Abstract
Calcium, phosphate, and magnesium are important components of cell structure, energy metabolism, and signal transduction and are required for enzymatic activity. All of these minerals are important components of bone as well. The serum levels of these substances are tightly regulated in a narrow range with complex interactions of hormones, transporters, and receptors in many organ systems. Recent advances using animal models and humans have advanced our understanding of the regulation of these minerals. The kidneys play a critical role in fine-tuning the serum levels of these minerals. Inherited disorders of dysregulation of calcium, phosphate, and magnesium are described in detail in this chapter. Many of these disorders are present in the neonatal period, and it is vital to diagnose these disorders early so that appropriate therapy can be initiated. Although some disorders do not present in the neonatal period, it is important for the neonatologist to be aware of the later presenting conditions in order to diagnose them prior to the development of complications and to prevent late diagnosis of patients especially when there is positive family history. Understanding the inheritance of these genetic disorders can be used to provide counseling to the families.
Keywords
hypercalcemia, hypocalcemia, hypermagnesemia, hypomagnesemia, hypophosphatemia, hyperphosphatemia
- •
Regulation of Calcium, Magnesium, and Phosphate Involves Multiple Hormones. Genetic Disorders Affecting Any of These Regulators Result in Dysregulation of These Minerals’ Ions and Subsequently Cause Clinical Symptoms.
- •
All Three Mineral Ions Are Absorbed in a Transcellular and Paracellular Fashion (Absorption Through the Cell Versus Absorption in Between the Cells, Respectively).
- •
The Vast Majority of Calcium and Phosphorus Is Absorbed in the Proximal Tubule, Whereas Most of the Magnesium Is Absorbed in the Thick Ascending Limb.
- •
Fine-Tuning of Tubular Calcium and Magnesium Absorption Occurs in the Distal Convoluted Tubule. However, Fine-Tuning of Phosphorus in the Distal Tubule Has Not Been Described.
- •
A Major Driving Force for Calcium and Magnesium Absorption in the Thick Ascending Limb Is the Lumen-Positive Potential.
- •
A Requirement for Magnesium Absorption in the Distal Convoluted Tubule Is the Negative Membrane Potential.
- •
Patients With Magnesium Disturbances May Not Present With Renal but With Neurologic Symptoms.
Inherited Disorders of Calcium
Introduction
Calcium (Ca 2+ ) is critical for many biologic functions in the body, and the vast majority of Ca 2+ is stored in the bone. Ca 2+ is vital for bone formation in the form of calcium phosphate hydroxyapatite and plays crucial roles in enzymatic reactions, in signaling pathways, and electric membrane potentials. The Ca 2+ content of a term newborn is approximately 30 g compared with approximately 1000 g in an adult. Serum Ca 2+ represents approximately 1% of the total body Ca 2+ . Ca 2+ regulation is tightly controlled and involves a complex interplay between the bone, intestine, and kidneys ( Fig. 20.1 ).

Intestinal Ca 2+ absorption occurs in the small intestine in a saturable, transcellular fashion and in the ileum and colon in a nonsaturable, paracellular fashion. Intestinal Ca 2+ absorption depends highly on the systemic Ca 2+ concentration. In the newborn, approximately 60% of the oral intake of Ca 2+ is absorbed in the gastrointestinal tract.
The kidneys are a major regulator of Ca 2+ homeostasis by modifying tubular Ca 2+ reabsorption versus urinary loss. Complexed and free Ca 2+ is filtered by the renal glomerulus. Along the nephron, approximately 95% of the filtered Ca 2+ is reabsorbed. The vast majority of Ca 2+ is absorbed in the proximal tubule (60%–70%) and the thick ascending limb of Henle (TAL) (20%) across the paracellular pathway (between epithelial cells), in parallel with sodium (Na + ) and water. In the TAL the major driving force for Ca 2+ absorption is the lumen-positive potential difference. Fine-tuning of renal Ca 2+ reabsorption occurs in the distal convoluted tubule, where most of the remaining 10% of Ca 2+ is reabsorbed in a transcellular fashion via the renal Ca 2+ channel TRPV5 at the apical membrane ( Fig. 20.2 ). Inside the renal epithelial cell Ca 2+ is bound to calbindin-D 28K to avoid the risk of calcium-induced cell death. Notably, the intracellular free Ca 2+ concentration is approximately 100 nM (0.1 µmol/L), whereas the extracellular free Ca 2+ concentration is approximately 1 mM (2.5 mmol/L). On the basolateral side, Ca 2+ is extruded by the plasma membrane Ca 2+ ATPase (PMCA) and the sodium/calcium exchanger (NCX) (see Fig. 20.2 ). Parathyroid hormone (PTH) and 1,25-dihydroxy vitamin D3 (1,25 Vitamin D) both increase serum Ca 2+ , whereas calcitonin decreases Ca 2+ concentration ( Fig. 20.1 ).

Regulators of Calcium Homeostasis
A complicated system consisting of hormones (PTH, FGF23, calcitonin), receptors (PTH receptor, CaSR), and vitamins (vitamin D) regulate Ca 2+ homeostasis involving different organs, such as the parathyroid gland, intestine, bone, and kidneys. Dysfunction of these organs, hormones, vitamins, or their receptors results in disturbed Ca 2+ homeostasis and causes either hypercalcemia or hypocalcemia. For a review of these different components of Ca 2+ , PO 4 − , and Mg 2+ regulation, please refer to the chapter “ Perinatal Calcium and Phosphorus Metabolism .”
Hypercalcemia
Neonatal hypercalcemia is defined as total serum Ca 2+ concentrations greater than 10.5 mg/dL (>2.6 mmol/L).
Symptoms of Hypercalcemia
Hypercalcemia may remain asymptomatic or may cause polyuria, nephrocalcinosis, dehydration, renal failure, abdominal pain, constipation, nausea, pancreatitis, poor feeding, muscular hypotonia, bradycardia, shortened QTc interval, and lethargy.
A list of inherited causes of hypercalcemia is provided in Tables 20.1 and 20.2 .
Disorder | OMIM | Inheritance | Gene Locus | 1,25 (OH) 2 Vitamin D | PTH | Serum Ca 2+ | Urine Ca 2+ | Gene (Protein) | Function | References |
---|---|---|---|---|---|---|---|---|---|---|
Congenital hyperparathyroidism | *168461 | Sporadic | 11q13.3 | n /↑ | ↑ | ↑ | ↑ | CCND1/PRAD1 (Cyclin D1) | Phosphorylase which inactivates RB oncogene | |
Multiple endocrine neoplasias type 1 | #131100 | AD | 11q13.1 | n /↑ | ↑ | ↑ | ↑ | MEN1 (Menin) | Tumor suppressor | |
Multiple endocrine neoplasias type 2 | #171400 | AD | 10q11.2 | n /↑ | ↑ | ↑ | ↑ | RET (RET) | RET protooncogene is overexpressed | |
Hyperparathyroidism–jaw tumor syndrome | #145001 | AD | 1q31.2 | n /↑ | ↑ | ↑ | ↑ | HRPT2/CDC73 (Parafibromin) | Tumor suppressor | |
Familial hypocalciuric hypercalcemia type 1 (FHH1) | #145980 | AD | 3q13.3 | n /↑ | n /↑ | ↑ | ↓ | CASR (CaSR) | Ca 2+ -sensing receptor | |
FHH2 | #145981 | AD | 19p13.3 | n /↑ | n /↑ | ↑ | ↓ | GNA11 (Gα 11 ) | G protein signaling | |
FHH3 | #600740 | AD | 19q13.32 | n /↑ | n /↑ | ↑ | ↓ | AP2S1 (AP2σ1) | Clathrin adaptor protein | |
Neonatal severe hyperparathyroidism | #239200 | AR | 3q13.3 | n /↑ | ↑ | ↑ | ↑ | CASR (CaSR) | Ca 2+ -sensing receptor | |
Jansen metaphyseal chondrodysplasia | #156400 | AD | 3p21 | n /↑ | n /↓ | ↑ | ↑ | PTHR1 (PTHR1) | Constitutive active PTH receptor |
Disorder | OMIM | Inheritance | Gene Locus | 1,25 (OH) 2 Vitamin D | PTH | Serum Ca 2+ | Urine Ca 2+ | Gene (Protein) | Function | References |
---|---|---|---|---|---|---|---|---|---|---|
Severe infantile hypophosphatasia | #241500 | AR | 1p36.12 | n /↓ | ↓ | ↑ | ↑ | ALPL (alkaline phosphatase) | Pyrophosphatase | |
Idiopathic infantile hypercalcemia | #143880 | AR | 20q13.2 | n /↑ | ↓ | ↑ | ↑ | CYP24A1 (25-hydroxyvitamin D 24-hydroxylase) | Vitamin D metabolism | |
Williams-Beuren syndrome | #194050 | AD | 7q11.23 | n /↑ | n /↑ | n /↑ | n /↑ | ELN (elastin) | Connective tissue protein | |
Bartter syndrome type 1 | #601678 | AR | 15q15-q21.1 | n | n /↑ | n /↑ | ↑ | SLC12A1 (NKCC2) | Na + -K + -Cl − -cotransporter | |
Bartter syndrome type 2 | #241200 | AR | 11q24 | n | n /↑ | n | ↑ | KCNJ1 (ROMK) | K + channel | |
Bartter syndrome type 3 | #607364 | AR | 1p36 | n | n | n | n /↑ | CLCNKB (CLC-Kb) | Chloride channel | |
Bartter syndrome type 4 | #602522 | AR | 1p31 | n | n | n | ↑ | BSND (Barttin) | Subunit of CLC-Kb channel | |
Bartter syndrome type 5 | #300971 | XLR | Xp11.21 | n.d. | n.d. | n | ↑ | MAGED2 (melanoma associated antigen D2) | Modifies NCC and NKCC2 |
PTH-Dependent Hypercalcemia
Hypercalcemia Due to Hyperparathyroidism
Congenital Hyperparathyroidism/Primary Hyperparathyroidism.
Primary hyperparathyroidism is a rare condition caused by parathyroid hyperplasia and occurs by sporadic or autosomal recessive inheritance. Clinical presentation includes symptoms listed previously but also hypophosphatemia, hypercalciuria, and hyperphosphaturia. Bone demineralization and even osteitis fibrosa and fractures can occur. A renal ultrasound may reveal nephrocalcinosis.
Parathyroid tumors are a rare event in neonates, with adenomas being the most common. Parathyroid tumors occur either isolated or as part of a tumor syndrome (e.g., the multiple endocrine neoplasias [MENs] or hyperparathyroidism with jaw tumors). Parathyroid tumors can be due to heterozygous somatic mutations (gain-of-function mutation) resulting in overexpression of oncogenes, such as RET (causing MEN2) or PRAD1 (parathyroid adenoma 1) or recessive mutations in tumor-suppressor genes (loss-of-function mutation). MEN type 1 is caused by autosomal dominant mutations in the MEN1 gene (a tumor suppressor gene which encodes Menin) and is characterized by parathyroid, pancreatic, or pituitary tumors. MEN type 2 (MEN2) is due to activating, heterozygous mutations in the proto-oncogene RET1. These mutations typically result in hyperparathryroidism due to a parathyroid adenoma, medullary carcinoma of the thyroid, and pheochromocytoma. Parathyroid carcinoma and tumors of the mandible occur in a rare genetic syndrome called hyperparathyroidism–jaw tumor syndrome, which is caused by mutations in the gene HRPT2 (which encodes the tumor suppressor parafibromin).
The diagnosis of primary hyperparathyroidism is made by the detection of elevated PTH or inappropriately normal PTH levels given higher circulating Ca 2+ levels, 1,25 Vitamin D concentrations are elevated or normal, serum phosphate levels are low-normal or low, and alkaline phosphatase can be elevated due to bone disease. A spot urine Ca 2+ to creatinine ratio (normally <1 in infants) and a 24-hour urine collection enable the diagnosis of hypercalciuria. A renal ultrasound may detect nephrocalcinosis, and an x-ray of the wrist may demonstrate bone involvement. The treatment of choice is total parathyroidectomy with partial autotransplantation. Preoperatively, hyperparathyroid adenomas are detected by technetium 99m sestamibi scan. Following parathyroidectomy the influx of Ca 2+ into the healing bone may aggravate the duration of hypocalcemia, a phenomenon called “hungry bone syndrome.” In very low birth weight infants and newborns the removal of the parathyroid glands may be complicated by the small size of the parathyroid glands. Adjunct medical treatment in such cases is possible with the Ca 2+ -sensing receptor (CaSR) mimetic Cinacalcet, which may provide intermittent relief until surgery is an option.
Familial Hypocalciuric Hypercalcemia/Neonatal Severe Hyperparathyroidism.
Characteristics of familial hypocalciuric hypercalcemia (FHH) are mild hypercalcemia, inappropriately normal or mildly elevated PTH, and hypocalciuria. Genetically, three different forms of FHH (FHH1-3) were identified by genetic linkage. For FHH1 and FHH2, there are usually no signs of hypercalcemia and no nephrocalcinosis, and PTH levels remain normal; there may be mild hypermagnesemia, but overall patients remain asymptomatic. Rarely, pancreatitis and chondrocalcinosis may occur. For FHH1, inactivating mutations in the CaSR gene have been described that are inherited in an autosomal dominant fashion. To maintain Ca 2+ homeostasis within the body, a sensing mechanism for Ca 2+ is required. This function is provided by CaSR, which is a seven transmembrane–spanning G protein–coupled receptor (GPCR). The gene encoding the CaSR is most highly expressed in organs involved in Ca 2+ homeostasis, such as the parathyroid glands, C cells of the thyroid gland, bone, and kidneys. CaSR recognizes surprisingly minor perturbations in extracellular Ca 2+ concentration and reacts by adjusting PTH secretion. Stimulation of CaSR by Ca 2+ binding reduces PTH secretion via G protein–coupled signaling via Gα q/11 , Gα i , Gα 12/13 , and Gα qs and other pathways such as MAPK. In FHH1 the altered CaSR on the parathyroid gland does not respond appropriately to increasing serum Ca 2+ concentrations so that higher serum Ca 2+ concentrations are required to suppress PTH. This results in a shift of the set point for PTH release to the right. The CaSR mutation can also impair renal Ca 2+ excretion, thereby contributing to the hypocalciuria. In the kidney, activation of CaSR increases Ca 2+ excretion, acidifies urinary pH, and impairs urinary concentration in an attempt to reduce the risk of nephrocalcinosis and nephrolithiasis. In addition, in an attempt to maintain solubility of urinary Ca 2+ salts and to prevent tubular Ca 2+ precipitation, CaSR activation at the apical membrane of the collecting duct enhances acid secretion by stimulation of H + -ATPase and inhibits water absorption by interference with vasopressin-induced aquaporin-2 (AQP2) insertion in the apical membrane. Chronic hypercalcemia results in diabetes insipidus by AQP2 downregulation via the CaSR.
In the context of hypophosphatemia, hypocalciuria helps to distinguish FHH versus hyperparathyroidsim (which causes hypercalciuria). Approximately 65% of FHH patients have CaSR mutations. The opposite pathophysiology can be seen with autosomal dominant hypocalcemia (see later). Gain-of-function mutations of the CaSR, which induce a higher sensitivity to extracellular Ca 2+ , can also result in Bartter syndrome. As these mutations imitate elevated extracellular Ca 2+ concentrations, PTH secretion is suppressed and impairs renal Ca 2+ and Mg 2+ absorption.
Over the following years, FHH was found to be a genetically heterogeneous condition. Loss-of-function mutations in Gα 11 , which encodes a protein involved in the signaling cascade of the CaSR, were found in FHH2 patients. Because Gα 11 is part of the CaSR signaling cascade, it was a candidate gene for FHH2. In vitro experiments confirmed that loss-of-function mutations in the encoding gene GNA11 decreased the sensitivity of the CaSR to extracellular Ca 2+ and resulted in a rightward shift in the concentration-response curve, with significantly higher half-maximal effective concentration (EC50) values. Interestingly, gain-of-function mutations in the genes encoding Gα 11 result in the opposite phenotype of autosomal dominant hypocalcemia (see later).
In FHH3, clinical characteristics can be different with elevated PTH levels, hypophosphatemia, and osteomalacia. FHH3 is caused by dominant mutations in the adaptor protein-2 sigma subunit (AP2σ1, encoded by the AP2S1 gene), which results in loss of function of the encoded protein, which is important as a component of clathrin-coated vesicles. AP2S1 mutations decrease the sensitivity of CaSR-expressing cells to extracellular Ca 2+ and impair CaSR endocytosis by reducing the affinity for the dileucine motif. Mutations were identified in 20% of FHH patients without CASR mutations. Overall the disease severity in FHH is relatively mild, and some patients remain asymptomatic. Sporadic CaSR mutations are also possible. FHH patients are usually treated with thiazides or calcimimetics. Parathyroidectomy is contraindicated.
A more pronounced phenotype than FHH is associated with neonatal severe hyperparathyroidism (NSHPT), which is due to homozygous or compound heterozygous, inactivating mutations in the CaSR gene. Symptoms include severe hypercalcemia, markedly elevated PTH levels, failure to thrive, polyuria, hypotonia, and hypophosphatemia. Infants with NSHPT often have pronounced bone disease. NSHPT patients are treated with rehydration, bisphosphonates, and calcimimetics. Patients who are not responding to medical treatment may benefit from parathyroidectomy.
Jansen Metaphyseal Chondrodysplasia
This rare, autosomal dominant condition is characterized by severe hypercalcemia, hypophosphatemia, abnormal chondrocyte proliferation, and normal or undetectable PTH or PTH-related peptide levels. Affected patients display short stature and short extremities. This condition is caused by heterozygous mutations of the PTH receptor result in constitutive activation of the receptor. These PTH receptor mutations resulted in agonist-independent accumulation of cyclic adenosine monophosphate (cAMP), a secondary messenger of the PTH receptor. Some of the identified PTH receptor mutations yielded milder skeletal phenotypes and overall normal stature but hypercalcemia and a higher risk for hypercalciuria resulting in a higher risk of nephrolithiasis. Bisphosphonates may be able to improve this condition.
PTH-Independent Hypercalcemia
Severe Infantile Hypophosphatasia
Hypercalcemia, hypercalciuria, paradoxically low alkaline phosphatase levels, failure to thrive, poor feeding, increased intracranial pressure, papilledema, and nephrocalcinosis are found in a rare autosomal recessive condition named infantile hypophosphatasia. In addition, loss of deciduous teeth is described in affected children. Symptoms can present perinatally, in infancy, in childhood, or in adolescence, spanning a surprisingly large clinical variability ranging from intrauterine death to unmineralized bone and dental complications. The variability is due to autosomal dominant versus recessive transmission of the gene defect with two affected alleles causing the more severe and earlier phenotypes. This disorder is due to mutations in the gene ALPL encoding tissue-nonspecific isoenzyme alkaline phosphatase. As a result, alkaline phosphatase activity is impaired in the liver, kidney, teeth, and bone. The encoded protein is a cell surface phosphohydrolase. When deficient, there is accumulation of the substrates for alkaline phosphatase, including inorganic pyrophosphate (alkaline phosphatase is a pyrophosphatase), a very portent inhibitor of mineralization, thereby explaining the dental and osseous phenotype. In the infantile form of hypophosphatasia, symptoms present prior to 6 months of age. Hypercalcemia and hyperphosphatemia with severe rickets are seen due to the inability of the patient’s blocked entry of minerals into the skeleton with appropriately reduced PTH concentrations. Infants may also present with pyridoxine-dependent seizures due to incomplete extracellular hydrolysis of pyridoxal 5′-phosphate, the major circulating form of vitamin B6. More than 300 ALPL mutations have been published. In the infantile form, approximately 50% of affected infants die. A lethal outcome is likely in the context of pyridoxine-dependent seizures. Diagnosis is made by elevated levels of phosphoethanolamine (serum and urine), inorganic pyrophosphate (serum and urine), and pyridoxal 5′-phosphate (serum). Radiographic signs for the infantile form include lack of mineralization, gracile ribs, poorly calcified metaphysis, fractures, progressive demineralization, and premature bony fusion. Treatment for these patients has improved with asfotase alfa, a bone-targeted recombinant enzyme replacement therapy.
Idiopathic Infantile Hypercalcemia (Lightwood Syndrome)
Idiopathic infantile hypercalcemia (IIH) occurs typically in the first year of life, with a peak between 5 and 8 months. Patients may develop failure to thrive, vomiting, hypercalciuria, and dehydration. Overall the prognosis is good even though many patients may develop nephrocalcinosis. No syndromic features were discovered. In some instances, IIH resolves spontaneously. Typically, PTH levels are suppressed, and there are upper limits of normal to elevated 25(OH) 2 D and 1,25 Vitamin D levels. In the past, excessive vitamin D intake, increased sensitivity to vitamin D, and increased PTHrP secretion were thought to be involved in the pathogenesis. In 2011 Schlingmann and coworkers published recessive mutations in the CYP24A1 gene, which encodes the 25-hydroxyvitamin D 24-hydroxylase as the main cause for IIH. Mutations were found in IIH patients and a second cohort of patients who displayed symptoms of vitamin D intoxication soon after receiving high-dose vitamin D prophylaxis. The encoded enzyme converts 1,25 Vitamin D to an inactive metabolite. The identified mutations were found to abolish 1,25 Vitamin D catabolism almost completely in vitro , resulting in higher levels of 1,25 Vitamin D and subsequently hypercalcemia. Patients with CYP24A1 mutations developed severe hypercalcemia when they received vitamin D prophylaxis. Given the routine use of cholecalciferol supplementation in children, medical professionals should be aware of this condition causing this rare side effect of vitamin D supplementation. Interestingly, adult patients with nephrolithiasis and hypercalcemia were also found to have CYP24A1 mutations.
A subgroup of IIH patients did not have CYP24A1 mutations but was characterized by pronounced hypophosphatemia and renal phosphate wasting, in addition to hypercalcemia, suppressed PTH, and nephrocalcinosis. Interestingly, all patients also received vitamin D prophylaxis. Genome-wide linkage analysis pointed to SLC34A1, which encodes the renal sodium–phosphate cotransporter 2a (NaPi2a), and recessive mutations in 16 patients with IIH were identified. This cotransporter is responsible for combined sodium and phosphate absorption from the proximal tubule, and affected patients demonstrated urinary phosphate wasting in addition to symptomatic hypercalcemia in infancy. Functional studies of mutant NaPi2a showed impaired trafficking of the cotransporter to the plasma membrane and thereby resulted in less phosphate transport activity.
One may wonder how renal phosphate wasting results in hypercalcemia and nephrocalcinosis. The biologic activity of 1,25 Vitamin D is regulated by its activating enzyme 1α-hydroxylase (CYP27B1) and its deactivating enzyme 24-hydroxylase (CYP24A1). Both enzymes are regulated by 1,25 Vitamin D itself, phosphate, Ca 2+ , PTH, and the fibroblast factor 23 (FGF23), a phosphatonin. FGF23 inhibits 1α-hydroxylase and promotes 1,25 Vitamin D degradation by enhancing 24-hydroxylase. A key role in the pathomechanism of this specific subgroup is the downregulation of FGF23 in the context of hypophosphatemia and hyperphosphaturia. The hypophosphatemia results in more active 1,25 Vitamin D synthesis and less 1,25 Vitamin D catabolism, thereby increasing the 1,25 Vitamin D level, serum Ca 2+ concentration, and hypercalciuria. As a proof of concept, treatment with furosemide, rehydration, corticosteroids, or ketoconazole or a low calcium diet does not improve the patient’s hypercalcemia. Phosphate supplementation improves the hypercalcemia in affected patients.
Williams-Beuren Syndrome
Williams-Beuren syndrome (WBS) is characterized by elfin facies, supravalvular aortic stenosis, peripheral pulmonary stenosis, motor disabilities, dental abnormalities, developmental delay, and a friendly personality. Hypercalcemia and hypercalciuria can occur with WBS and can result in severe nephrocalcinosis. Additional endocrine disorders can include diabetes mellitus (DM), mild hypothyroidism, hyperparathyroidism, and impaired bone metabolism, and approximately 20% of patients may have congenital anomalies of the kidney and urogenital tract (CAKUT). Approximately 90% of WBS patients carry a microdeletion of chromosome 7, which includes the elastin gene, causing a hemizygous continuous gene deletion syndrome of 25 to 30 genes.
Bartter Syndrome
Bartter syndrome is characterized by salt wasting, polyuria, hypokalemia, metabolic alkalosis, massive polyuria, which can result in life-threatening dehydration, hyperreninemic hyperaldosteronism, polyhydramnios, failure to thrive, hypercalciuria, nephrocalcinosis, osteopenia, and almost always prematurity. The development of these features can take weeks to months. The causes for antenatal Bartter syndrome include recessive mutations of the Na + -K + -2Cl − -cotransporter NKCC2 and the renal outer medullary potassium channel ROMK. Mutations of the gene encoding ROMK frequently result in postnatal hyperkalemia in contrast to all other genes contributing to Bartter syndrome. In the TAL CaSR is expressed at the basolateral membrane and impairs paracellular and transcellular NaCl and divalent cation reabsorption by inhibiting ROMK and NKCC2 ( Fig. 20.3 ). This results in a decrease of the lumen-positive potential and reduces the driving force for paracellular Na + , Ca 2+ , and Mg 2+ reabsorption and causes urinary wasting of these electrolytes.

A less common cause for antenatal Bartter syndrome are mutations of Barttin, which is a subunit of the basolateral chloride channel CLCNKB. In addition to the symptoms described previously, these patients also have sensorineural deafness. Recessive mutations in CLCNKB cause classical Bartter syndrome. Hypercalciuria, hyperparathyroidism, and nephrocalcinosis are detected with NKCC2 mutations. In rare instances, hypercalcemia is described. Transient hypercalciuria and nephrolcalcinosis were also noticed with MAGED2 mutations, a novel cause for antenatal Bartter syndrome. Bartter syndrome is discussed more in detail in a different chapter.
Hypocalcemia
Neonatal hypocalcemia is defined as total serum Ca 2+ concentrations less than 7 mg/dL (<1.75 mmol/L) and for ionized Ca 2+ less than 4.4 mg/dL (1.1 mmol/L) compared with adult hypocalcemia with Ca 2+ concentrations less than 8.4 mg/dL (2.1 mmol/L). After delivery the maternal Ca 2+ supply is interrupted and the main source of Ca 2+ is the bone. Due to the low dietary Ca 2+ intake on the first day of life, the nadir (4.4–5.4 mg/dL, 1.1–1.36 mmol/L) in full-term neonates is reached within 24 hours postdelivery and gradually increases thereafter. Approximately 30% of preterm infants will encounter hypocalcemia within the first 48 hours. Serum Ca 2+ concentrations correlate with gestational age. Therefore premature infants have a higher likelihood of developing hypocalcemia. Low serum albumin levels are a common contributing factor for hypocalcemia, but ionized Ca 2+ concentrations should remain normal.
Symptoms of Hypocalcemia
Symptoms of hypocalcemia may be mild but can manifest as neonatal jitteriness and convulsions (generalized and focal). Because hypocalcemia and hypomagnesemia may present in a similar fashion, both electrolytes should be measured. A chest x-ray may help to determine the thymic silhouette in case of suspicion for DiGeorge syndrome. An ECG may reveal increased corrected QT interval longer than 0.4 seconds.
A list of inherited causes of hypocalcemia is provided in Tables 20.3–20.5 .
Disorder | OMIM | Inheritance | Gene Locus | 1,25 (OH) 2 Vitamin D | PTH | Serum Ca 2+ | Urine Ca 2+ | Gene (Protein) | Function | References |
---|---|---|---|---|---|---|---|---|---|---|
Congenital hypoparathyroidism | #146200 | AD | 11p15.3 | ↓ | ↓ | ↓ | ↓ | PTH (PreproPTH) | PTH hormone | |
Hypoparathyroidism, deafness, and renal anomalies (HDR) | #146255 | AD | 10p14 | ↓ | ↓ | ↓ | ↓ | GATA3 (GATA3) | Transcription factor | |
DiGeorge syndrome | #188400 | AD | 22q11.2 | ↓- n | ↓ | ↓ | ↓ | Heterogenous gene deletion syndrome/ TBX1 | TBX1 encodes transcription factor | |
Parathyroid gland agenesis | #146200 | AD/AR | 6p24.2 | ↓ | ↓ | ↓ | ↓ | GCMB/GCM2 (GCMB/GCM2) | Transcription factor | |
Autoimmune polyendocrine syndrome | #240300 | AR | 21q22.3 | ↓ | ↓ | ↓ | ↓ | AIRE (AIRE) | Nuclear transcription factor | |
Kearns-Sayre syndrome | #530000 | mito. | mito. | ↓ | ↓ | ↓ | ↓ | MTTL1 (MTTL1) | tRNA for leucine | |
MELAS syndrome | #540000 | mito. | mito. | ↓ | ↓ | ↓ | ↓ | MTTL1, MTTQ, … (MTTL1, MTTQ) | tRNA for leucine, glutamine, … | |
Kenney-Caffey syndrome | #244460 | AR | 1q42.3 | ↓ | ↓ | ↓ | ↓ | TBCE (TBCE) | Tubulin-specific chaperone | |
Blomstrand disease | #215045 | AR | 3p21.31 | ↓ | n -↑ | ↓ | ↓ | PTHR1 (PTH receptor) | PTH receptor |
Disorder | OMIM | Inheritance | Gene Locus | 1,25 (OH) 2 Vitamin D | PTH | Serum Ca 2+ | Urine Ca 2+ | Gene (Protein) | Function | References |
---|---|---|---|---|---|---|---|---|---|---|
Autosomal dominant hypocalcemia | #601198 | AD | 3q13.3-q21.1 | ↓ | n -↓ | ↓ | ↑ | CASR (CaSR) (gain-of-function) | Ca 2+ -sensing receptor | |
Autosomal dominant hypocalcemia | #615361 | AD | 19p13.3 | ↓ | n -↓ | ↓ | ↑ | GNA11 (Gα 11 ) (gain-of-function) | G protein signaling | |
Pseudohypoparathyroidism—type Ia | #103580 | AD | 20q13.32 | ↓ | ↑ | ↓ | n.d. | GNAS1 (Gα S ) | G protein signaling | |
Pseudohypoparathyroidism—type Ib | #603233 | AD | 20q13.32 | ↓ | ↑ | ↓ | n.d. | STX16 (Syntaxin 16) | Endosome-trans-Golgi-network | |
Pseudohypoparathyroidism—type II | %203330 | AD | n.d. | ↑ | ↓ | n.d. | n.d. | |||
Pseudopseudohypoparathyroidism | #612463 | AD | 20q13.32 | ↓ | ↑ | ↓ | n.d. | GNAS1 (Gα S ) | G protein signaling |
Disorder | OMIM | Inheritance | Gene Locus | 1,25 (OH) 2 Vitamin D | PTH | Serum Ca 2+ | Urine Ca 2+ | Gene (Protein) | Function | References |
---|---|---|---|---|---|---|---|---|---|---|
Vitamin D-dependent rickets type 1 | #264700 | AR | 12q14.1 | ↓ | ↑ | ↓ | n -↓ | CYP27B1 (25-(OH) vitamin D3-1-α-hydroxylase) | Enzyme | |
Vitamin D-dependent rickets type 2 | #277440 | AR | 12q13.11 | ↑ | ↑ | ↓ | n -↓ | VDR (vitamin D receptor) | Receptor |
Hypocalcemia Due to PTH Abnormalities/Hypoparathyroidism
Congenital Hypoparathyroidism.
Familial isolated hypoparathyroidism can be inherited as an autosomal recessive, dominant, or X-linked trait or it may occur sporadically. Mutations occur in genes regulating parathyroid gland development or in the PTH gene (in the PTH precursor preproPTH). Patients present with hypocalcemia, hyperphosphatemia, inappropriately low PTH, and low 1,25 Vitamin D concentrations. Due to the lack of renal PTH action, tubular absorption of Ca 2+ is diminished, resulting in hypercalciuria and a higher risk for nephrocalcinosis and chronic kidney disease (CKD). Interestingly, CASR gene mutations have also been found to result in hypoparathyroidism in addition to causing autosomal dominant hypocalcemia (see later).
Hypoparathyroidism, Deafness, and Renal Anomalies Syndrome.
Hypoparathyroidism, deafness, and renal anomalies (HDR) syndrome is characterized by congenital hypoparathyroidism, bilateral sensorineural deafness, and renal dysplasia/cysts. HDR patients present with hypocalcemia and low to undetectable PTH concentrations. HDR syndrome is caused by heterozygous mutations in the transcription factor GATA3 on chromosome 10p14. GATA3 expression during human and murine development (e.g., in otic vesicle, parathyroid glands, kidneys) is consistent with the HDR phenotype.
DiGeorge Syndrome.
Hypoparathyroidism can be part of DiGeorge syndrome, which is characterized by branchial cleft abnormalities including hypoplasia of pharyngeal arches, parathyroid glands, thymus, cardiac and craniofacial defects. This syndrome is due to chromosomal deletions of 22q11.2, which includes approximately 30 genes. One of these genes is the TBX1 gene, and mice lacking Tbx1 and humans with TBX1 point mutations develop DiGeorge syndrome. TBX1 encodes a transcription factor of the T-box family and is crucial in organogenesis. Another form of DiGeorge syndrome is due to a deletion of 10p. Approximately 17% of DiGeorge patients have no detectable chromosomal abnormality. In approximately 50% to 60% of DiGeorge patients, hypocalcemia is diagnosed. Transient hypocalcemia at birth can be seen due to abrupt discontinuation of maternal Ca 2+ supply and the delivery stress. Patients are treated with vitamin D and Ca 2+ to keep serum Ca 2+ concentrations in the low normal to avoid nephrocalcinosis and nephrolithiasis.
GCMB Mutations.
Isolated parathyroid gland agenesis was published due to heterozygous (dominant negative) or recessive (loss-of-function) mutations in the glial cell missing B ( GCMB or GCM2 ) gene, which encodes for a parathyroid specific transcription factor and contributes to regulation of PTH gene expression.
Autoimmune Polyendocrine Syndrome.
Another syndrome that includes hypoparathyroidism is autoimmune polyendocrine syndrome type 1 (APS1), which is caused by autosomal recessive mutations in the autoimmune regulator gene (AIRE). APS1 is characterized by multiple endocrine gland insufficiencies (e.g., hypoparathyroidism, adrenal insufficiency, hypogonadism, DM, hypothyroidism) and chronic mucocutaneous candidiasis in childhood. This gene encodes a nuclear transcription factor which eliminates self-reactive T cells in the thymus. As a consequence the immune system fails to establish immunologic tolerance.
Mitochondrial Disorders Associated With Hypoparathyrodism.
Three mitochondrial disorders are associated with hypoparathyroidism, including Kearns-Sayre syndrome (KSS), mitochondrial encephalopathy, lactic acidosis, and stroke-like episodes syndrome (MELAS), and mitochondrial trifunctional protein deficiency syndrome.
Other Syndromes (e.g., Kenney-Caffey and Blomstrand Disease).
In approximately 50% of patients with Kenney-Caffey syndrome (KCS), hypoparathyroidism has been described. Genetic heterogeneity with mutations in the genes encoding tubulin-specific chaperone (TBCE) and family with sequence similarity 111A (FAM111A) were described.
Another rare, autosomal recessive cause for hypocalcemia is Blomstrand chondrodysplasia. This condition is characterized by accelerated bone maturation, advanced chondrocyte differentiation, hyperdensity of the skeleton, accelerated ossification, and early lethality. The causes for this disease are mutations of the PTH receptor impairing its binding of PTH, function, or expression.
Treatment for these conditions include Ca 2+ and 1,25 Vitamin D supplements, which may be complicated by developing hypercalciuria and nephrocalcinosis. Thiazide diuretics may be able to improve hypercalciuria. Recombinant PTH (1–84) and (1–34) have been successfully used to improve successfully Ca 2+ homeostasis. An orally active small molecule PTHR1 agonist called PCO371 was discovered as potential therapeutic option.
Hypocalcemia Due to CaSR Abnormalities or Downstream Signaling
Autosomal Dominant (Hypercalciuric) Hypocalcemia.
In autosomal dominant hypocalcemia type 1, approximately 50% of patients remain asymptomatic or have mild hypocalcemia. Hypocalcemia may be present in the neonatal period. The other 50% can develop seizures, paresthesia, and carpopedal spasms. Although overall rare, this condition may be responsible for up to a third of patients with idiopathic hypoparathyroidism. Approximately 10% have hypercalciuria with nephrocalcinosis and kidney stones. Patients with autosomal dominant hypocalcemia often present with hypomagnesemia and hypermagnesuria. Approximately one-third of patients have ectopic and basal ganglia calcifications. PTH concentration may be normal or inappropriately low. As a cause for autosomal dominant hypocalcemia type 1, gain-of-function mutations in the CaSR were identified resulting in higher serum Ca 2+ threshold for PTH release (see Fig. 20.3A ). This imitates higher extracellular calcium concentrations, and as a result PTH secretion is suppressed and renal Ca 2+ and Mg 2+ absorption is impaired. Some patients may present with hypokalemia and alkalosis, symptoms consistent with Bartter syndrome, which is thought to be due to the CaSR effect on sodium absorption in the TAL. The Bartter syndrome phenotype is thought to be due to impaired NKCC2 and ROMK function induced by an inhibitable adenyl cyclase, thus reducing Na + , K + , and Cl − reabsorption in the TAL (see Fig. 20.3A ).
In a cohort of autosomal dominant hypocalcemia patients without hypercalciuria, heterozygous gain-of-function mutations of GNA11 were identified, also resulting in an increased sensitivity of CaSR-expressing cells to extracellular Ca 2+ , thereby causing a leftward shift in the concentration-response curves. GNA11 encodes a G protein that connects CaSR sensing with the Ca 2+ /IP3/PKC signaling pathway in the parathyroid gland. Differentiation between autosomal dominant hypocalcemia and hypoparathyroidism is important because treatment of autosomal dominant hypocalcemia patients with calcium and vitamin D may aggravate hypercalciuria and may worsen nephrocalcinosis.
Pseudohypoparathyroidism
Pseudohypoparathyroidism (PHP) is due to a partial or complete peripheral resistance of the biologic actions of PTH. PHP is a heterogeneous syndrome characterized by increased PTH concentrations but functional hypoparathyroidism. Biochemical features of PHP are similar to hypoparathyroidism with hypocalcemia, hyperphosphatemia, and low 1,25 Vitamin D concentrations, but PHP patients have an elevated PTH level. PHP type 1a (PHP-Ia) is also called Albright hereditary osteodystrophy and is characterized by short stature, round facies, barrel chest, obesity and brachydactyly, shortening of the fourth and fifth metacarpals, and mild developmental delay. This disorder is due to autosomal dominant inheritance of mutations in the GNAS1 gene, which encodes the alpha subunit of the stimulatory G protein (Gα s ). Other forms of PHP include PHP-Ib and pseudo-PHP.
Heterozygous loss-of-function mutations in the GNAS1 gene, leading to a 50% reduction of Gα s activity, cause PHP-Ia. However, the GNAS1 gene is an imprinted gene and the clinical presentation depends on whether the mutant allele is inherited from the mother or the father. In case the mutation is inherited from the mother the patient will present with PHP, whereas the patient will present with pseudo-PHP when the mutation is inherited from the father. Pseudo-PHP is characterized by the same physical characteristics as patients with PHP-Ia but lacks the endocrine abnormalities and also frequently does not show obesity. In case of a maternally inherited mutation, only the paternal GNAS allele is available. Due to silencing/imprinting of the paternal GNAS allele in multiple tissues, including the proximal tubule, this results effectively in a complete GNAS knockout. These patients present with PHP-Ia or Albright hereditary osteodystrophy and do not respond appropriately with an increase of urinary cAMP and PO 4 − excretion after PTH exposure due to resistance of the proximal tubule to PTH. Because Gα s and cAMP are crucial in the downstream signaling of other peptide hormones, patients may develop resistance to hormones such as luteinizing hormone, follicle stimulating hormone, and thyroid-stimulating hormone (TSH). In pseudo-PHP the patient inherits the GNAS mutation from the paternal allele. Because the paternal mutant GNAS gene will be silenced due to imprinting, many adult tissues will be left with one functional copy of GNAS. Therefore these patients do not develop PTH or other hormone resistance and are characterized by normal serum Ca 2+ and phosphate concentrations.
Patients with PHP-Ib do not develop the phenotype of Albright hereditary osteodystrophy but still exhibit PTH resistance limited to the kidney. PHP-Ib patients have a higher risk of TSH resistance and shortening of the fourth and fifth metacarpals. These patients have mutations in regulatory elements of the GNAS gene which alter the control of paternal DNA methylation and its imprinting in the kidney. Despite the limitation of PTH resistance to the kidney and laboratory tests indicating hypoparathyroidism, these patients’ bones still respond to PTH and are therefore exposed to the skeletal complications of chronic PTH exposure, such as osteoporosis or osteitis fibrosa cystica.
Another cohort of patients with hypoparathyroidism and inappropriate renal phosphaturic response to PTH but normal renal cAMP and Gα s activity after PTH exposure is defined as pseudo-PHP type II. This condition is thought to be due to intracellular resistance to cAMP, possibly a defective cAMP-dependent kinase. The different forms of PHP can be classified according to the renal and systemic response to PTH exposure (e.g., Ellsworth-Howard test).
Hypocalcemia Due to Disturbed Vitamin D Metabolism
Inherited forms of low vitamin D include vitamin D synthesis and the vitamin D receptor. Impaired 1α hydroxylation of 25(OH) vitamin D is due to recessive loss-of-function mutations in the CYP27B1 gene, resulting in vitamin D–dependent rickets type 1. These patients are characterized by hypocalcemia, hypophosphatemia, normal or elevated 25(OH)D, and very low 1,25 Vitamin D concentrations and elevated PTH levels. Infants present with rickets, osteomalacia, and seizures and respond well to treatment with 1,25 Vitamin D. A more common cause for impaired 1α hydroxylation of 25(OH) vitamin D is CKD.
Vitamin D–dependent rickets type 2 is characterized by resistance of the tissues to the biologic effects of calcitriol caused by recessive, inactivating mutations in the VDR gene. The biochemical profile is very similar to patients with vitamin D–dependent rickets type 1 (e.g., hypocalcemia, hypophosphatemia, hyperparathyroidism), but in type 2 patients, elevated concentrations of 1,25 Vitamin D are detected. Infants present with rickets and seizures. Some patients also develop alopecia. Patients are treated with large doses of 1,25 Vitamin D to overcome the vitamin D receptor resistance and may also need Ca 2+ infusions.
Inherited Disorders of Magnesium
Introduction
Magnesium (Mg 2+ ) is the second most abundant intracellular cation but is frequently overlooked. Mg 2+ plays important roles in human physiology by acting as a cofactor for more than 600 enzymes, participating in cardiac contractility and nerve conduction, providing stability against DNA and RNA damage via oxidative stress, controlling cell cycle and cell proliferation, and finally ATP has to bind Mg 2+ to be biologically active. An adult body contains a total of 27 g Mg 2+ , whereas a term newborn has approximately 0.8 g. Similar to Ca 2+ the vast majority (80%) of Mg 2+ is accrued in the third trimester. Mg 2+ is stored in three compartments: (1) the bone (65% of total body Mg 2+ ), (2) intracellular (34%), and (3) in the extracellular fluid (ECF) (1%). The role of Mg 2+ in bone is not well understood, and the significance of Mg 2+ for bone is frequently underestimated. Infants with extended fetal Mg 2+ exposure from maternal treatment for tocolysis can develop congenital rickets. Fetal Mg 2+ toxicity leads to impaired bone mineralization and may cause fractures. On the other hand, Mg 2+ seems to be involved in bone metabolism by inducing osteoblast proliferation.
Due to the small Mg 2+ content in ECF, plasma Mg 2+ concentration may not provide the most reliable assessment of total body Mg 2+ content. Mg 2+ is present as the free ion (55%), bound to protein (35%), and complexed by different anions (e.g., oxalate, phosphate) (15%). Serum Mg 2+ is controlled within tight limits (1.5–2.8 mg/dL or 0.62–1.16 mmol/L) and is the same for neonates, infants, children, and adults. Preterm and term neonates have similar cord serum Mg 2+ concentrations. After delivery, both display an initial decrease in serum Mg 2+ which reaches a nadir at 48 hours with 1.87 mg/dL (0.77 mmol/L), after which serum Mg 2+ increases in the first week of life. Dependent on the accompanying disease process, serum Mg 2+ may vary: increased Mg 2+ concentrations are found with hyperbilirubinemia, acidosis, and preterm respiratory distress syndrome.
Postdelivery, blood Mg 2+ concentration reflects the balance between intestinal and renal Mg 2+ excretion. Intestinal Mg 2+ absorption occurs in the small intestine, cecum, and colon and ranges between 25% to 80%, depending on the total body Mg 2+ content. Intestinal Mg 2+ absorption is higher in low Mg 2+ states. In the small intestine, Mg 2+ is absorbed in a paracellular fashion (in between epithelial cells), which is nonsaturable. In the cecum and colon, Mg 2+ is absorbed in a transcellular, saturable fashion via apical Mg 2+ channels transient receptor potential melastatin 6 (TRPM6) and TRPM7 in epithelial cells. Very little is known about Mg 2+ handling in epithelial cells. A basolateral Mg 2+ ATPase and/or Mg 2+ -Na + exchanger has been hypothesized to facilitate basolateral Mg 2+ extrusion. SLC41A3 encodes a basolateral Mg 2+ -Na + exchanger in the distal convoluted tubule (DCT) and was published as a component for Mg 2+ extrusion ( Fig. 20.4A ).

In the kidney, approximately 80% of the total body Mg 2+ is filtered by the glomerulus and 95% to 99% of the filtered Mg 2+ is absorbed along the nephron. Finally, only approximately 100 mg of Mg 2+ is excreted in urine per day. Although the proximal tubule plays a key role for tubular absorption of most electrolytes such as potassium, sodium, and phosphorus, it is responsible for only 10% to 25% of renal Mg 2+ absorption. In contrast, the vast majority of Mg 2+ is absorbed in the TAL (65%–75%). Mg 2+ transport in the TAL occurs in a paracellular fashion, and the lumen-positive transepithelial potential difference is the main driving force. Fine-tuning of renal Mg 2+ absorption takes place in the DCT, where 10% of Mg 2+ is reabsorbed. Here, Mg 2+ is transported in a transcellular fashion via the epithelial, apical Mg 2+ channels TRPM6 and TRPM7 (see Fig. 20.4A ).
Regulators of Magnesium Homeostasis
Similar to Ca 2+ , Mg 2+ regulation is dependent on bone, intestine, and kidneys. The kidneys have the most intricate hormonal regulation for Mg 2+ homeostasis. In particular, transcellular Mg 2+ absorption in the DCT via TRPM6 is influenced by a number of hormones, including epidermal growth factor (EGF), insulin, and estrogen.
Epidermal Growth Factor
EGF was identified as the first magnesiotropic hormone in Dutch sisters with isolated recessive hypomagnesemia (IRH) (see later under “Isolated Recessive Hypomagnesemia”).
Insulin
Hypomagnesemia has been associated with type 2 DM (T2DM) and gestational DM. Insulin phosphorylates at least two specific sites in the Mg 2+ channel TRPM6 which are also known to associate with a higher risk of DM. Insulin increases TRPM6 activity by phosphoinositide 3-kinase and Rac1 signaling, thereby enhancing TRPM6 cell surface abundance.
Estrogen
Because estrogen therapy in postmenopausal women improved hypermagnesuria, estrogen has also been discussed as a magnesiotropic hormone. Renal Trpm6 mRNA level was decreased in ovariectomized rats. This effect was reversed by 17β-estradiol. This is consistent with estrogen enhancing Trpm6 transcription or mRNA stabilization.
Other Hormone Interactions Involving Mg 2+ Homeostasis
Overall, many of the same hormones regulating Ca 2+ homeostasis are also involved in maintenance of Mg 2+ concentration. For example, changes in Mg 2+ affect PTH secretion similar to Ca 2+ , with Mg 2+ being less potent compared with Ca 2+ . Although short-term decrease in Mg 2+ increases PTH secretion, chronic hypomagnesemia abolishes PTH secretion significantly, subsequently causing hypocalcemia. The latter effect is caused by alterations of the Ca 2+ -sensitive, Mg 2+ -dependent adenylate cyclase system, which is crucial for the secretion of PTH. Thus Mg 2+ deficiency may cause or aggravate hypocalcemia by blunting the PTH response to lower serum Ca 2+ concentrations. The effect of calcitonin is thought to be minimal, but there are some indications that calcitonin is involved in fetal Mg 2+ homeostasis because knockout mice lacking calcitonin displayed total body Mg 2+ depletion and lower serum Mg 2+ concentrations. Moreover, calcitonin has been shown to enhance Mg 2+ absorption in the DCT. The effect of vitamin D seems minor and appears to be indirect, but pharmacologic dosages of vitamin D enhance intestinal Mg 2+ absorption. However, there is no correlation between serum Mg 2+ concentration and plasma 1,25 Vitamin D. Interestingly, Mg 2+ is an essential cofactor of the 1,25-hydroxylation reaction in the renal tubular cells to form 1,25 Vitamin D, presumably due to its role as a cofactor for 1α-hydroxylase in the renal tubular cells. Mg 2+ deficiency increases FGF23 concentration and enhances renal 24,25-hydroxylase. Other hormones known to have a mild to modest influence on Mg 2+ homeostasis are thyroxine, aldosterone (both reduce Mg 2+ absorption), epinephrine, glucocorticosteroids, and progesterone.
Hypermagnesemia
Symptoms of Hypermagnesemia
Symptoms include muscle weakness, fatigue, and somnolence. Hypermagnesemia usually occurs in preeclamptic women and their offspring after Mg 2+ therapy and in patients with end-stage renal disease (ESRD).
Hypomagnesemia
Symptoms of Hypomagnesemia
Although mild to moderate hypomagnesemia does not cause acute symptoms, more pronounced hypomagnesemia results in muscle spasms, arrhythmia, and seizures.
Hypomagnesemia is more common and can result as a side effect of medications, insufficient Mg 2+ intake, or rare inherited renal defects. Here we will focus on inherited renal disorders causing Mg 2+ abnormalities. These genetic conditions are divided into hypercalciuric, Gitelman-like, and other hypomagnesemias ( Tables 20.6–20.8 ).
Disorder | OMIM | Inheritance | Gene Locus | Serum Mg 2+ | PTH | Serum Ca 2+ | Urine Ca 2+ | Gene (Protein) | Function | References |
---|---|---|---|---|---|---|---|---|---|---|
Familial hypomagnesemia with hypercalciuria and nephrocalcinosis | #248250 | AR | 3q28 | ↓ | n -↑ | ↓ | ↑ | CLDN16 (Claudin-16) | Tight junction proteins | |
Familial hypomagnesemia with hypercalciuria and nephrocalcinosis plus ocular involvement | #248190 | AR | 1p34 | ↓ | n -↑ | ↓ | ↑ | CLDN19 (Claudin-19) | Tight junction proteins | |
Classical Bartter syndrome (type 3) | #607364 | AR | 1p36 | n -↓ | ↑ | ↓ | ↑ | CLCNKB (ClC subunit B) | Basolateral chloride channel | |
Autosomal dominant hypocalcemia/Bartter syndrome (type 5) | #601198 | AD | 3q13 | n -↓ | ↓ | ↓ | ↑ | CASR (CaSR) | Calcium sensing receptor |
Disorder | OMIM | Inheritance | Gene Locus | Serum Mg 2+ | PTH | Serum Ca 2+ | Urine Ca 2+ | Gene (Protein) | Function | References |
---|---|---|---|---|---|---|---|---|---|---|
Gitelman syndrome | #263800 | AR | 16q13 | ↓ | n | n -↓ | ↓ | SLC12A3 (NCC) | Na + -Cl − cotransporter | |
Antenatal Bartter syndrome with sensorineural deafness (type 4) | #602522 | AR | 1p31 | ↓ | n | n | n -↑ | BSND (Barttin) | Subunit of ClC-Ka/b | |
EAST/SeSAME syndrome | #612780 | AR | 1q23 | ↓ | n | n | ↓ | KCNJ10 (Kir4.1) | Apical potassium channel | |
Isolated dominant hypomagnesemia | #154020 | AD | 11q23 | ↓ | n | n | ↓ | FXYD2 (FXYD2) | Na + /K + -ATPase (γ subunit) | |
*176260 | AD | 12p13 | ↓ | n | n | ↓ | KCNA1 (Kv1.1) | Apical potassium channel | ||
HNF1B nephropathy | #137920 | AD | 17q12 | ↓ | n | n | n | HNF1B (HNF1beta) | Transcription factor | |
Hypomagnesemia after transient neonatal hyperphenyl-alaninemia | #264070 | AR | 10q22 | ↓ | n.d. | n.d. | n.d. | PCBD1 (PCBD1) | Tetrahydrobiopterin metabolism |
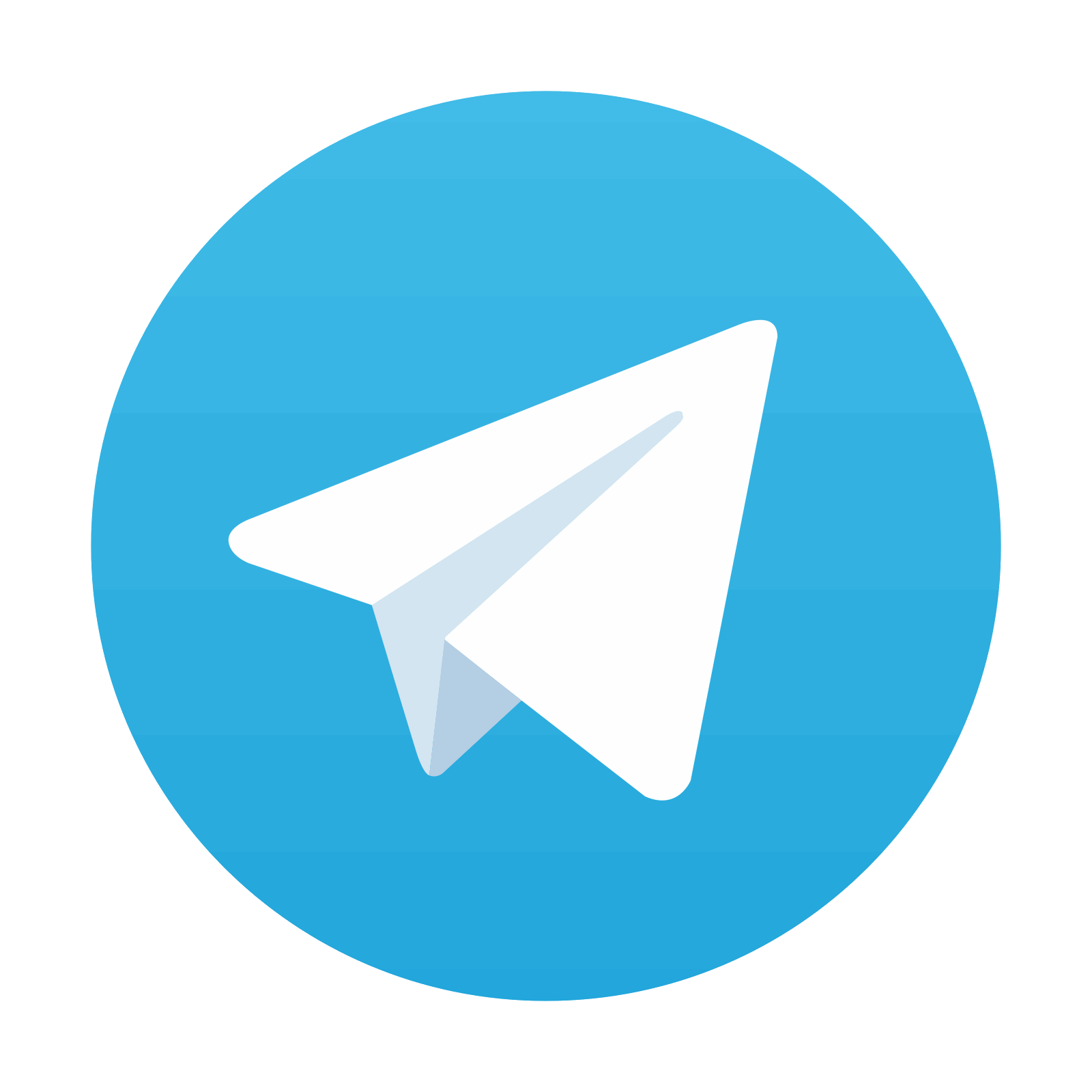
Stay updated, free articles. Join our Telegram channel

Full access? Get Clinical Tree
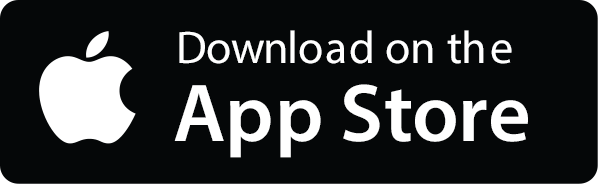
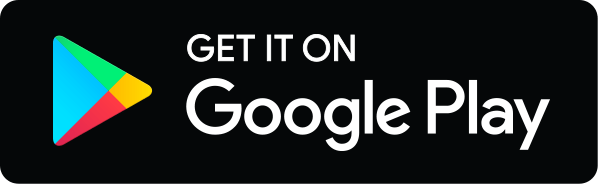
