The intrinsic ability of the kidney to regulate renal blood flow and glomerular filtration rate (GFR) over a wide range of perfusion pressures has fascinated physiologists for decades. These adaptive responses to physiologic and pathologic alterations in renal perfusion, known collectively as renal autoregulation, serve to maintain renal excretory function in settings of decreased renal blood flow, such as in states of volume depletion, hypotension, or reduced cardiac output. Another important and unique setting of renal hypoperfusion is that of renal arterial occlusive disease due to a fixed stenosis, a situation that typically presents with a chronic, gradual, and eventually critical reduction of perfusion. This physiologic stress depends on renal autoregulation to maintain GFR, but the ongoing global renal hypoperfusion appears to eventually produce chronic ischemic injury to the renal parenchyma through mechanisms that are not clearly understood. This latter prospect is clearly a threat to the viability of the kidney and appears to be an important cause of chronic and often irreversible kidney disease. An understanding of the limits, mechanisms, and the pharmacologic manipulation of renal autoregulation is important in this setting because the disordered physiology is silent. Likewise, an understanding of the pathophysiology of chronic ischemic injury is important in designing effective clinical strategies to treat this disorder.
This chapter will focus on the clinically important issue of atherosclerotic renal artery stenosis (RAS) as a cause of ischemic renal disease, also known as ischemic nephropathy (IN). This disorder is recognized more frequently as a cause of end-stage renal disease. The patient population most likely to benefit from revascularization procedures is, however, not clearly defined. However, recently completed clinical trials, in conjunction with ongoing studies, are likely to bring clarity to this clinical conundrum. This chapter will review the clinical presentations of ischemic renal disease, the modalities available for diagnosis, the pathophysiology of renal hypoperfusion and ischemic renal injury, and provide insights into treatment.
Key Words
Renal Artery, Renal Artery Obstruction, Renal Artery Stenosis
The intrinsic ability of the kidney to regulate renal blood flow and glomerular filtration rate (GFR) over a wide range of perfusion pressures has fascinated physiologists for decades. These adaptive responses to physiologic and pathologic alterations in renal perfusion, known collectively as renal autoregulation, serve to maintain renal excretory function in settings of decreased renal blood flow, such as in states of volume depletion, hypotension, or reduced cardiac output. Another important and unique setting of renal hypoperfusion is that of renal arterial occlusive disease due to a fixed stenosis, a situation that typically presents with a chronic, gradual, and eventually critical reduction of perfusion. This physiologic stress depends on renal autoregulation to maintain GFR, but the ongoing global renal hypoperfusion appears to eventually produce chronic ischemic injury to the renal parenchyma through mechanisms that are not clearly understood. This latter prospect is clearly a threat to the viability of the kidney and appears to be an important cause of chronic and often irreversible kidney disease. An understanding of the limits, mechanisms, and the pharmacologic manipulation of renal autoregulation is important in this setting because the disordered physiology is silent. Likewise, an understanding of the pathophysiology of chronic ischemic injury is important in designing effective clinical strategies to treat this disorder.
This chapter will focus on the clinically important issue of atherosclerotic renal artery stenosis (RAS) as a cause of ischemic renal disease, also known as ischemic nephropathy (IN). This disorder is recognized more frequently as a cause of end-stage renal disease. The patient population most likely to benefit from revascularization procedures is, however, not clearly defined. However, recently completed clinical trials, in conjunction with ongoing studies, are likely to bring clarity to this clinical conundrum. This chapter will review the clinical presentations of ischemic renal disease, the modalities available for diagnosis, the pathophysiology of renal hypoperfusion and ischemic renal injury, and provide insights into treatment.
Atherosclerotic Renal Artery Stenosis: A Common Disorder With Increasing Clinical Recognition and Public Health Importance
Over the past few decades, the lesions of renal artery stenosis have been more frequently diagnosed in atherosclerotic patients with hypertension or deteriorating renal function. In addition to an increased awareness of the disorder among clinicians, the proliferation of noninvasive diagnostic techniques such as magnetic resonance angiography, computed tomography (CT) angiography, and duplex sonography has fueled this trend. Among the estimated 60 million Americans with hypertension, the prevalence of significant RAS in this population has been estimated at 2 to 5%. Autopsy series done in the 1960s indicate that RAS is common in the elderly. An autopsy study by Holley et al. demonstrated a 27% prevalence of 50% stenoses in patients with a mean age of 64 years, however, the prevalence increased to 62% in individuals greater than 70 years old. Alternatively, a study by Schwartz and White demonstrated a lower prevalence of stenoses ≥50%: 5% if younger than 65 years, 18% in 65- to 74-year-olds, and 42% if 75 years and older. Kuroda et al. found atherosclerotic RAS of at least 75% luminal area narrowing in 10% of autopsies of 60-year-old Japanese individuals with a history of stroke, and a 24% prevalence in individuals with carotid stenosis. Importantly, it is likely that these autopsy series over-estimate the rate since Edwards et al. have described a prevalence of 6.8% in a prospective U.S. cohort of adults >65 years old when a liberal Duplex velocity threshold of 1.8 meters/second was used during community screening. In aggregate these studies suggest that renal stenoses are rather common, and that the prevalence rises rather dramatically in the elderly and it is undoubtedly true that many of the “50%” stenoses documented at autopsy were hemodyamically irrelevant. This latter point is of importance as more patients are now identified with non-invasive imaging.
As would be expected given the generally diffuse involvement of atherosclerosis, the prevalence of RAS is high when renal angiography is performed on patients who are undergoing angiography for other indications. When performed in the evaluation for aortic disease, coronary artery disease, or peripheral arterial occlusive disease, the prevalence has ranged from 11 to 42%. The highest prevalence seems to be in patients with peripheral vascular and aortic disease.
The public health importance of kidney disease due to RAS can be gauged by the number of individuals who progress to end-stage renal disease (ESRD) as a result of this disorder. The true prevalence in this population has been difficult to accurately estimate. It is widely believed that clinical diagnosis of IN is often missed and that many individuals who have been labeled as having hypertensive nephrosclerosis as a primary cause of ESRD may actually have suffered IN. On the other hand, the mere presence of RAS in an individual with chronic kidney disease does not prove IN as its etiology. Using the U.S. Renal Data System database, Fatica et al. reviewed the primary causes of ESRD in patients starting ESRD therapy during 1991 to 1997, and they found that the incidence of renovascular disease as a primary cause of ESRD increased from 1.4% of new ESRD cases to 2.1%. The annualized increase was 12.4% per year. This is a greater rate of increase than for ESRD from diabetes mellitus (8.3% per year) and ESRD overall (5.4% per year).
In a report from England, Scoble and colleagues prospectively performed renal arteriography in all new patients with ESRD during an 18-month period in the late 1980s. Atherosclerotic renal artery disease was ultimately believed to be the cause of ESRD in 6% of all patients and in 14% of patients older than 50 years. Appel and coworkers suggest that renovascular disease accounts for 11% of all patients with ESRD older than 50 years and 20% of ESRD cases among whites older than 50 years. Mailloux et al. performed an analysis of patients entering their ESRD program between 1982 and 1985 for possible ischemic nephropathy and estimated a prevalence of 16%. This represented a significant increase from a prevalence of 8% reported among those who entered their program between 1970 and 1982. This was thought to most likely be a reflection of increased awareness of the disease and the rising age of the dialysis population.
Further supporting the public health importance of IN is the clear evidence that this diagnosis imparts a high mortality risk and that patients with ESRD due to IN do poorly on dialysis. Given the frequent coexistent atherosclerotic coronary artery disease in these patients, cardiovascular deaths are common. In a Swedish study of a cohort of patients with RAS lesions exceeding 50%, Johansson and colleagues found a risk ratio (versus age-matched controls) was 3.3 for overall mortality and 5.7 for cardiovascular mortality. Baboolal et al. published a report in 1998 describing the poor outcome of medically treated patients with severe bilateral RAS: such patients had a crude mortality rate at 60 months of 45%. In an analysis of survival of patients who started dialysis from 1970 to 1985 by Mailloux et al., patients with renovascular disease as the cause of ESRD had very poor survival, a mean survival of 27-months and a 12% 5-year survival rate.
Over the past few decades intraluminal renal artery revascularization has enjoyed tremendous technologic advances and has become a very commonly performed procedure. This is likely in response to the increased awareness of the diagnosis and in hopes of favorably altering its poor prognosis. Among the estimated two to four million cases of renal artery stenosis in the United States, approximately 7000 such procedures are done annually, at an estimated cost of $1.7 billion per year. It remains unclear if the rate of utilization is appropriate, and some experts have suggested that this rate is far too low while others have suggested that it is far too high. Unfortunately, the current body of literature in this area supports only opinion-based rather than firm evidence-based recommendations. Although there is controversy surrounding the management of this disorder and conflicting recommendations from experts regarding treatment, the prevalence of the disorder mandates that clinicians know how to efficiently diagnose the disorder and be familiar with the current understanding of the pathophysiology and natural history of IN.
Clinical Presentations of Ischemic Nephropathy
IN can present as one of several clinical manifestations. Mild to moderate kidney disease in a patient with known renovascular disease should raise suspicion for the presence of ischemic renal disease. These patients tend to be older, have a shorter duration of hypertension, require more medications for blood pressure (BP) control, show other features of diffuse vascular disease, and have a higher incidence of hypertensive retinopathy and vascular bruits than patients with essential hypertension. These patients may develop a progressive decrease in renal size by sonography along with a rising serum creatinine concentration. Ischemic renal disease should also be considered in any elderly patient who presents with unexplained acute or chronic kidney disease, especially if there is evidence of generalized atherosclerosis, even in the absence of hypertension. Development of hypertension later in life (after age 60) or an abrupt loss of blood pressure control in previously well-controlled essential hypertension, with evidence of a decline in renal function, should also raise the suspicion of this entity. Bilateral renovascular disease may be suspected from the occurrence of acute kidney injury following the initiation or intensification of antihypertensive therapy, especially with an angiotensin-converting enzyme (ACE) inhibitor or angiotensin receptor blocker. Nevertheless, the absence of ARF in this setting does not rule out the possibility of ischemic renal disease. A similar reduction in GFR may occur in the setting of intravascular volume depletion, congestive heart failure (CHF), and use of other antihypertensive agents such as vasodilators. A clinical scenario in which the diagnosis of renal artery disease is often suspected is in patients with poorly controlled hypertension, renal insufficiency, and left ventricular hypertrophy who develop acute pulmonary edema rapidly, so-called “flash pulmonary edema.” Finally, unstable angina with poorly controlled hypertension may be a presenting complaint, especially when the degree of coronary stenosis seems proportionally less than the severity of the presentation.
The character of the renal disease is nonspecific, with progressive azotemia with mild-to-moderate proteinuria (rarely nephrotic) and a bland urinary sediment. There have been reports of nephrotic-range proteinuria that has been attributed to the development of focal glomerulosclerosis in the kidney contralateral to a unilateral RAS lesion. Rarely the disease may present with acute oligoanuric kidney disease due to progression from a high-grade, compensated lesion to complete or critical obstruction.
Diagnosis of Ischemic Nephropathy
Given the large population of atherosclerotic patients and the nonspecific nature of the presentation, the diagnosis of IN is often considered in an extremely large number of patients. A cost-efficient, effective, and safe diagnostic strategy is desirable to identify these patients with IN. This section addresses various tests available. A recommended strategy for diagnosis is then presented.
There is an extensive body of literature and many reviews on the diagnosis of renovascular hypertension (RVH), but there is a relative dearth of data on the diagnosis of IN. To what degree this literature on RVH can be applied to the setting of IN is not clear. The tests that depend on the presence of a high renin state or high angiotensin II (Ang II) tone would not be expected to extrapolate well to the diagnosis of IN given the differences in pathophysiology of the disorders. However, data derived from the RVH literature on the anatomic diagnosis of RAS are useful. A meta-analysis published in 2001 by Vasbinder et al. evaluated the literature on diagnostic tests for renal artery stenosis in patients suspected of having RVH. These authors constructed receiver-operating characteristic (ROC) curves, and these are shown in Figure 77.1 . From this analysis they concluded that computed tomography angiography (CTA) and gadolinium-enhanced three-dimensional magnetic resonance angiography (MRA) were the most reliable noninvasive tests when compared to the gold standard of renal angiography. The authors also noted that few studies on these tests had been published and recommended further research in this area. In this effort, this same Dutch group later published their results of a prospective study of these two diagnostic modalities compared to intra-arterial DSA in this setting. They found suboptimal diagnostic characteristics of CTA and MRA, with the combined sensitivity and specificity of 64 and 92% for CTA and 62 and 84% for MRA. They concluded that CTA and MRA are not sufficiently sensitive to rule out RAS in hypertensive patients, and they contended that DSA is the diagnostic method of choice.

Angiography
Angiography remains the single most accurate test to diagnose and quantitate renal artery disease. This may be performed with conventional technique but has largely been supplanted by intra-arterial Digital Subtraction Angiography (DSA) technique. Although intravenous DSA was once considered as a less invasive alternative to conventional angiography, in that it does not require arterial catheterization, it still requires nephrotoxic radiocontrast which limits its utility in patients with renal insufficiency. In contrast intra-arterial DSA can be performed with small volumes of contrast, and it has been reported to have similar sensitivity as conventional angiography. Intra-arterial angiography can be accomplished using carbon dioxide, however, the images are of lesser quality and may create greater uncertainty about lesion severity unless combined with judicious use of iodinated contrast.
Renal angiography during intra-arterial angiography performed for other indications may be a reasonable diagnostic strategy, for example, during evaluation for aortic disease, lower extremity peripheral arterial occlusive disease, or coronary artery disease. When a cardiologist performs a renal angiography during a cardiac catheterization that is done through the femoral approach (pejoratively termed “drive-by” renal angiography), essentially the only incremental risk is that of the minimal volume of contrast needed to visualize the renal arteries. The suprarenal aorta has already been traversed for the coronary study, and therefore the risk for lower extremity and renal embolic sequelae has already been realized. The limitations of noninvasive evaluation for RAS (as discussed previously), the high incidence of incidental RAS in this population, the prolonged phase of silent progression that may result in irreversible renal injury when it finally becomes clinically manifest, and the potential for interrupting the course of IN (to be discussed in the following sections) have been the justification for routinely including this renal artery evaluation during coronary catheterizations done via the femoral approach. Apposing this is the increased contrast exposure resulting from additional angiographic images of the abdominal and scraping of the aorta by the catheters (with an attendant risk of atheroembolization) if selective images of the renal arteries is performed. To balance these risks consensus guidelines were developed and published. The more difficult issue involves the decision of whether to place a renal artery stent during the procedure and this issue requires circumspection, knowledge of the competing risks and benefits of revascularization versus medical management, and informed consent from the patient.
Duplex Ultrasonography
Another anatomic test for RAS that has a role in noninvasive evaluation of RAS is duplex ultrasonography. There are several major advantages of Duplex, namely that it is entirely safe and that the data provides functional information about lesion severity (systolic velocity and turbulence of flow) as well as anatomic information (lesion location). However, as shown in the meta-analysis by Vasbinder, it appears to have inferior diagnostic characteristics compared with MRA and CTA. This technique involves B-mode imaging with a pulsed Doppler beam to measure end-diastolic to peak systolic velocities in the renal artery and aorta. Some centers have reported sensitivity of 92.5 to 98% and specificity of 96 to 98%. It is an operator-dependent test, and although diagnostic accuracy is improving, it will require further improvements to become the universal noninvasive test of choice. Obesity, recent food intake, increased bowel gas, and adhesions from prior surgery may limit the quality of images and may hinder diagnostic utility.
Functional Testing for RAS
Given the suboptimal characteristics of these anatomic tests, the functional tests—or “hemodynamic probes” as some authors have called them—may be useful in certain settings. Most rely on the interruption of Ang II–maintained GFR or the lowering of renal perfusion distal to a lesion by inducing controlled systemic hypotension. Textor et al. have shown that nitroprusside-controlled lowering of arterial blood pressures will decrease GFR in individuals with bilateral RAS, but the logistics preclude using this as a common diagnostic test. Combining the use of captopril with renal scintigraphy, may yield helpful information about blood flow beyond a stenotic lesion in the renal artery. The most commonly used renal scans are the 99mTc-DTPA scan (which estimates GFR) and the 131I-iodohippurate scan (Hippuran) (which estimates renal plasma flow). The 99mTc-mercaptoacetyltriglycine (Mag3) combines the advantages of both of these, and can be used to quantify the relative blood flow to each kidney. However, several studies have demonstrated poor performance of renal scintigraphy for the evaluation of renal artery stenosis. In one circumstance, the evaluation of renal function in a patient with an occluded renal artery, scintigraphy may still be useful if it demonstrates significant residual function of the post-occlusion kidney.
Diagnostic Strategies
When one has at least a moderate suspicion of the disease and when a finding of RAS would guide management, many experts feel that an anatomic test for RAS should be pursued. Unless an angiogram is planned for a separate indication (such as to evaluate for coronary artery disease), a noninvasive evaluation is generally a prudent next step. Until recently MRA with gadolinium would be considered, however, in patients with IN and an estimated GFR less than 40 ml/min/1.72m 2 the risk of nephrogenic systemic fibrosis, is prohibitive. If the patient has normal renal function (which is generally not the case when the diagnosis is considered in the evaluation of ischemic nephropathy), CT angiogram is an acceptable alternative. Duplex ultrasonography is a useful alternative since it is entirely non-invasive, safe, and provides information on the functional significance of lesion severity as measured by the flow velocity. If a coronary catheterization or aortic angiography is being done on such a patient, then the angiographer should visualize the renal arteries as part of the study. In selected patients it may be justified to proceed directly to angiography if the pre-test suspicion is sufficiently high and the other modalities are contraindicated (MRA or CTA because of contrast concerns) or the images are unlikely to be of diagnostic utility (example: morbid obesity and Duplex ultrasonography). CO 2 may be used as an adjunct to limit the volume of iodinated radiocontrast in individuals at risk for radiocontrast nephropathy.
Clinical Significance of Atherosclerotic Renal Artery Stenosis
After the diagnosis of a renal artery lesion is secure, the next step is to assess the clinical significance of the lesion in hopes of prospectively identifying patients who will benefit from therapy. In this regard, it is important to have an appreciation of the anatomic natural history, the clinical natural history, and the pathophysiology of the disorder. These issues will be addressed later in the chapter. Several diagnostic tests have been proposed to test the physiologic and clinical significance of a given lesion, and these are reviewed here.
A parameter that may have a role in assessing the clinical significance of a lesion and in guiding the use of invasive revascularization interventions is that of the sonographically measured renal resistance-index value. It has been hypothesized that intrarenal small-vessel disease may limit the benefit of main renal artery revascularization in patients with atherosclerotic RAS, and increased intrarenal renal vascular resistance may be a functional marker of this microvascular disease. The sonographically determined resistance index (RI) is considered a noninvasive measure of intra-renal resistance to blood flow, and it is calculated as follows:
RI = [ 1 − ( end – diastolic velocity / maximal systolic velocity ) ] × 100
Radermacher et al. assessed the prognostic value of this parameter among 138 patients who had unilateral or bilateral RAS of at least 50% of the luminal diameter and who underwent angioplasty or surgical revascularization. Their data indicated that a resistive-index value of at least 80 reliably identifies patients in whom angioplasty or surgery will not improve renal function, blood pressure, or kidney survival. Others, such as the group led by Hansen, have demonstrated that a high resistive index was associated with loss of GFR post-revascularization and high long-term mortality, but not with blood pressure response.
Hemodynamic Assessment of RAS
The physiologic tests or “hemodynamic probes” may also have a role in this setting. As will be discussed later in the chapter, GFR is maintained in settings of reduced renal perfusion (such as may be the case with a high-grade RAS lesion) by maintenance of increased efferent arteriolar tone by Ang II. These tests are intended to demonstrate whether there is a drop in GFR distal to a RAS lesion after the pharmacologic interruption with an ACE inhibitor (ACE-I) of Ang II–maintained efferent arteriolar tone. Safian and Textor suggest using captopril renography with nuclear scans to assess the functional significance of lesions by determining the renal function of the individual kidneys and by determining the dependence of renal function on Ang II. As described above, the practice of using scintigraphy diagnostically though has not been validated. In contrast there is a growing literature about the use of pressure gradients measured during invasive angiography, either at rest or with a pharmacologic agent, to predict outcome of revascularization. These investigators have observed that a higher pressure gradient (>21 mmHg) induced by intra-arterial papaverine was associated with an improvement in blood pressure post-procedure.
Another important issue that must be considered when assessing the clinical significance of an individual lesion is what degree of stenosis is considered hemodynamically significant? The most important consideration is the cross-sectional area through which renal blood flows. Assuming that the stenotic lesion narrows the lumen circumferentially, the luminal can be calculated as the area of a circle by multiplying pi by the square of the radius (A=π r 2 ). The severity of stenosis is generally assessed clinically by an estimation of the degree of reduction of luminal diameter (rather than luminal area) on angiographic longitudinal projections. Thus, decreasing a luminal radius or diameter to half would reduce the luminal area to one quarter. A basic understanding of fluid dynamics is also helpful in understanding the flow and pressure effects of the length and degree of stenotic lesions. This rearranged version of Poiseulle’s equation shows the relationship of the parameters:
P1 − P2 = 8 [ Q × n × 1 / π × r 4 ]
where Q=flow, n=viscosity coefficient, r=radius, l=length of narrowing, and P1−P2=pressure gradient across the stenosis. Obviously the situation is much more complicated in a physiologic system with distensible blood vessels and with pulsatile, less-than-perfectly laminar flow. Nevertheless, the equation indicates that the radius of the stenosis should be much more hemodynamically important than length of the lesion: a reduction of radius by half would increase the pressure gradient by a factor of 16 while a doubling of the length would increase the gradient by a factor of 2.
With these considerations in mind, it should be noted that autoregulation fails to maintain GFR below SBPs of 75 to 80 mm Hg and that experts have stated that this occurs when reduction of luminal diameter is reduced by 70%. Classic studies on dogs by May et al. in the early 1960s support the contention that the degree of obstruction must exceed 70 to 80% of the cross-sectional luminal area before appreciable pressure gradients develop across a lesion. Using cardiac-gated magnetic resonance cine phase-contrast flow measurements with high temporal resolution in a canine model of renal artery stenosis, Schoenberg and colleagues made more sophisticated descriptions of pulsatile flow profiles and pressure gradients to describe the immediate hemodynamic impact of various degrees of stenosis (as measured by reductions in luminal diameter). As shown in Figure 77.2 , the area under the single cardiac-cycle flow-versus-time curve as a measure of mean flow did not substantially decrease until the degree of stenosis reached levels between 70 and 90%. They also showed that trans-stenotic pressure did not significantly change until the animal was confronted with similarly high degrees of stenosis. As shown in Fig. 77.2 , the only significant flow abnormality observed at lesser degrees of stenosis was a gradual loss of the early systolic peak of flow. The ultimate clinical significance of such subtle flow changes is not known. Raising concern that lesser degrees of obstruction may have a long-term deleterious impact, Myers et al. found that subcritical (<65%) stenosis is a significant risk factor for progressive chronic kidney disease in hypertensive patients with type 2 diabetes mellitus and azotemia (serum creatinine of at least 1.8 mg/dl), as shown in Figure 77.3 . This finding raises the possibility that large-vessel RAS may also be a marker for progressive small vessel disease. As described above the pressure gradient across the stenosis may be predictive of the response to revascularization, although this remains somewhat controversial. A concept that has received little attention is the relationship between intrarenal resistance and translesional pressure gradients. For example, it is possible that translesional gradients are lower when post-lesion intra-renal resistance is high. The drop in pressure sensed by the kidney is likely dependent upon the relative degree of obstruction of the renal artery before the kidney and the amount of intra-renal resistance to flow. Whether these factor(s) are dynamic or static, and their relationship within patients and to the response to revascularization has not been explored experimentally.


Assessment of Kidney Size
The global renal atrophy of IN is an important noninvasive marker of the disorder, and its implications on the reversibility and prognosis of disease may be different than that in other chronic renal diseases, where small kidney size is widely considered a marker of irreversibility. Given the distinct pathophysiology of IN, it is not clear when reduced renal size indicates “death” versus “hibernation” of the kidney. Caps et al. elucidated the incidence of renal atrophy when they followed 204 kidneys in 122 subjects prospectively with serial (every 6 months) duplex scans for a mean of 33 months and observed that the 2-year cumulative incidence of renal atrophy (defined as >1 cm reduction in length) was 5.5, 11.7, and 20.8% when the index scan revealed a normal artery, less than 60% stenosis, and greater than or equal to 60% stenosis, respectively. They also found that systolic blood pressure (SBP) above 180 mm Hg and elevations in creatinine also correlated with atrophy. Greco and Breyer contend in their 1997 review of atherosclerotic renal disease that renal atrophy is reversible, and they cite evidence from studies that indicate good renal functional outcomes following surgical revascularization of small kidneys and that indicate that renal size may increase after revascularization. Tubular atrophy and glomerular collapse, which are generally considered reversible, are often seen in IN, and perhaps this could contribute to renal shrinkage in this setting. Alternatively, glomerular sclerosis and interstitial fibrosis could be present, and this pathology is considered irreversible. A renal biopsy could be used to distinguish between these causes of atrophy and gauge prognosis and guide therapy, but this is rarely done in this setting.
Despite the availability of these various anatomic and hemodynamic tests, the current state of the art in predicting which patients with RAS will benefit from invasive revascularization is inadequate. As will be discussed, the literature on treatment of RAS indicates that a substantial proportion of patients with RAS do not benefit from or are harmed by revascularization procedures. To understand why repairing a stenosis in the renal artery might not work, one must have an appreciation of the pathophysiology of IN.
Pathophysiology of Renal Underperfusion and Ischemic Nephropathy
The classic experiments of Goldblatt provide insight into the acute physiologic alterations that result in renovascular hypertension following constriction of the renal artery. Goldblatt produced in vivo renal artery stenosis by applying a metal clip to partially occlude the renal artery. In the Goldblatt two-kidney–one clip model of renal artery stenosis, the hypertensive state that results is associated with increased renin levels. However, because of pressure natriuresis in the nonstenotic kidney, volume status is normal. This situation approximates unilateral stenosis in an individual with two functioning kidneys. In contrast, the one clip-one kidney model is characterized by normal renin levels and hypervolemia. Volume expansion persists due to the absence of a compensatory pressure natriuresis in an unaffected contralateral kidney. Further studies have demonstrated that the renin dependence of the two-kidney–one-clip model is not permanent. During the first few months following renal artery constriction, renin levels are high and removal of the clip will reverse the hypertensive state (known as phase I). This evolves to a state in which renin declines, but in which hypertension is still reversible (phase II), and finally to a state in which removal of the clip does not reverse the hypertension (phase III). These experiments, along with many subsequent studies, have made it clear that the pathophysiology of renal artery stenosis is at least partly time-dependent, that RAS may result in irreversible renal damage, and that the anatomic correction of RAS lesions does not necessarily correct the underlying pathophysiology. Understanding why this is the case requires an understanding of the physiology of renal autoregulation, the pathophysiology of prolonged states of renal underperfusion, and the causes of nephropathy in the clinical scenarios of renal ischemia.
Adaptive Responses to Renal Underperfusion
The unique positioning and independent control of resistance vessels proximal and distal to the glomerular capillary loops, the afferent and efferent arterioles, provide the kidney with an effective means of regulating the hydraulic forces driving glomerular filtration. An understanding of the hemodynamic effects of Ang II in renal artery occlusion requires some knowledge of the determinants of GFR. A summary of the interactions between these determinants is shown in the Figure 77.4 . The rate of filtrate formation depends on several dynamic variables: systemic blood pressure, plasma flow rate (QA), glomerular hydraulic pressure (PGC), and afferent and efferent arteriolar resistance (RA and RE, respectively). In general, the driving force for filtration is PGC, which is determined by the systemic blood pressure, QA and the relative pre- and postglomerular resistances, RA and RE. For any given pressure gradient across the glomerular capillary wall, the absolute rate of filtrate formation also depends on the ultrafiltration coefficient (Kf), which is the product of the capillary surface area and the intrinsic hydraulic permeability of the capillary wall. As renal perfusion pressure declines, the afferent arteriole dilates and total renal vascular resistance declines. This vasodilation appears to be primarily an intrinsic, myogenic response to the decline in perfusion pressure. In addition to the direct effect of reducing perfusion pressure on the afferent arteriole, a decrease in solute delivery to the macula densa region of the distal tubule elicits vasodilation in the afferent arterioles. This communication between the macula densa cells and the afferent arteriole is termed “tubuloglomerular feedback” (TGF). The exact mechanisms responsible for this action are not completely understood. Potential local mediators include adenosine, thromboxanes, and nitric oxide.

The most common cause of a reduction in renal artery pressure is low systemic blood pressure. Under circumstances in which systemic blood pressure is reduced, circulating levels of several vasoconstrictive factors, including Ang II, arginine vasopressin (AVP), the sympathetic nervous system, and plasma catecholamines rise, leading to an increase peripheral resistance,. If renal vascular resistance increased in proportion to systemic vascular resistance, renal plasma flow, glomerular capillary pressure, and GFR would fall. However, at least two mechanisms combine to differentially alter the the response of the renal vasculature to a reduction in perfusion pressure and to preserve GFR; increased renal prostaglandin production leading to a reduction in afferent arteriolar resistance and the relative constriction of the efferent arterioles.
Renal prostaglandins are autocoids that are derived from the cyclo-oxygenase (COX) enzyme-catalyzed metabolism of arachadonic acid. Prostaglandins synthesized in the kidney act locally and have minimal systemic effects. They oppose the vasoconstrictive effects of Ang II, AVP, and increased renal nerve activity on the afferent arteriole, resulting in a net reduction in afferent arteriolar resistance in response to decreased renal artery pressure. In this regard, prostacyclin and prostaglandin E2 (PGE2) appear to be the most important renal prostaglandins. The renal prostaglandin system appears to be inactive and insignificant in states of normal renal perfusion, and inhibition of the renal prostaglandin system in health typically has few detectable effects. However, under circumstances in which systemic vascular resistance is increased in order to preserve blood pressure, renal prostaglandin synthesis and release dramatically increase. It is under these circumstances that inhibition of renal prostaglandins would produce deleterious effects on renal perfusion and glomerular filtration. This explains the well-known adverse effect on GFR that has been observed when a nonsteroidal anti-inflammatory drug (NSAID) is administered to patients with conditions that impair renal perfusion such as true volume depletion, effective circulating volume depletion (such as liver failure), and states of low cardiac output (such as CHF). Hemodynamically, significant RAS is another condition in which inhibition of prostaglandin synthesis could adversely effect GFR.
The second factor that preserves GFR during reductions in renal artery pressure is preferential constriction of the efferent arterial in response to increased local concentrations of Ang II. The increase in efferent arteriolar resistance is associated with an increase in total renal vascular resistance and a decrease in renal plasma flow; however, because of the downstream location of this resistive force relative to the filtering unit (glomerulus), PGC remains constant tending to maintain GFR. In this manner, the kidney is able to increase the filtration fraction (GFR/renal plasma flow [RPF]) and preserve GFR despite reductions in renal plasma flow, such as occur in states of reduced cardiac output, volume depletion, or renal artery obstruction. There is also evidence that norepinephrine and the renal sympathetic nerves (possibly via Ang II) preferentially constrict the efferent arteriole relative to the afferent arteriole.
Given the dependence of P GC and GFR on Ang II during reductions in renal perfusion pressure, it would be expected that pharmacologic interruption of the renin/angiotensin/aldosterone system could have clinically important effects, and this is clearly the case. When ACE-I therapy decreases Ang II production or when angiotensin receptor blockers inhibit Ang II effects in conditions of increased vasoconstrictor tone, efferent resistance may decline leading to clinically important reductions in PGC and GFR. The magnitude of the effect of administration of an ACE-I on GFR depends in large part upon the local Ang II level and on systemic hemodynamics. If ACE-I administration fails to improve systemic hemodynamic parameters such as cardiac output, is associated with a marked decline in systemic blood pressure or is administered in a setting where GFR is markedly dependent on efferent arteriolar constriction, then RE will fall sharply and PGC and GFR will decline. As might be expected, this situation may exist when the blood flow through the renal artery is impaired by a fixed obstruction.
It is important to appreciate that the effects of ACE inhibition in the stenotic or ischemic, underperfused kidney are theoretically different from those in other progressive renal diseases, where renal artery pressure is not is not reduced. In the latter circumstance, glomerular hyperfiltration and glomerular hypertension may contribute to the progressive injury and scarring that leads to loss of function. In that setting, the effect of ACE-I and angiotensin II receptor blocker (ARB) therapy to dilate the efferent arteriole and lower glomerular capillary is beneficial in slowing the rate of progression of chronic diseases such as diabetic renal disease. The renal protective effects of ACE-I and ARB may also be relevant to the non-stenotic kidney in patients with unilateral ischemic renal disease. ACE-I and ARB therapy may also be useful in reducing the amount of proteinuria in chronic glomerular diseases. The chronically ischemic, underperfused kidney distal to an obstructive renal artery lesion, on the other hand, may respond differently to ACE-I therapy if there is dependence on Ang II to maintain a physiologically acceptable GFR.
Pathophysiology of Ischemic Nephropathy
The kidneys receive a blood supply that is three- to fivefold higher than other metabolically active organs such as heart and liver. There appears to be a critical perfusion pressure of about 70 to 80 mm Hg (which generally correlates with a greater than 70% stenosis in a systemically normotensive individual), below which the adaptive physiologic mechanisms described previously are no longer able to sustain GFR. Given that less than 10% of the oxygenated blood flow to the kidney is needed for metabolic requirements of the kidney, it would be expected that reductions in excretory function would occur in an affected kidney well before major ischemic injury develops. The intermediate steps by which this initially reversible decline in GFR then progresses into structural changes in the kidney are not well understood. The pathological changes do not appear to be directly a result of tissue hypoxia, as supported by the following observations: (1) the kidney is endowed with a generous supply of oxygen; (2) as renal artery pressure and GFR decline as a result of decreased perfusion pressure, active transport and solute reabsorption also cease, markedly reducing oxygen utilization by the kidney; (3) measurements of oxygen tension in the renal veins of patients with renal artery stenosis do not demonstrate desaturation; (4) there is no augmentation of erythropoietin production from the affected kidney; and (5) IN develops commonly in individuals with atherosclerotic RAS, but rarely develops distal to RAS lesions of similar hemodynamic impact produced by fibromuscular dysplasia (FMD). Based on these observations, it appears that ischemic nephropathy is not simply the result of global hypoxia of the kidney and that other mechanisms are at play.
The precise pathophysiology by which RAS causes IN is not well understood. In addition to or as a result of the ischemia of the kidney, the process probably involves a complex interaction of oxidative, vasoactive, apoptotic, fibrogenic, inflammatory, atheroembolic, and micro-atherosclerotic factors. The relationship and relative importance of these various factors will require further study. An understanding of these mechanisms will be critical in developing optimal treatment strategies and guiding a rational use of medical and invasive interventions. A review by Lerman and Textor addresses in detail the pathophysiology of ischemic nephropathy, and their schematic diagram, shown in Figure 77.5 , lists many of these proposed pathways and indicates complex interactions among them. Several of these factors will be addressed in the following sections. The main reason for understanding the IN process in the kidney ipsilateral to renal artery stenosis is that the presence of IN may underlie the failure in renal function and blood pressure to improve after the renal artery obstruction is corrected.

Atherosclerosis
As stated previously, IN develops commonly in individuals with atherosclerotic RAS, but it rarely develops distal to RAS lesions of similar hemodynamic impact produced by FMD. The sparing of patients with FMD, who are generally younger women and otherwise free of atherogenic risk factors, suggest that additional factors related to atherosclerosis [diabetes, hyperlipidemia, platelet activation or atheroembolization] may contribute significantly to kidney injury. Chade et al. demonstrated using the porcine model of RAS that hypercholesterolemia superimposed on RAS accelerates the development of fibrosis in the stenotic kidney. Clinically, high resistance to flow in smaller arteries of the kidney (sonographically measured noninvasively by calculating intrarenal resistive index of the segmental arteries) has been shown to be highly predictive of poor renal recovery after successful revascularization, and this may be due to atheromatous intrarenal small vessel disease. Interestingly, in patients with atherosclerotic renal disease a combination of vascular sclerosis, interstitial fibrosis, inflammatory cell infiltration, atubular glomeruli, and focal or global glomerulosclerosis is seen when biopsy of the affected kidney is performed. While some of these changes are seen in animal models of experimentally induced renal stenosis, “this mixture (of changes) cannot be reproduced in animal models. ”
Redistribution of Intrarenal Blood Flow
In experimental models of reduced renal artery blood flow, relative blood flow is altered to favor the corticomedullary circulation over more superficial cortical areas. This probably serves to protect the more ischemic medullary parenchyma. The changes in flow distribution are probably mediated by various vasoactive substances such as prostaglandin E2, nitric oxide, urodilatin, endothelin, Ang II, and adenosine. The involvement of this mechanism is supported by findings by Mounier-Vehier et al., which show that a reduction in ultrasound-measured cortical thickness is an early morphologic marker of atherosclerotic renal disease, one that tends to develop before gross renal length decreases.
Atheroemboli
Histopathologic abnormalities in IN often include evidence of renal cholesterol microembolization, and the cholesterol emboli syndrome can be an obvious complication of angioplasty or surgery. The cholesterol microemboli may lodge in a small artery and impair distal blood flow. The ensuing foreign body reaction causes intimal proliferation, giant cell formation, and further narrowing of the vascular lumen. The microemboli seem to elicit an immune reaction, as hypocomplementemia, eosinophilia, and eosinophiluria are frequently observed with the cholesterol emboli syndrome. Work by Edwards, Hiramoto and others data suggests that a large number of emboli may be released during renal stenting, This has led to interest in devices to capture emboli liberated during stenting. As stated above, evidence for spontaneous atheroemboli can be demonstrated with renal biopsy, although the degree to which this contributes to loss of kidney function is not known.
Imbalance of Vasoconstrictors
One of the predominant effects of renal artery stenosis may be activation of the renin-angiotensin-aldosterone axis. The decrease in renal blood flow in animal models of RAS can be blunted by Ang II receptor blockers, suggesting that Ang II plays a role in renal ischemia. On the other hand, effects of Ang II blockade by ACE-Is have also been shown to accentuate the renal atrophic changes in the two-kidney–one-clip model independent of the effects of lowering of systemic blood pressure. In addition to Ang II, other potent vasoconstrictors such as thromboxane and endothelin appear to be activated by ischemic insults. The renal vasculature is especially sensitive to the effects of endothelin, which causes afferent and efferent arteriolar vasoconstriction and decreases the filtration coefficient (Kf), producing a net decline in GFR. In experimental models of acute and chronic ischemia (the latter induced by cyclosporine administration), functional and morphologic changes can be blunted by administration of anti-endothelin antibodies. High levels of endothelin have been observed in chronic renal ischemia models as well as in renal hypoperfusion in the setting of hepatorenal syndrome. However, systemic endothelin levels do not appear to be elevated in patients with renovascular disease when compared with patients with essential hypertension, suggesting that if endothelin plays a role in human ischemic renal disease, it must be intrarenal.
Reduced Shear Stress
Under conditions of hypoperfusion, the normal counterbalancing release of vasodilator substances such as prostaglandins and NO may be impaired, thereby accentuating the ischemia. One of the reasons for this may be a loss of shear stress due to decreased renal blood flow. Even subtle reductions of perfusion pressure and flow, as demonstrated by Schoenberg ( Fig. 77.2 ), could conceivably initiate injury through this mechanism. It has been shown that shear stress is a major regulatory mechanism for production of nitric oxide.
Oxidative Stress
As discussed previously, hemodynamically significant RAS stimulates the renin-angiotensin system, resulting in increased production of Ang II. It has also been reported that Ang II stimulates the production of reactive oxygen species such as superoxide, probably through the activation of membrane-bound nicotinamide adenine dinucleotide (NADH) or NADPH (nicotinamide adenine dinucleotide phosphate) oxidase. The pro-oxidant effects of Ang II may interact with those of coexisting metabolic disorders and hypertension such that a relative increase in reactive oxygen species (especially superoxide) and reduced production of nitric oxide (NO) may be present. This imbalance may promote endothelial dysfunction by a resultant increase in oxidative stress. Lerman et al. demonstrated increased oxidative stress in their pig model of RAS. Higashi et al. demonstrated evidence of excessive oxidative stress in patients with RVH that appeared to reverse after angioplasty and was associated with evidence of endothelial dyfunction that also improved after angioplasty. Minuz et al. showed that oxidative stress, as indicated by measurements of lipid peroxidation products, is increased in patients with renovascular disease and hypertension as compared with patients with essential hypertension. Furthermore, this appeared to be due to activation of the renin-angiotensin system, and it was associated with an increase in thromboxane A2 biosynthesis, which would be expected to enhance platelet activation. Enhanced platelet aggregation and adhesion could contribute to microvascular renal injury as well as cardiovascular disease associated with this disorder. Additionally, this oxidative stress and reduced NO production may impair endothelium-dependent vasodilation, which may have local and systemic consequences. Locally, the resulting vasoconstriction could increase ischemic tissue injury. Systemically, hypertension could result. Increased free-oxygen radicals could also impact on fibrogenic and inflammatory mediators. Chade et al. demonstrated in the porcine model of RAS described previously that chronic blockade of the oxidative stress pathway using antioxidant vitamins improved renal hemodynamics and decreased inflammation and fibrosis in the ischemic kidney.
Fibrogenic Factors
Fibrogenic, trophic, and inflammatory mediators are probably involved in the progression of IN. These may include nuclear factor kappa B (NFκB), tumor necrosis factor (TNF), transforming growth factor beta (TGF-β), interleukin-1 and others. Pathologically, interstitial fibrosis and glomerular sclerosis are often seen in chronically ischemic kidneys. Chade et al. demonstrated in pigs increased expression of TGF-β, increased trichrome staining (indicating collagen deposition), as well as more prominent interstitial fibrosis, tubular atrophy, and glomerular sclerosis in kidneys distal to a RAS lesion.
Inflammation
Inflammatory responses to the altered ischemic milieu may also contribute to the renal injury. Histological examination of ischemic kidneys often shows evidence of mononuclear infiltrates, tubulitis, and interstitial fibrosis. Immunoglobulin and complement deposition has been noted in the areas of sclerosis in human ischemic kidneys. The inflammatory process is likely initiated by alterations in cellular antigenicity induced by ischemia. Inflammation may then contribute to interstitial fibrosis and atherosclerosis.
Disruption of Cellular Membrane Integrity and Intracellular Milieu
Ischemic injury can result in derangements of cellular membrane pumps responsible for maintenance of the internal milieu. One of the key events in this process may be calcium influx. Anoxic injury results in a rapid increase in cytosolic calcium, which can impair several vital cell functions. This may explain the protective effects of calcium channel blockers in some models of ischemic injury. Mitochondrial calcium uptake impairs oxidative phosphorylation, further reducing cellular adenosine triphosphate (ATP) levels. In the acute kidney injury model, an increase in cytosolic calcium also activates phospholipases and generates toxic oxygen radicals, which can cause further membrane damage. Ischemia delays the recovery of adenine nucleotide levels, and leads to depletion of high-energy stores from phosphate bonds in the acute kidney injury model. It has been shown that a decrease in cellular ATP by 50% is associated with severe apical microfilament disruption and cytoplasmic actin polymerization, and these changes can be reversed by increasing the cellular ATP to 70% of control values. Van Why and others have devised an in vivo ischemic model, which results in graded ATP depletion. They have shown generation of heat-shock proteins when cellular ATP decreased to 50 to 60% of control values.
Apoptosis
Enhanced renal apoptotic activity is evident within one week of unilateral RAS, but whether there is true cellular reduction or whether it represents a natural compensation for the hyperplastic response after injury is unclear. It appears that enhanced apoptosis is ongoing for several months after an insult, a finding that suggests that apoptosis is necessary for remodeling of tubular structure. Apoptosis occurs primarily in the thick ascending limb after an acute ischemic insult. Tubular atrophy is commonly observed by routine histology and is primarily due to a decrease in cell size. This is accompanied by a decrease in cell organelles and cellular protein content. It has been noted that the autophagolysosome activity, which increases degradation of cell organelles, is transiently increased in ischemic kidneys. Apoptosis likely contributes to the global renal atrophy that is observed in IN.
Other Issues Pertinent to the Pathophysiology of IN
The true hemodynamic events in IN likely involve numerous,transient acute reductions superimposed on chronic, progressive and, eventually, a critical reduction in blood flow to the involved kidney. Acute hypoperfusion and chronic, slowly progressive ischemia clearly have different consequences for the kidney. It must be noted that some of the hypotheses discussed above have been derived in large part from data obtained in models of acute ischemic kidney injury or isolated chronic ischemia models that do not adequately incorporate the complex combination of hemodynamic, metabolic, and atherogenic insults that typically exist in human disease. Lerman et al. developed a swine model of renal artery stenosis, which is induced by the intravascular placement of a local irritant coil in the renal artery, and the gradual and progressive renal artery narrowing in this model resembles human RAS lesion progression and has been useful in elucidating mechanisms of injury. The administration of a high-cholesterol diet has been used to produce an atherogenic milieu in the animals. However this model probably does not incorporate repetitive acute ischemic insults, which have been postulated to be important in human IN and appear to provoke delayed, cumulative pro-fibrotic sequelae in the kidney.
Another important issue in the pathophysiology of IN is that renal blood flow may be maintained via collateral arterial supply to a kidney whose flow is impaired by a severely stenotic artery. Presumably, the collateral circulation would be more substantial in settings of a slowly progressive RAS lesion. Collateral arteries may arise from the suprarenal, lumbar, and ureteric vessels. Because of this collateral circulation, renal viability and function may be maintained despite occlusion of the main renal artery. In fact, salvage of renal function by revascularization of dialysis-dependent patients with IN has been associated with the presence of collateral circulation on angiogram.
Clinically, it is also important to understand that reductions in systemic blood pressure, even when the reductions are from frankly elevated to normal blood pressures, may further impair perfusion distal to a RAS lesion. When the kidney senses underperfusion, it releases renin thereby activating pressor and sodium-retaining mechanisms (primarily via the renin-angiotensin II–aldosterone system) that induce a state of systemic arterial hypertension in order to restore renal perfusion across the resistance of the stenotic lesion. This process of RAS producing systemic hypertension is known as renovascular hypertension (RVH), and a full discussion of this topic is beyond the scope of this chapter. Due to the risks of uncontrolled hypertension (which is often very severe or malignant in patients with RVH), antihypertensive medications are indicated and generally administered to such patients. Antihypertensive medications are usually effective in achieving a sustained normalization of systemic blood pressures. Interruption of RVH may lower the forward pressure head that pushes blood through the RAS lesion, and consequently worsen the perfusion to the post-stenotic kidney. This perfusion impairment may be severe enough that autoregulation fails to maintain GFR and that ischemic pathologic changes occur. Experimental observations by Michel et al. support this theoretical concern, as they demonstrated much more severe histopathologic changes (glomerular collapse and fibrosis) after 5 weeks of therapy with ACE inhibitor. Discussed below, there is now observational data suggesting a lower rate of dialysis for patients with RAS treated with ACE or ARB, however this may result from better preservation of the contralateral, non-stenotic kidney in patients with unilateral RAS, despite more rapid progression of IN in the post-stenotic kidney.
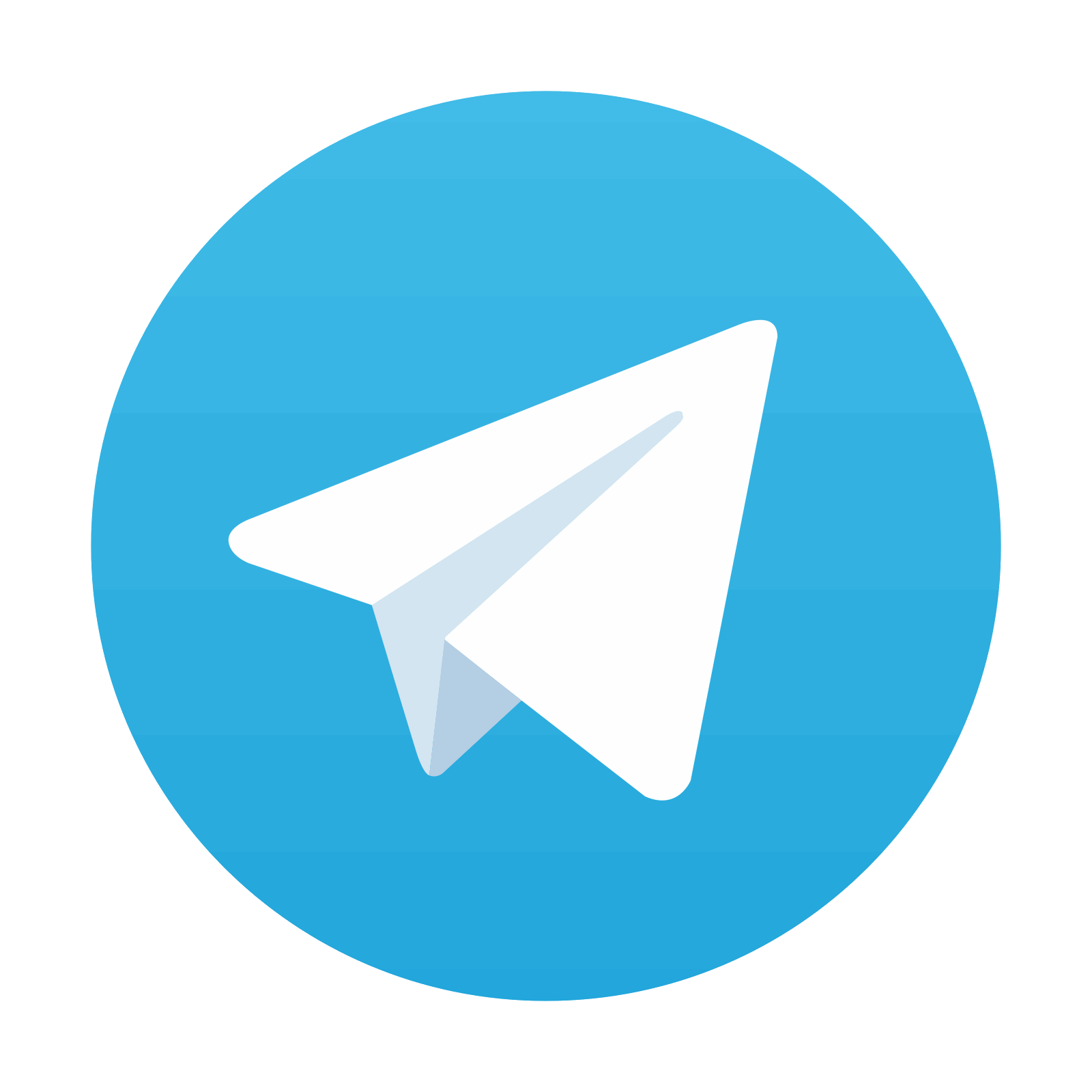
Stay updated, free articles. Join our Telegram channel

Full access? Get Clinical Tree
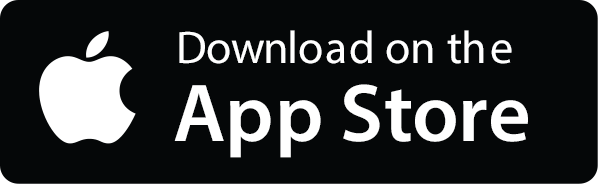
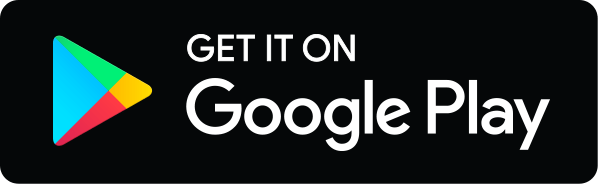