it is likely that two molecules of urate may be transported per cycle. Possibly, there are two independent transport sites interacting allosterically. Together with OAT v 1/NPT1 and NPT4, MRP4 is involved in urate secretion in proximal tubules.
Human MRP4 transported a number of drugs including antibiotics (ceftizoxime, cefazolin, cefotaxime, cefmetazole), diuretics (hydrochlorothiazide, furosemide), antihypertensives (enalapril, olmesartan), statins (pravastatin), antivirals (adefovir, tenofovir), and anticancer drugs (methotrexate, topotecan). Non-steroidal anti-inflammatory drugs (indomethacin, sulindac), and probenecid inhibited MRP4. The decreased renal excretion of ceftizoxime, adefovir, tenofovir, hydrochlorothiazide, and furosemide in Mrp4 knockout mice proved an important role of Mrp4 in drug secretion.
TR − rats lacking Mrp2 in liver and kidneys, did not show a lower PAH clearance than wildtype rats, suggesting that the contribution of rMrp2 to urinary PAH excretion is low. Indeed, human MRP4 transported PAH with a K m of 160 µM, whereas MRP2 exhibited a much lower affinity. ATP-driven PAH transport by MRP4 was sensitive to probenecid, sulfinpyrazone, dipyridamole, and acyclovir. The expression of MRP4 protein (tested by Western blots) was fivefold higher than that of MRP2. Together with the approximately 30 fold higher affinity for PAH, MRP4 should clearly dominate over MRP2 in the efflux of PAH across the apical membrane.
Overview of Transporters Involved in Transport of Selected Anionic Drugs
β-Lactam Antibiotics
Most β-lactam antibiotics are excreted by the kidneys and undergo proximal tubular secretion. Benzylpenicillin (BP in Figure 72.2 ) is taken up across the basolateral membrane into proximal tubule cells mainly by OAT3, because OAT3 has a higher affinity for this antibiotic than OAT1. Since OAT4, NPT1, and MRP2 interacted with benzylpenicillin, one or more of these transporters may be involved in its exit across the apical membrane. However, the quantitative contribution of the apical transporters to overall benzylpenicillin secretion is not clear at present. Most cephalosporines (CS in Figure 72.2 ) interacted with OAT1 with higher affinity than with OAT3, and OAT2 had a very low affinity, rendering OAT1 the prime candidate for uptake across the basolateral membrane. OAT4 was inhibited by cephalosporines with low affinity, leaving it open whether this transporter contributes much to cephalosporin exit across the apical membrane. A considerably higher affinity for ceftizoxime, cefazolin and cefmetazole strongly suggests that MRP4 is the most important transporter for the release of cephalosporines from tubule cells into the urine.

Antiviral Drugs
Many antiviral drugs (AVD in Figure 72.3 ) are renally excreted and interact with organic anion transporters, irrespective whether they carry a negative charge, such as adefovir, cidofovir, 9-(-2-phosphonylmethoxyethyl)guanidine (PMEG) and 9-(2-phosphonoethyl)diaminopurine (PMEDAP), or are uncharged, e.g. acyclovir, ganciclovir and zidovudine. Uptake of these drugs across the basolateral membrane is largely mediated by OAT1; only zidovudine was transported also by OAT2 and OAT3 ( Figure 72.3 ). Hence, OAT1 is most probably responsible for the dose-limiting, nephrotoxic effects of these compounds. Although OAT4 translocated zidovudine it is more likely that MRP4 is the efflux transporter at the apical membrane, because it afforded a resistance against several antiviral drugs. In addition, Mrp4 knockout mice accumulated more adefovir and tenofovir in their kidneys and excreted less with the urine.

Non-Steroidal Anti-Inflammatory Drugs (NSAIDs)
Most NSAIDs are preferentially excreted by the liver. However, they interact with renal organic anion transporters, and some of them have been shown to be translocated, i.e., indomethacin by human and rat OAT1/Oat1 and acetylsalicylate and salicylate by rOat1. For all other NSAIDs, an inhibition of OAT1/Oat1 mediated transport was found. In general, the affinity of human OAT1 decreased with increasing hydrophilicity of the NSAIDs. Since labeled acetylsalicylate, indomethacin, and salicylate were transported by rOat2, and salicylate by hOAT3, these transporters may also be involved in uptake of selected NSAIDs from blood into proximal tubule cells. Again, inhibition by various NSAIDs of both transporters were observed.
Interestingly, it has been proposed that NSAIDs should be used to decrease the toxicity of antiviral drugs by drug interaction at OAT1. An interaction at OAT3 was proposed to be involved in the potentially life-threatening accumulation of methotrexate in patients taking NSAIDs (for literature see ), In this regard, it is important to compare therapeutic plasma levels of NSAIDs with the affinity of OATs. Only the plasma concentrations of salicylate, phenylbutazon, indomethacin, and probenecid are high enough to inhibit OAT3.
NSAIDs were also tested with organic anion transporters present in the apical membrane. OAT4 was inhibited by diclofenac, ibuprofen, ketoprofen, phenylbutazone, but not, or only weakly, by salicylate (see ). Oat v 1-mediated transport was inhibited by salicylate, indomethacin, ibuprofen, and diclofenac. The human NPT1 showed a weak transport of labeled indomethacin. Human NPT4 was inhibited by diclofenac, ibuprofen and indomethacin. For rOat-K1, an interaction with indomethacin, ketoprofen, ibuprofen, flufenamate, phenylbutazone, but not of salicylate was reported. Oat-K2, however, did not translocate indomethacin. The competition for Oat-K1 could contribute to the above mentioned methotrexate/NSAID interaction. Several NSAIDs including flurbiprofen, ibuprofen, indomethacin, indoprofen, ketoprofen and diclofenac inhibited MRP4. It is not known, however, whether these compounds inhibit only or are indeed translocated by MRP4. Since methotrexate is translocated by MRP4, inhibition by NSAIDs could contribute to the accumulation of methotrexate in patients treated with analgesics.
In summary, NSAIDs interfere with organic anion transporters in proximal tubule cells. Although renal excretion is not the main route for elimination of most NSAIDs, some of these compounds can inhibit the secretion of other organic anions such as the cytostatic drug methotrexate and cause side effects. On the other hand, NSAIDs may deliberately be used to decrease the nephrotoxicity of, e.g., antiviral drugs during HIV therapy.
Diuretics
Diuretics are secreted in proximal tubules to reach their target salt transporters in the distal tubule from the lumenal side. Among the human basolateral OATs, OAT1 translocated labelled bumetanide and furosemide, OAT2 bumetanide, and OAT3 furosemide; all other diuretics inhibited (see for K i values). OAT1 possessed a higher affinity for most thiazide diuretics (TZ in Figure 72.4 ), whereas OAT3 had a higher affinity for loop diuretics (LD in Figure 72.4 ). Both Oat1 and Oat3 knockout mice showed a decreased renal excretion of furosemide and bendroflumethiazide, strongly suggesting that both transporters are involved in proximal tubular secretion of diuretics.

At the lumenal membrane, it is likely that OAT4 is involved in the efflux of diuretics into the urine. OAT4 transported labeled bumetanide and was inhibited by thiazides and loop diuretics (see ). The export of diuretics, e.g., torasemide, in exchange for urate could explain the hyperuricemia observed in antihypertensive monotherapy with diuretics. An interaction of furosemide with rOat-K1 and rOat-K2, pOat v 1, and hMRP2 was reported. However, it is not known whether any of these transporters translocates furosemide and other diuretics across the apical membrane. NPT4, however, is clearly a candidate for the release of diuretics, because thiazides and loop diuretics inhibited NPT4-mediated PAH transport and labelled bumetanide was taken up into NPT4-expressing oocytes. Since extracellular bumetanide induced an outward current it is highly likely that transport is electrogenic. Given the inside negative electrical potential difference across the apical membrane, NPT4, probably acts as an important exit transporter for the negatively charged diuretics.
Organic Cation Transport Systems
Characteristics of Organic Cation Transport in Proximal Tubules
In the kidney, organic cations may be ultrafiltrated in the glomeruli and reabsorbed or secreted in renal tubules. Hydrophilic organic cations that do not bind to plasma proteins are readily filtrated and may be reabsorbed in renal tubules. However, most organic cations are not filtrated efficiently because they are relatively hydrophobic and bound to plasma proteins. These organic cations are actively secreted. Reabsorption and secretion of organic cations have been investigated in proximal tubules, distal tubules, and collecting ducts, however, secretion of organic cations in proximal tubules has been studied in most detail. Substrates for organic cation secretion include primary, secondary, tertiary, or quaternary amines that have a positive charge at physiological pH. Organic cations are secreted by a two-step procedure. In the first step, they are taken up across the basolateral membrane by an electrogenic transport system that accepts a variety of structurally different organic cations and uses the electrochemical gradient to accumulate cations intracellularly. The energy provided by the membrane potential is restored by the Na + ,K + -ATPase. In a second step, organic cations are released across the luminal membrane by a proton/organic cation antiporter that helps to overcome the inside negative membrane potential. This step is energized by an extracellular over intracellular proton concentration difference that is mainly generated by the Na + /H + exchanger. For reabsorption, organic cations are transported across the luminal membrane and leave the cells via a polyspecific transporter in the basolateral membrane. Renal handling of organic cations is dependent on their concentrations in the plasma. For example, in rabbit and dog, choline is secreted at high plasma concentrations, whereas it is reabsorbed at normal plasma concentrations below 25 µM.
Figure 72.5 depicts transporters that mediate secretion and reabsorption of organic cations in human renal proximal tubules. The Na + ,K + -ATPase in the basolateral membrane generates and maintains a high intracellular concentration of potassium, which is the prerequisite for the membrane potential that drives organic cation uptake by the electrogenic organic cation transporters. The extracellular-over-intracellular proton gradient across the luminal membrane generated by the sodium/proton antiporter provides the driving force for the proton/organic cation exchangers that mediate the efflux of organic cations into the tubular lumen. Transporters OCT1 (SLC22A1), OCT2 (SLC22A2), OCT3 (SLC22A3), OCTN1 (SLC22A4), and OCTN2 (SLC22A5) belong to the organic cation transporter family SLC22 which is a member of the major solute facilitator (MFS) superfamily. MATE1 ( SLC47A1 ) and MATE2-K ( SLC47A2 ) are members of the multidrug and toxin extrusion family SLC47 whereas MDR1 (ABCB1) belongs to the ATP-binding cassette transporter family ABC.

Cloning and Functions of OCT1-3/Oct1-3; SLC22A1-3/Slc22a1-3
OCT1/Oct1, OCT2/Oct2, and OCT3/Oct3 are electrogenic polyspecific transporters that are expressed in kidney. On the protein level, individual transporter subtypes from different species exhibit cross-species identities of 78 to 95%. Within a given species, the amino acid identities between different subtypes are 67 to 70% between OCT1 and OCT2, 47 to 57% between OCT1 and OCT3, and 49 to 51% between OCT2 and OCT3. In addition to renal excretion and reabsorption, OCT1–3 are critically involved in small intestinal absorption and hepatic excretion of cationic drugs. The OCT-transporters influence the distribution of cationic drugs and endogenous compounds in various tissues, for example in brain and heart. Species-specific expression and membrane locations of the three OCT subtyes have been observed. In humans, OCT2 is most strongly expressed in kidneys whereas OCT1 and OCT3 are most strongly expressed in liver and adrenal gland, respectively.
OCT1/Oct1, OCT2/Oct2, and OCT3/Oct3 have been isolated from rats, mice, and humans. Oct1 and Oct2 were also cloned from rabbit and Oct2 also from pig.
Expression and Main Functions of OCT1/Oct1
In the species tested so far, OCT1/Oct1 is mainly expressed in liver where it is located at the sinusoidal membrane of the hepatocytes. In rat, mouse, and rabbit, strong expression of Oct1 was also observed in kidneys whereas relatively small amounts of OCT1 mRNA were detected in human kidney. In humans, OCT1 is also expressed in various other organs and in various tumors. Using immunohistochemistry, Oct1 protein was localized at the basolateral membranes of S1 and S2 segments of rat proximal tubules, however, in human OCT1 has been recently localized to luminal membranes of proximal and distal tubules. Apical location of OCT1/Oct1 has been also observed in lung bronchial epithelial cells and brain microvessel endothelial cells of rodents and human. Human OCT1 transports the endogenous Cyclo(His-Pro) and salsosinol, xenobiotics and drugs ( and Table 72.1 ). Important transported drugs are the antidiabetic metformin; the antineoplastic drugs oxaliplatin, picoplatin and metaiodobenzylguanidine; the antiparasitic drugs furaminidine and pentamidine; and the antiviral drugs acyclovir, ganciclovir, lamuvidine and zalzitabine ( Table 72.1 ). Studies with mice in which either Oct1, Oct2 or Oct3 or Oct1 plus Oct2 were removed indicated the importance of Oct1 and Oct2 for renal secretion of tetraethylammonium (TEA) and of the antidiabetic metformin. In humans with defect mutations in OCT1, increased renal excretion of metformin was observed, suggesting that OCT1 is critically involved in renal reabsorption rather than in excretion of organic cations.
Compound | hOCT1 | hOCT2 | hOCT3 | hOCTN1 | hOCTN2 | MATE1 | MATE2-K | MDR1 | |
---|---|---|---|---|---|---|---|---|---|
Antiallergic | Fexofenadine | substrate | substrate | ||||||
Anestheticantiarrhythmic | Lidocaine | 294 | K m :139 | 0.8 | |||||
Antiarrhythmic | Procainamide | 15–74 | 28–406 | 355–738 substrate | (<500) | (<300) | K m : 1230 | K m : 1580 , or 4100 | |
Antiarrhythmic | Quinidine | 5.4–114 substrate | 7.1–446 | K m : 216 | 69 | <100 | 29 | 23 | Substrate |
Antiarrhythmicvasodilator | Verapamil | 1.2–2.9 | 13–85 | 8.4 substrate | <50 substrate | 28 | 32 substrate | Substrate | |
Antibacterial | Cephaloridine | <1000 | K m :230 | ||||||
Antibacterial | Levofloxacin | substrate | |||||||
Antibacterial | Tetracycline | substrate | |||||||
Anticonvulsanr | Gabapentin | substrate | |||||||
Antidiabetic | Metformin | K m : 1470–2160 | K m : 990–1380 | K m : 2260 | K m : 780 | K m : 1050–1980 | |||
Antifungal | Salicylic acid | substrate | |||||||
Antihypertensive | Captopril | substrate | |||||||
Antihypotensive | Etilefrine | 447 | 4009 | K m : 2800 | |||||
Antineoplastic | Cisplatin | 1000–5000 | 1.5–10000 substrate | 1000–5000 | 1000–5000 substrate | >4000 substrate | |||
Antineoplastic | Metaiodoben-zylguanidin | substrate | substrate | substrate | |||||
Antineoplastic | Oxaliplatin | substrate | substrate | substrate | substrate | ||||
Antineoplastic | Picoplatin | substrate | substrate | substrate | |||||
Antineoplastic | Topotecan | K m : 70 | K m : 60 | Substrate | |||||
Antimalarial | Quinine | 13–52 substrate | 3.4–34 substrate | 37 | 2500 | <100 | substrate | substrate | |
Antiparasitic | Furaminidine | K m : 6.1 | 182 | 20 | |||||
Antiparasitic | Pentamidine | K m : 36 | 3.8–11 | 15 | |||||
Anti-ulcer | Cimetidine | 95–166 | K m : 67–73 | 240 | 435 | <2000 | K m : 170 | K m : 120–370 | Substrate |
Anti-ulcer | Famotidine | K m : 56 | ~20 | 0.6 | 9.7 | ||||
Anti-ulcer | Ranitine | 22–28 | K m : 265 | 372 | 25 | 25 | |||
Antiviral | Acyclovir | K m : 151 | K m : 2640 | K m : 4320 | |||||
Antiviral | Ganciclovir | K m : 516 | K m : 5120 | K m : 4280 | |||||
Antiviral | Lamuvidine | K m : 249–1250 | K m : 248–1900 | K m : 2140 | |||||
Antiviral | Tenofovir | 9 x 10 −4 | 6 x 10 −4 | 5 x 10 −6 | substrate | ||||
Antiviral | Zalcitabine | K m : 242 | K m : 232 | ||||||
Anti-Parkinson | Amantadine | 18–236 | K m : 27 | >1000 | 112 | 1167 | |||
Anti-Parkinson | Memantine | 3.7–27 | K m : 34 | 236 | |||||
Cardioprotective | Mildronate | K m : 26 |
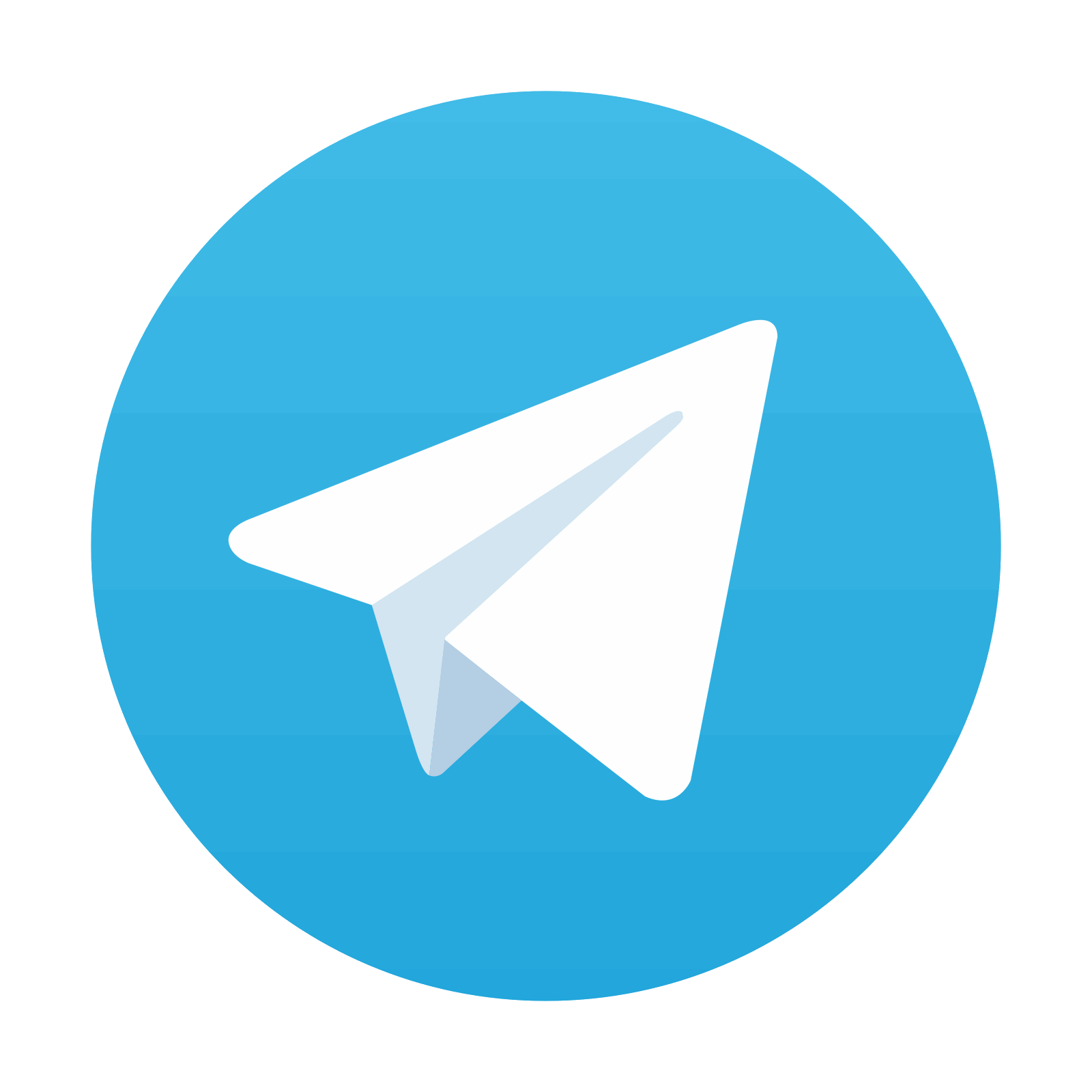
Stay updated, free articles. Join our Telegram channel

Full access? Get Clinical Tree
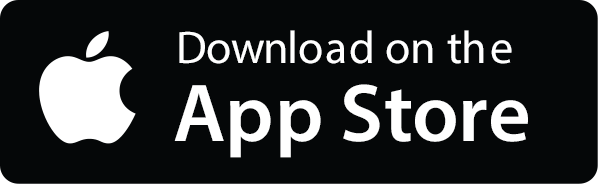
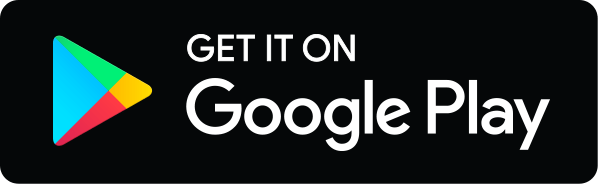
