Magnesium plays a vital role in virtually all cellular processes as a cofactor of enzymes, a structural element of proteins and nucleic acids, and a modulator of receptors and ion channels. The control of magnesium homeostasis primarily resides in the kidney tubules. Nephron segments critical for renal magnesium conservation mainly comprise the thick ascending limb and the distal convoluted tubule where different control mechanisms act. Over the past two decades, genetic and molecular studies in patients with hereditary forms of hypomagnesemia led to the identification of important components of epithelial magnesium transport. These discoveries have greatly advanced our understanding not only of inherited but also of acquired disorders of magnesium metabolism at the molecular level. This chapter highlights the different transport pathways involved in tubular magnesium reabsorption and their regulation; it also summarizes important discoveries in hereditary disorders of renal magnesium handling and provides an overview on acquired forms of hypomagnesemia.
Introduction
Body magnesium homeostasis depends on the balanced regulation of intestinal magnesium absorption and renal magnesium conservation. Renal magnesium handling is a filtration–reabsorption process, as there is little evidence of secretion along the nephron segments ( Fig 61.1 ). About 80% of the total plasma magnesium (0.65–1.2 mM) is filtered through the glomeruli. Of the ultrafilterable magnesium (0.5–0.9 mM), 15–20% is reabsorbed by the convoluted and straight portions of the proximal tubule. The thick ascending limb (TAL) of the loop of Henle, specifically the cortical segment, plays a major role in reclaiming filtered magnesium (55–70%), whereas only 5–10% are reabsorbed in the distal convoluted tubule (DCT). However, the DCT provides the selective regulation of magnesium reabsorption and plays an important role in determining the final urinary excretion. About 3–5% of the filtered load normally appear in the urine. Magnesium transport in both the TAL and DCT responds to changes in magnesium to effect sensitive control of magnesium balance.

Physiology of Renal Magnesium Handling
Glomerular Filtration
Approximately 70% of plasma magnesium is in the ionic form, Mg 2+ . The remaining magnesium is bound to circulating proteins (essentially albumin) or is complexed with citrate, oxalate, and phosphate anions. About 80% of the total serum magnesium is ultrafilterable through the glomerular membrane that varies with the amount bound to nonfilterable proteins.
Proximal Tubule
Physiology of Proximal Tubule Magnesium Reabsorption
In the adult, proximal tubule magnesium reabsorption rate (15–20%) is considerably less than the fractional reabsorption of sodium and calcium. In neonates, the proximal tubule reabsorbs about 70% of the filtered magnesium that is the same as the fractional reabsorption of sodium and calcium. Clearly, the permeability of the proximal tubule changes during development so that more magnesium is delivered to the loop of Henle in the adult. This maturation in segmental handling of magnesium should be taken into consideration when assessing renal magnesium handling in very young children. Proximal tubule magnesium transport in adults is thought to be active and transcellular in nature, whereas it is probably passive in the immature individual occurring through the paracellular pathway entrained with water movement.
Various hormones alter magnesium reabsorption within the proximal tubule. They do so by influencing salt and water transport, and thus luminal magnesium concentration. Reabsorption is load dependent, so that transport is greater with elevated luminal magnesium concentrations. Extracellular volume expansion or anything that retards salt and water transport results in diminished fluid absorption and greater magnesium delivery into the loop of Henle and the DCT. The increase in distal delivery is normally reclaimed in these nephron segments but may be large enough to cause an increase in urinary magnesium excretion and hypermagnesiuria.
Loop of Henle
Physiology of Loop of Henle Magnesium Reabsorption
The thin descending limb is able to reclaim significant amounts of filtered magnesium. Inference from micropuncture data of the differences in magnesium delivery to the late proximal tubule and the hairpin turn of rat juxtamedullary nephrons indicate that a significant fraction of the filtered magnesium, on the order of 30%, is reclaimed along the descending limb in the concentrating kidney. The amount of magnesium absorption is associated with water transport in the descending limb. Accordingly, magnesium reabsorption is considerably diminished during forced diuresis when water reabsorption is negligible in this segment. The TAL reabsorbs the predominant portion of the magnesium, amounting to some 70% of the filtered load. Moreover, de Rouffignac and colleagues have shown that it is the cortical thick ascending limb (cTAL), not the medullary segment (mTAL), that reabsorbs magnesium. They have further reported that transepithelial magnesium absorption is passive moving from lumen to the interstitial space through the paracellular pathway ( Fig 61.2 ), consistent with earlier observations. The driving force for magnesium movement is the positive luminal transepithelial voltage generated by K + recycling across the apical membrane ( Fig 61.2 ). Any influence that alters transepithelial voltage or the permeability of the paracellular pathway will alter magnesium reabsorption in the cTAL. The voltage in the TAL is dependent on apical 70-pS K + channel (ROMK) activity and Na–K–2Cl cotransport that control current flow across the apical membrane of the TAL cell. Sodium exits by the basolateral Na–K–ATPase and chloride through basolateral ClC-Ka and ClC-Kb members of the chloride channel family (ClC). Functional expression of ClC-Ka and ClC-Kb channels requires the coexpression of barttin, an essential β-subunit of the channels. As barttin controls ClC-Ka and ClC-Kb activity, it importantly influences salt absorption in the TAL. Changes in their transport rates will affect the transepithelial voltage and thus the magnesium absorption. The permeability of the paracellular pathway also plays an important role in determining transepithelial magnesium transport. Paracellular Mg 2+ movement is influenced by electrostatic charges of proteins comprising this route. Moreover, there appears to be selectivity of the pathway to divalent cations. Paracellular proteins of the claudin family of tight junction proteins claudin-16 and claudin-19 have been identified in the TAL and are involved in controlling magnesium and calcium permeability of the paracellular pathway. This notion is supported by the phenotype resulting from mutations in the encoding genes CLDN16 and CLDN19 . Affected individuals present with massive calcium and magnesium wasting due to defective reabsorption in the cTAL.

In humans, the claudin family comprises at least 22 proteins with a molecular weight between 20 and 27 kDa. They consist of four transmembrane helices, two extracellular loops, and intracellular N- and C-termini. While the first loop is believed to influence paracellular charge selectivity, the second is probably responsible for the interaction between opposing claudins of adjacent cells. In association with occludin, claudins polymerize into linear fibrils that ultimately alter ion selectivity and permeability of tight junctions. Claudins have been shown to be differentially expressed along the nephron and to be regulated by receptor-mediated activation.
Control of Loop of Henle Magnesium Reabsorption
Hormonal Controls
A large number of hormones stimulate magnesium reabsorption in the loop of Henle ( Table 61.1 ). Included are peptide hormones such as parathyroid hormone (PTH), calcitonin, glucagon, and arginine vasopressin (AVP). Adrenergic agonists, isoproterenol, and insulin also increase magnesium transport. Mineralocorticoids stimulate NaCl absorption and in turn transepithelial voltage in the loop and by inference increase magnesium reclamation in this segment. Prostaglandins, on the other hand, diminish both salt and magnesium conservation in the cTAL. All of these hormonal responses are mediated by changes in transepithelial voltage by altering apical K + recycling and Na–K–2Cl cotransport and the paracellular permeability by modifying tight junctions proteins.
TAL | DCT | |
---|---|---|
Peptide hormones (PTH, Calcitonin, Glucagon, Vasopressin, Insulin) | ↑ | ↑ |
Prostaglandins (PGE 2 ) | ↓ | ↑ |
Aldosterone | ↑ | ↑ |
1,25-(OH) 2 -vitamin D 3 | ? | ↑ |
Magnesium restriction | ↑ | ↑ |
Hypermagnesemia | ↓ | ↓ |
Hypercalcemia | ↓ | ↓ |
Extracellular volume expansion | ↓ | ↑ |
Metabolic acidosis | ↓ | ↓ |
Metabolic alkalosis | ↑ | ↑ |
Phosphate depletion | ↓ | ↓ |
Potassium depletion | ↓ | ↓ |
Nonhormonal Controls
The extracellular Ca 2+ -sensing receptor (CaSR) importantly influences divalent cation transport in the TAL. Activation of the CaSR decreases hormone-dependent cAMP release by inhibition of adenylyl cyclase. CaSR activation has been reported to reduce the activity of the 70-pS K + channel, and thus potassium recycling and Na–K–2Cl cotransport leading to diminished transepithelial voltage. Though putatively acting through this mechanism, it does not affect salt reabsorption much. A number of studies have shown that elevated calcium and magnesium, as well as the multivalent gadolinium and the calcimimetic NPS R-467, inhibit the hormonal stimulation of intercellular calcium and magnesium movement at any given voltage, suggesting that the CaSR may also have an effect on paracellular pathways, again perhaps acting through the modification of tight junctions. CaSRs are expressed at the basolateral membrane of both medullary and cortical TALs so that they respond to increases in divalent cation concentrations in the blood/interstitium rather than luminal Ca 2+ and Mg 2+ . This explains earlier findings that increases in plasma calcium or magnesium inhibit calcium and magnesium transport but increases in luminal concentrations do not.
Hypermagnesemia and hypercalcemia have long been known to result in diminished divalent cation reabsorption. Using clearance studies, Wong et al. elevated serum magnesium levels in thyroparathyroidectomized dogs and determined the urinary magnesium excretion rates with elevated filtered magnesium. They showed that elevated filtered magnesium concentration was initially associated with increases in magnesium reabsorption until an apparent tubular maximum, Tm, was attained, beyond which any additional filtered magnesium was excreted in the urine. Micropuncture studies showed that this Tm was the result of increased proximal tubule reabsorption and diminished loop transport, and has been extensively used in the clinical assessment of renal magnesium conservation. The cellular basis for these observations, as revealed by Hebert and colleagues, is the CaSR present at the basolateral membrane of the TAL that inhibits transport upon elevation of either calcium or magnesium. The concept of a Tm as an index of total renal magnesium conservation is still useful as long as the segmental contribution is considered and the plasma magnesium concentration is not extreme.
Dietary magnesium restriction and hypomagnesemia have been reported to stimulate magnesium absorption within the cTAL. Wittner et al. have shown that the increase in magnesium transport is due to an increase in the permeability of the paracellular pathway to magnesium, in keeping with the passive nature of magnesium transport within the loop. Interestingly, calcium absorption in the loop is also increased by magnesium restriction, so the response is not selective to magnesium. Again, we assume that these changes in paracellular magnesium transport involve modification of tight junction proteins including claudin-16 and claudin-19. In support of this notion, Efrati et al. have reported that the amount of cldn16 transcript is increased in mice with dietary-induced hypomagnesemia.
Loop Diuretics
Furosemide and bumetanide diminish the luminal positive voltage by virtue of their effects on the Na–K–2Cl cotransporter. Because of this, the acute furosemide usage leads to enhanced distal delivery and increased urinary excretion of magnesium. However, with chronic furosemide therapy urinary excretion usually returns to near control levels. This is likely due to enhanced reabsorption in the proximal tubule and DCT. Thus, prolonged use of furosemide does not often lead to renal magnesium wasting.
Distal Convoluted Tubule
Physiology of Distal Convoluted Tubule Magnesium Reabsorption
The DCT plays an essential role in determining the final urinary magnesium excretion since little or no magnesium is reabsorbed beyond this segment. Magnesium transport within the DCT is transcellular and active in nature ( Fig 61.3 ). Magnesium enters the cell through selective ion channels across the apical membrane, driven by the transmembrane negative electrical potential. Apical magnesium entry is the rate-limiting step in reabsorption, and many of the hormonal and nonhormonal controls act at this site. Cellular magnesium is actively extruded at the basolateral membrane, possibly by a sodium-dependent exchange mechanism which is still unresolved at the molecular level. Micropuncture studies and experiments with immortalized mouse DCT (mDCT) cells have shown that magnesium transport in the DCT is dependent on luminal magnesium concentration and apical transmembrane voltage.

Though being far from completely understood, our knowledge of magnesium transport in the DCT has greatly evolved over the recent years. First, the divalent cation channel, TRPM6, had been implicated in renal magnesium absorption based on the observation that mutations in the TRPM6 gene cause autosomal recessive hypomagnesemia with secondary hypocalcemia (HSH). HSH is characterized by very low serum magnesium due to diminished intestinal magnesium absorption and renal magnesium wasting (see section IIA6). TRPM6 is a member of the transient receptor potential melastatin (TRPM) family of cation channels. They are characterized by six transmembrane segments, a highly conserved pore-forming region, and a Pro-Pro-Pro motif following the last transmembrane domain. Unlike the other members of the TRPM family, TRPM6 and its close homologue TRPM7 possess a unique structure in that they have an alpha-kinase domain in the C-terminal region. Dorovkov and Ryazanov have shown that this alpha-kinase phosphorylates and activates annexin 1. Annexin 1 plays a pleiotropic role in cell proliferation, inflammation, and apoptosis. What this has to do with magnesium transport is not known but Nadler et al. have reported that TRPM7 is essential in cell viability so that it likely plays an important role in cell metabolism. Takezawa et al. have provided evidence that TRPM7 activity is modulated by its own endogenous kinase domain, responding to changes in intracellular levels of cAMP induced by G i – and G s -coupled receptors. Earlier it was found that TRPM6 and TRPM7 channels are permeable to calcium and magnesium, are inhibited by increases in intracellular magnesium levels or Mg-ATP, and, although controversial, possibly inactivated by phosphatidylinositol 4,5-bisphosphate hydrolysis. TRPM7 is ubiquitously expressed among tissues whereas TRPM6 expression is restricted to few tissues including intestine and kidney. Chubanov et al. have shown that TRPM6 and TRPM7 form heteromultimeric complexes that form functional magnesium permeable channels at the cell surface. Voets et al. have reported that TRPM6 is located along the apical membrane of the small intestine and DCT, transports both magnesium and calcium, and is associated with the divalent cation-binding proteins, parvalbumin, and calbindin-D 28K . Whether these binding proteins play a role in regulation of cellular magnesium reabsorption, as they do for calcium, is not known. Several authors have also shown that other divalent ions, such as zinc and cobalt, were also transported by TRPM6, which supports earlier observations demonstrating that TRPM6/TRPM7 are permissive metal ion transporters. Nevertheless, magnesium appears to be the preferred substrate so that TRPM6 and TRPM7 are considered as a primary magnesium entry channel.
Control of Distal Convoluted Tubule Magnesium Reabsorption
Hormonal Controls
A large number of peptide hormones stimulate magnesium absorption within the DCT ( Table 61.1 ). Many of these are the same hormones that influence magnesium transport in the cTAL of Henle’s loop. These include PTH, calcitonin, glucagon, and AVP, all of which act through individual receptor-mediated signaling (reviewed in Dai et al. ). Renal magnesium conservation seems to be a concerted response to all of these hormones. However, these hormones also stimulate sodium, potassium, and chloride transport in the mTAL, cTAL, and calcium transport in both the cTAL and the distal tubule so that hormonal control is not specific to magnesium.
There is good evidence that vitamin D is important for renal magnesium conservation. Ritchie et al. have demonstrated that 1,25(OH) 2 D 3 increases magnesium entry rates in isolated DCT cells. The response is concentration dependent, involves transcriptional processes necessitating de novo protein synthesis, and does not appear to be related to receptor-mediated cAMP release and stimulation of magnesium uptake. Finally, 1,25(OH) 2 D 3 -stimulated magnesium transport is additive to PTH-mediated uptake suggesting that the peptide and steroid hormones regulate magnesium absorption through distinctive intracellular signaling pathways. These studies show that vitamin D 3 metabolites modulate magnesium transport in the DCT independent of other hormonal influences.
Vitamin D 3 administration has often been associated with increases in urinary magnesium and calcium excretion. Vitamin D 3 metabolites increase intestinal absorption of magnesium and calcium so that a positive divalent cation balance may lead to hypermagnesemia and hypercalcemia which in turn diminish magnesium absorption in the loop of Henle through the extracellular CaSR. This may also explain the increase or absence of changes in urinary magnesium excretion following administration of vitamin D 3 . The net effect on magnesium balance would thus depend on the relative magnitude of vitamin D 3 actions at the intestinal and renal levels.
Aldosterone enhances hormone-stimulated magnesium entry by potentiating receptor-mediated intracellular signaling, including cAMP formation. Accordingly, acute mineralocorticoids increase magnesium conservation within the DCT in the presence of circulating hormones. Chronic aldosterone administration, on the other hand, generally results in renal magnesium wasting. This has been explained by extracellular volume expansion leading to diminished salt and magnesium reabsorption within the loop of Henle. The potentiation of hormone-mediated magnesium reabsorption in the distal convolute would tend to minimize magnesium loss in hypermineralocorticoidism
Other humoral factors influence renal magnesium conservation such as prostaglandins, insulin, adrenergic agonists, and purinogenic metabolites. Prostaglandin E 2 (PGE 2 ) is the major arachidonate metabolite synthesized by cyclooxygenase in the mammalian kidney. PGE 2 has a number of diverse actions on the kidney in addition to its ability to influence renal hemodynamics. PGE 2 inhibits salt transport in the mTAL and salt as well as calcium and magnesium absorption within the cTAL and also modulates sodium and water transport in the cortical collecting duct (CCD). By its actions in the DCT, prostaglandins may limit magnesium excretion and maintain magnesium balance. Insulin has clearly been shown to have antinatriuretic and antimagnesiuric effects by its actions on the TAL. but insulin also increases magnesium transport in isolated DCT cells. Furthermore, the nephron is richly innervated along its length from the glomerulus to the collecting tubule. Renal nerve stimulation significantly increases salt and water reabsorption in the proximal tubule, loop of Henle, and distal tubule. Dai and colleagues have shown that isoproterenol increases magnesium transport in DCT cells. It can be concluded from these studies with immortalized mDCT cells that renal nerves and circulating catecholamines play a role in the control of magnesium transport in the DCT.
The interactions of the various peptide and steroid hormones, prostaglandins, and renal innervation are complex. It can be inferred that overall distal magnesium absorption is controlled by all of these influences initiated individually but coming together through shared intracellular signaling pathways. Few studies have been directed at describing these interactions. It is clear that control of renal magnesium handling is orchestrated by many hormones and influences.
The most interesting discovery concerning the hormonal control of tubular magnesium reabsorption stems from the discovery of a mutation in pro-EGF in a family with hereditary magnesium wasting (see below). The mutation impairs the basolateral sorting of epidermal growth factor (EGF) in the DCT. Thereby, binding of EGF to basolaterally expressed EGF receptor (EGFR) is abolished which finally leads to renal magnesium wasting. The supposed mechanism of EGF action is a stimulation of intracellular Src kinase as well as MAP kinase signaling cascades which in turn activate magnesium reabsorption via TRPM6. Thebault and colleagues could show that incubation with EGF leads to an activation of TRPM6-induced currents in HEK293 cells. The authors suggest EGF to be the first selectively acting magnesiotropic hormone as no influence on calcium transport was detected. However, EGF is also known to influence sodium reabsorption in the aldosterone sensitive distal nephron by activation of MAP kinase signaling.
Nonhormonal Controls
Early in vivo microperfusion studies have demonstrated that elevated extracellular magnesium or calcium also inhibit fractional magnesium transport in superficial rat distal tubules. This has been attributed to the actions of the extracellular CaSR. Riccardi et al. have shown that the CaSR is located on the basolateral membrane of the DCT. Bapty et al. reported that high extracellular magnesium or calcium concentrations inhibit hormone-stimulated magnesium uptake in immortalized mDCT cells. These studies clearly indicate that hypermagnesemia and hypercalcemia per se can modify hormone regulation of active, transcellular magnesium transport within the DCT leading to increased magnesium excretion.
The CaSR in the loop of Henle and also in the DCT provides a negative feedback mechanism to mitigate the over exuberant responses of PTH. PTH stimulates calcium and magnesium reabsorption within both nephron segments leading to a rise in serum divalent cation concentrations. Increments in serum calcium and magnesium feedback at the parathyroid gland to inhibit secretion of PTH and at the kidney tubule to inhibit calcium and magnesium transport in the cTAL and the DCT ( Fig 61.4 ). Both extrarenal and intrarenal feedback mechanisms involve the same CaSR located in the parathyroid gland and on renal epithelial cells, respectively. There is evidence that the scenario for PTH feedback may also apply to other calcium- and magnesium-conserving hormones such as vitamin D. Vitamin D metabolites enhance magnesium uptake in isolated DCT cells through a genomic mechanism involving transcriptional/translational processes requiring 2–3 hours following the addition of the hormone. Elevation of extracellular calcium abolishes 1,25(OH) 2 D 3 stimulation indicating that the CaSR may modify gene expression in DCT cells. In order to determine whether elevated extracellular calcium inhibits 1,25(OH) 2 D 3 responses through the CaSR, Dai and coworkers preincubated the cells with a specific antibody to the CaSR protein before treating them with high calcium and 1,25(OH) 2 D 3 . The antibody prevented the effect of calcium on 1,25(OH) 2 D 3 -induced magnesium uptake, clearly demonstrating a receptor-mediated response. Accordingly, excess 1,25(OH) 2 D 3 increases distal calcium and magnesium transport leading to elevated serum concentrations and activation of the CaSR that provides a negative feedback on divalent cation reabsorption. The CaSR plays an important additional role in mineral metabolism: The CaSR also inhibits water reabsorption in the collecting duct so that excess luminal calcium does not accumulate to form calcareous concretions. There, the CaSR is expressed both at the luminal and basolateral membrane. Therefore, it not only senses interstitial divalent cation concentrations but also intraluminal or urinary cation levels. CaSR activation results in a reduction of autosomal-dominant hypoparathyroidism (ADH)-mediated Aquaporin-2 incorporation into the apical membrane and consecutively a reduced water permeability of the inner medullary collecting duct (IMCD) (citation). The net effect is a concomitant increase in volume flow along with a rise in calcium and magnesium excretion which minimizes the risk of stone formation.

Distal Diuretics
The distal diuretics, hydrochlorothiazide, and amiloride increase magnesium uptake in DCT cells. These agents inhibit sodium chloride cotransport and sodium channels (ENaC), respectively, leading to diminished intracellular chloride concentration and hyperpolarization of the apical membrane. The increased apical membrane voltage stimulates magnesium entry and transepithelial transport. Although amiloride has clearly been shown to be a magnesium-sparing diuretic, chronic hydrochlorothiazide usage may lead to renal magnesium wasting. The cellular mechanisms for the chronic hydrochlorothiazide effects are unclear but may involve hypokalemia, which can jeopardize renal magnesium conservation.
Overall Regulation of Renal Magnesium Handling
In addition to hormonal controls and CaSR-mediated regulation of magnesium reabsorption in TAL and DCT, magnesium transport in these parts of the nephron is regulated in response to altered magnesium status ( Table 61.1 ). A reduction in serum magnesium levels directly results in a decrease in filtered load and consecutively a diminished magnesium flow through the distal nephron. To elucidate the response of the distal nephron to changes in magnesium status, different techniques have been applied including (1) animal studies using magnesium restricted diets and inbred strains with high or low magnesium status, (2) micropuncture and microperfusion studies, and (3) in-vitro experiments with distal tubular cell lines. The discovery of new genes and their encoded proteins involved in paracellular and transcellular magnesium transport in TAL and DCT, respectively, now enables us to investigate the adaptive changes at the molecular level. Classic microperfusion studies in microdissected nephron segments had indicated an increased fractional reabsorption of magnesium in the TAL in rats maintained on a low magnesium diet for only 6 hours, when there is a maximum decline in renal magnesium excretion, but serum magnesium levels are still normal. Calcium and sodium reabsorption rates during this adaptive response remained unchanged. An analogue response was also described for the DCT and furthermore demonstrated in isolated mDCT cells. An inhibition of this adaptive process by pretreatment of cells with cycloheximide, an inhibitor of protein synthesis, implicated genetically controlled mechanisms. More recent studies now identified putative underlying mechanisms of changes in renal tubular magnesium handling in response to magnesium restriction or excess. Efrati and colleagues demonstrated changes in claudin-16 expression in the TAL in mice fed diets with differing magnesium content. Two further studies in mice fed magnesium restricted diets could show that one mechanism underlying the upregulation of renal magnesium conservation in the DCT is an increased expression of TRPM6. Contrariwise, Groenestege and colleagues demonstrated that a magnesium enriched diet results in an increase in urinary magnesium, but also calcium wasting. They also found that TRPM6 expression was not dependent on 1,25(OH) 2 -vitamin D or PTH but upregulated by estrogens.
Similarly, hormonal responses are serially organized to regulate magnesium transport in both TAL and DCT. This may be beneficial in that perturbing influences acting in either the loop or distal tubule that can be balanced by homeostatic responses in the other segment. The antenatal Bartter syndrome (aBS) typifies this serial control in magnesium conservation. It results from mutational changes in sodium chloride cotransport in the TAL leading to defective salt absorption ( Fig 61.2 ). Patients with this condition are characterized by volume depletion, hyperreninemia, secondary hyperaldosteronism, hypokalemia, and metabolic alkalosis (see below). Despite defective TAL function, few of these patients demonstrate hypomagnesemia, presumably due to increased reclamation of magnesium in the DCT not affected by the TAL defect. In this case, DCT function compensates for diminished TAL reabsorption even though the hormonal controls are similar.
Pathophysiology of Renal Magnesium Handling
Genetic Magnesium-Wasting Diseases
Hereditary hypomagnesemia comprises a set of rare genetically determined disorders primarily or secondarily affecting renal magnesium handling. Over the past years, numerous genetic defects in genes encoding components of the renal tubular salt and electrolyte transport machinery or regulating factors have been described ( Table 61.2 ). The spectrum ranges from the most frequent variant, the Gitelman syndrome (GS) with a primary defect in salt reabsorption in the DCT to rare disorders discovered in single patients or families only. The following sections try to summarize essential data from a multitude of research studies published over the last years. Defects primarily or solely affecting renal magnesium handling are preceded by a paragraph on renal salt wasting disorders (Bartter syndrome).
Disorder | OMIM | Gene | Protein | S-Mg 2+ | S-Ca 2+ | U-Mg 2+ | U-Ca 2+ | Nephrocalcinosis | Additional Findings |
---|---|---|---|---|---|---|---|---|---|
Antenatal Bartter syndrome (aBS) | 601678 241200 | SLC12A1 KCNJ1 | NKCC2 ROMK (Kir1.1) | N | N | ? | ↑↑ | Yes | Polyhydramnios, Salt wasting, Hypokalemic alkalosis |
Bartter syndrome with sensorineural deafness (BSND) | 602522 613090 | BSND CLCNKA+CLCNKB | Barttin ClC-Ka+ClC-Kb | ↓ | N | ? | N to ↑ | No | Polyhydramnios, Salt wasting, Hypokalemic alkalosis, Deafness |
Classic bartter syndrome (cBS) | 607364 | CLCNKB | ClC-Kb | N to ↓ | N | N to ↑ | variable | rare | Failure to thrive, Salt wasting, Hypokalemic alkalosis |
Gitelman syndrome (GS) | 263800 | SLC12A3 | NCCT | ↓ | N | ↑ | ↓ | No | Mild salt wasting, Hypokalemic alkalosis, Chondrocalcinosis |
EAST/SeSAME syndrome | 612780 | KCNJ10 | Kir4.1 | ↓ | N | ↑ | ↓ | No | Salt wasting, Epilepsy, Ataxia, Mental retardation, Deafness |
Autosomal-dominant hypocalcemia (ADH) | 146200 | CASR | CaSR | ↓ | ↓ | ↑ | ↑ to ↑↑ | Yes | (Salt wasting, Hypokalemic alkalosis) |
Familial hypocalciuric hypercalcemia (FHH) | 145980 | CASR | CaSR | N | ↑ | ↓ | ↓ | No | |
Neonatal severe hyperparathyroidism (NSHPT) | 239200 | CASR | CaSR | N to ↑ | ↑↑↑ | ↓ | ↓ | No | Skeletal deformities, Extraosseous calcifications, Muscle wasting, Mental retardation |
Familial hypomagnesemia with hypercalciuria and nephroclacinosis (FHHNC) | 248250 248190 | CLDN16 CLDN19 | Claudin-16 Claudin-19 | ↓ | N | ↑↑ | ↑↑ | Yes | Urinary tract infections, Polyuria/polydipsia, Nephrolithiasis, Chronic renal failure, (eye abnormalities) |
Hypomagnesemia with secondary hypocalcemia (HSH) | 602014 | TRPM6 | TRPM6 | ↓↓↓ | ↓ | ↑ | N | No | Defect in intestinal magnesium uptake |
Isolated dominant hypomagnesemia (IDH) | 154020 | FXYD2 | γ-subunit | ↓ | N | ↑ | ↓ | No | |
Isolated dominant hypomagnesemia (IDH) | 160120 | KCNA1 | Kv1.1 | ↓↓ | N | ↑ | N | No | Episodic ataxia, Myokymia |
Isolated dominant hypomagnesemia (IDH) | 613882 | CNNM2 | cyclin M2 | ↓ | N | ↑ | N to ↓ | No | |
Isolated recessive hypomagnesemia (IRH) | 611718 | EGF | EGF | ↓ | N | ↑ | N | No | |
Mitochondrial hypomagnesemia (MH) | 500005 | MTTI | tRNA-ILE | ↓ | N | ↑ | ↓ | No | Hypercholesterolemia, Hypertension |
Renal Cysts and Diabetes Syndrome (RCAD) | 137920 | HNF1B | HNF1β | ↓ | N | ↑ | ↓ | No | Diabetes (MODY5), Renal dysplasia |
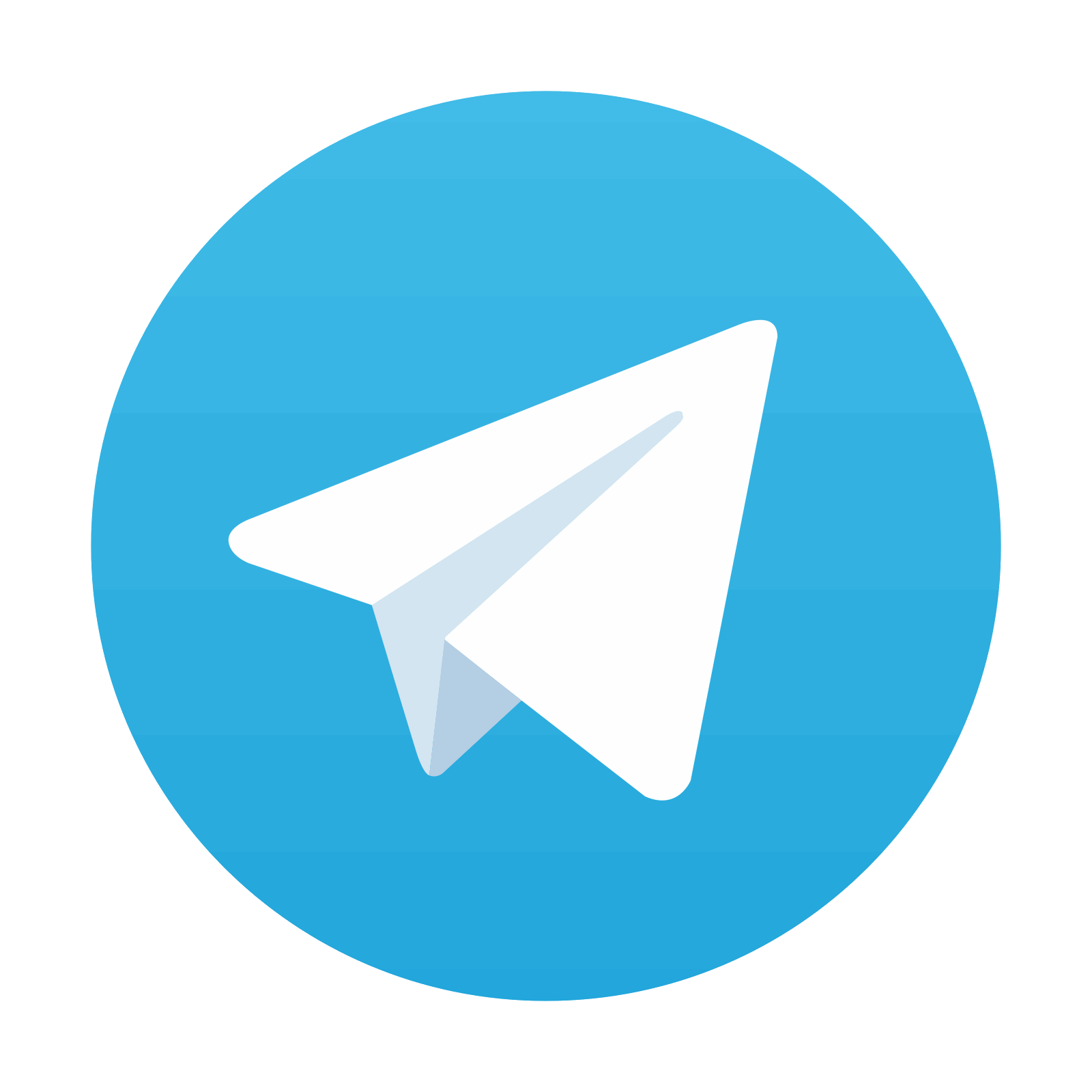
Stay updated, free articles. Join our Telegram channel

Full access? Get Clinical Tree
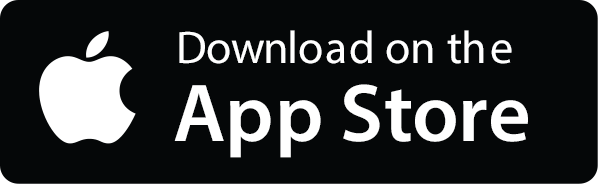
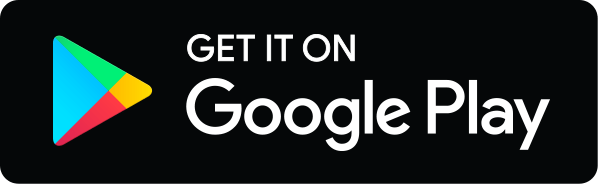
