Abstract
Disturbances in mineral homeostasis, common in newborns, may be caused by altered responses to normal physiologic transition from the intrauterine environment to neonatal independence. Mineral disturbances in newborns, either calcium (Ca) or phosphorus (P), may result from pathologic intrauterine conditions, fetal immaturity, birth stress, inadequate mineral intakes, or genetic defects. Diagnosis requires understanding of unique perinatal, clinical, and biochemical features of newborn mineral metabolism. This chapter reviews Ca and P metabolism during fetal and neonatal periods with emphasis on neonatal transition followed by causes, pathophysiology, and treatment of mineral disturbances.
Keywords
newborn, calcium, phosphorus, hypocalcemia, hypercalcemia, hypophosphatemia, hyperphosphatemia
- •
Body Distribution
- •
Regulation of Serum Calcium and Phosphorous Concentrations
- •
Clinical Disorders Associated With Abnormal Calcium and Phosphorus Homeostasis
Disturbances in mineral homeostasis, common in newborns, may be caused by altered responses to normal physiologic transition from the intrauterine environment to neonatal independence. Mineral disturbances in newborns, either calcium (Ca) or phosphorus (P), may result from pathologic intrauterine conditions, fetal immaturity, birth stress, inadequate mineral intakes, or genetic defects. Diagnosis requires understanding of unique perinatal, clinical, and biochemical features of newborn mineral metabolism. This chapter reviews Ca and P metabolism during fetal and neonatal periods with emphasis on neonatal transition followed by causes, pathophysiology, and treatment of mineral disturbances.
Body Distribution of Calcium and Phosphorous
Large amounts of Ca and P are needed to allow normal mineralization of the skeleton of developing fetuses and neonates. To meet the high mineral requirements of the developing skeleton, fetuses maintain higher blood Ca and P than maternal levels through active transport of Ca and P across placenta against a concentration gradient.
Calcium
At birth, term newborns contain approximately 30 g Ca in total body, 80% of which is accrued during the last trimester of pregnancy at a maximum rate of 150 mg/kg fetal weight/day. Fetal serum Ca (total and ionized) is higher than maternal serum Ca levels, primarily driven by transcellular active transport rather than passive paracellular pathways.
At all ages, 99% of total body Ca is either in bone as hydroxyapatite or as noncrystalline, amorphous Ca phosphate form (predominant form in early life). One percent of total body Ca is in extracellular fluid (ECF) and soft tissues. Ca of mineral phase (at crystal surface) is in equilibrium with ECF; only about 1% is freely exchangeable with ECF. Although this exchangeable pool is a small percentage of skeletal content, it approximates total Ca content in ECF and soft tissues and serves as a Ca reservoir. In children ages 3 to 16 years, total exchangeable Ca pool (TEP) size, by stable isotope technique, correlates with age independent of body weight variations. The bone Ca accretion rate (Vo + ) and Vo + /TEP ratio are greater in children than adults, indicating increased bone flow of Ca in children compared with adults.
Ca concentration in ECF is kept constant by a process that constantly feeds Ca into and withdraws Ca from this fluid compartment. Ca enters the plasma via intestinal absorption and bone resorption. In Ca balance, rates of Ca release from and uptake into bone are equal. In normal adults, total serum Ca ranges from 2.2 to 2.6 mmol/L (8.8–10.4 mg/dL) and is remarkably constant. Ionized Ca, although subject to changes directed by parathyroid hormone (PTH), calcitonin (CT), vitamin D, and blood pH, is also stable within individuals over prolonged periods and ranges from 1.2 to 1.3 mmol/L (4.8–5.2 mg/dL).
At birth, abrupt termination of maternal-to-fetal Ca supply occurs. To maintain serum Ca homeostasis, an increase of 16% to 20% in Ca flux from bone to ECF is required unless sufficient exogenous Ca intake is achieved. In term newborns, cord blood total Ca is 2.6 mmol/L (10.2 mg/dL), and ionized Ca is 1.5 mmol/L (5.8 mg/dL). By 2 hours of age, serum total Ca declines by 5%, and by 24 to 36 hours, serum Ca reaches its nadir of total Ca of 2.3 mmol/L (9.0 mg/dL) and ionized Ca of 1.2 mmol/L (4.9 mg/dL). After a stabilization period, serum Ca slowly rises, reaching levels by 1 week of total Ca of 2.6 mmol/L (10.4 mg/dL) and ionized Ca of 1.4 mmol/L (5.5 mg/dL), similar to levels in childhood ( Fig. 5.1 ).

In preterm infants, mean cord serum ionized Ca is 1.45 mmol/L (95% confidence interval 1.29–1.61 mmol/L [5.8 (5.74–5.86) mg/dL]), which decreases during the first 24 to 36 hours of life and rises at 6 days to values exceeding original cord blood. An early decrease in ionized Ca may be associated with parathyroid glandular unresponsiveness because of prematurity or hypomagnesemia, and severe hypocalcemia may result. Very low birth weight (VLBW) infants are likely to exhibit the lowest nadirs of ionized Ca; however, most are unassociated with tetany or decreased cardiac contractility (see Fig. 5.1 ).
Cord serum Ca differs by season of birth (lower Ca in summer-born vs. winter-born infants) and delivery mode, but is unaffected by gender, race, or weight appropriateness for gestation.
Phosphorus
In term newborns, total body P is approximately 16 g (0.6% of body weight). As with Ca, approximately 80% of P in term newborns is accumulated during the last pregnancy trimester at a rate of 75 mg/kg fetal weight/day, closely linked to Ca accretion, with Ca/P ratio of 1.7:1; 75% of P is for bone mineralization and 25% is in other tissues. Transplacental P transport is an active sodium-dependent process against the concentration gradient.
About 85% of total body P is in bone, primarily as hydroxyapatite and as more loosely complexed amorphous forms of bone crystal. P plays key structural roles in bone. In contrast to Ca, P is widely distributed in nonosseous tissues, as an inorganic form, and as a component of structural macromolecules. Unlike Ca, 15% of total body P is in the ECF, largely as inorganic P ions. Soft tissue P is almost totally P esters. P is taken up from circulation into cells via type II and type III sodium phosphate cotransporters (NPT) to facilitate cellular functions such as DNA and membrane lipid synthesis, generation of high-energy P esters (energy metabolism), and intracellular signaling. Intracellular P esters and phosphorylated intermediates are involved in important biologic processes and bone mineralization. Thus, P deficiency results in muscle weakness, impaired leukocyte function, and abnormal metabolism.
Serum total P is higher in children than adults. Adult normal ranges are 1.24 to 1.86 mmol/L (3.0–4.5 mg/dL). In normal term infants, cord serum P does not differ by gender, race, season of birth, weight appropriateness for gestation, or mode of delivery. P values in term infants range from 2.3 to 3.5 mmol/L (5.6–8.4 mg/dL) and in preterm infants from 1.7 to 3.3 mmol/L (4–8 mg/dL). The relatively low initial birth values, 2.6 mmol/L (1.5–3.4) (6.2 [3.7–8.1] mg/dL), increase shortly after birth, thought to be related to increased gluconeogenesis and endogenous P release or secondary to low glomerular filtration rate (GFR) and reduced P excretion. Mean serum P increases to 3.4 mmol/L (8.1 mg/dL) by 1 week and decreases to 1.7 mmol/L (4.1 mg/dL) in childhood.
Regulation of Calcium Homeostasis
After the first few days of life, serum Ca is maintained at a nearly constant level primarily through interaction of three hormones: PTH, 1,25-dihydroxyvitamin D [1,25(OH) 2 D], and CT; these hormones direct intestinal Ca absorption, renal Ca reabsorption or excretion, and transfer of Ca stores from bone. In the fetal period, both PTH and parathyroid hormone–related peptide (PTHrP) act in regulation of fetal mineral metabolism through possible regulation of placental Ca transfer and maintaining fetal blood Ca.
Placental Transport
Active Ca transport is facilitated by Ca-sensing receptor (CaSR) that “senses” extracellular Ca. Placental CaSRs regulate Ca transport from mother to fetus. Thus, the fetus develops in a “hypercalcemic state” (≈1 mg/dL higher Ca than maternal levels), and PTHrP (midregion fragment) is critical for maintaining this level. Cord PTH is low, presumably suppressed by hypercalcemia in utero. The set point for fetal serum Ca is higher than the maternal set point.
Ca enters placental trophoblast at brush-border membrane (maternal–placental interface) primarily via the transient receptor potential (TRP) channel superfamily, especially the TRPV6 channel (voltage-dependent Ca 2+ channel). The time course of TRPV6 mRNA expression in wild-type fetuses reveals a 14-fold increase during the last trimester of pregnancy, coinciding with a period of maximal Ca transfer. Ca transport within trophoblast cells is facilitated by intracellular Ca-binding proteins (calbindin D9K) and actively transported into fetal circulation at the placental–fetal interface through a Ca pump, plasma membrane Ca 2+ ATPase protein (PMCA3).
Fetal PTH production is very low, especially at the end of gestation, despite a stable serum ionized Ca. In mice with homozygous ablation of transcriptional factor Hox 3, fetal parathyroids are not developed, with undetectable PTH production; serum ionized Ca is significantly lower in the Hox 3 ablated fetus than in maternal serum. Measures of placental Ca transfer are not changed, suggesting that fetal PTH has no effect on transplacental Ca transfer. However, PTH-ablated mice show significant abnormalities in fetal bone formation, including decreased mineralization of cartilage matrix, suggesting an additional role.
In mice with lack of PTHrP, ionized Ca is significantly low in fetuses and similar to maternal levels, suggesting abolition of the maternal–fetal gradient. Transplacental Ca transfer was restored by infusions of PTHrP (1–86) or midregion fragment (67–86), but not by PTHrP (1–34) nor by PTH (1–84). However, in PTHrP-ablated mice, using a different technique to assay placental Ca transfer (in situ artificially perfused placenta), surprisingly there is increased maternal–fetal Ca transfer, presumably related to significantly higher fetal Ca accretion, despite pronounced fetal hypocalcemia and abolition of fetomaternal Ca gradient. Thus, the influence of PTHrP on placental Ca transport is unclear, with data for facilitating and inhibiting effects.
Possible factors explaining this discrepancy in these studies include technical differences in the conduct of experiments as well as modifying genes in the settings of different mouse strains. Additional studies will be required to clarify the role of PTHrP.
Parathyroid Hormone
After birth, PTH is the main regulator of Ca and P homeostasis, acting in two main target tissues, kidney and bone, through the PTH/PTHrP receptor (PTHR1). PTH elevates blood Ca. PTH secretion by parathyroid glands is regulated by circulating Ca ion, sensed by CaSRs in parathyroids. Increased serum Ca inhibits PTH secretion, and decreased serum Ca stimulates PTH secretion. CaSRs play key roles in maintenance of a narrow range (1.2–1.3 mM/L; 4.8–5.2 mg/dL) of extracellular ionized Ca (Ca 2+ ). CaSR is expressed in target tissues for PTH, such as the kidneys, bone, and placenta, to sense alterations in Ca 2+ , responding with changes directed at normalizing blood Ca 2+ . Genetic mutations of the CASR gene, on the long arm of chromosome 3, result in either activation or suppression: CaSR is “reset” so that higher or lower than normal blood Ca 2+ is sensed by the receptor as “normal.” Whereas inactivating (loss-of-function) mutations of the CaSR cause hypercalcemia (hyperparathyroidism), activating (gain-of-function) mutations cause a hypocalcemic syndrome of varying severity (hypoparathyroidism).
PTH acts directly on bone, stimulating resorption, thereby releasing Ca and P into circulation. PTH acts directly on the kidneys to increase urinary P excretion and decrease urinary Ca excretion. PTH indirectly enhances intestinal Ca absorption through effects on 1,25(OH) 2 D synthesis. Thus, the net actions of PTH increase serum Ca.
After birth, the kidneys play an important role in Ca and P homeostasis by regulating mineral loss via urine. Under normal circumstances, nearly all (98%) filtered Ca is reabsorbed in the renal tubule, but Ca excretion is modified by local and systemic factors (e.g., PTH) to regulate extracellular Ca. Urinary Ca increases over the first 2 weeks of life. Both in preterm and term neonates, the kidneys respond to exogenous PTH, as measured by increased production of nephrogenous cyclic AMP (cAMP), and improve with increasing postnatal age.
In normal term infants, when serum Ca decreases after birth, PTH increases appropriately (twofold to fivefold increase during 48 hours) and remains elevated for days. PTH secretion in term infants appears substantial and negatively correlates with Ca levels. Term neonates also show appropriate calcemic response when challenged with PTH. However, in extremely preterm infants, even with significant hypocalcemia as a stimulus, PTH remains low for the first 2 days. Transient hypocalcemia typically resolves within the first week and often requires no treatment.
Vitamin D
Vitamin D is necessary for maintenance of normal Ca and P homeostasis. 1,25(OH) 2 D is the major hormone affecting active intestinal Ca and P absorption. As a steroid, vitamin D binds to genomic receptors in intestinal epithelial cells and increases synthesis of Ca-binding proteins (calbindin), leading to increased Ca absorption. 1,25(OH) 2 D acts on kidneys to conserve Ca and P. The overall result of this hormone is to increase serum Ca and P. 1,25(OH) 2 D production by renal proximal tubule is enhanced by hypocalcemia, hypophosphatemia, PTH, and PTHrP and appears tightly regulated. 1,25(OH) 2 D production is inhibited by elevated serum Ca and P.
25-hydroxyvitamin D (25OHD) crosses the placenta, and cord 25OHD correlates significantly with maternal 25OHD; fetal vitamin D pool depends entirely on the maternal vitamin D status (i.e., maternal sun exposure and dietary D intake). 1,25(OH) 2 D is produced by the fetal kidneys and placenta, and vitamin D receptor (VDR) is present in many fetal tissues, including the placenta. The placenta synthesizes and metabolizes 1,25(OH) 2 D through activity of 25OHD-1α hydroxylase and 1,25(OH) 2 D-24 hydroxylase, two key enzymes for vitamin D metabolism.
However, mice deficient in 1-α-hydroxylase are grossly normal at birth and until weaning. Additionally, mice with null mutation of VDR show no alteration in placental Ca transfer or fetal serum of Ca, P, or Mg despite significant maternal hypocalcemia. The effect of 1,25(OH) 2 D on placental Ca transfer, if any, could relate to expression of placental Ca transporter PMCA3 mRNA.
Insufficiency of infant vitamin D arises from maternal vitamin D deficiency (sunshine deprivation with insufficient vitamin D intake), reduced production of active vitamin D metabolites caused by liver or renal disease, congenital deficiency of renal 1-α-hydroxylase, and 1,25(OH) 2 D resistance. Deficiency of vitamin D or its metabolites causes decreased intestinal Ca absorption and renal Ca reabsorption and neonatal hypocalcemia.
25-hydroxyvitamin D (25OHD), the major marker of vitamin D status, is lower in preterm than in term infants. However, conversion of 25OHD to 1,25(OH) 2 D occurs normally in premature infants. In normal term infants, serum 1,25(OH) 2 D is low at birth but increases to adult ranges by 24 hours, possibly reflecting a need for optimum intestinal Ca and P absorption. In preterm infants, serum 1,25(OH) 2 D at birth is comparable to that in healthy children and adults, increases significantly during the first few days, and is far above reference values between 3 and 12 weeks. Cord serum 1,25(OH) 2 D is lower in small-for-gestational-age infants compared with weight-appropriate infants, possibly reflecting decreased 1,25(OH) 2 D production from reduced uteroplacental blood flow.
Calcitonin
CT is a Ca 2+ -lowering hormone produced by thyroid parafollicular cells and acts as a physiologic antagonist to PTH. CT secretion is under direct control of blood Ca. Elevation in Ca 2+ stimulates CaSR and lowers Ca 2+ by enhancing CT secretion; decreased Ca 2+ causes a decrease in CT. After being secreted, CT has a circulatory half-life of 2 to 15 minutes. CT inhibits osteoclast-mediated bone resorption (decreasing Ca and P release) and secondarily increases renal Ca and P excretion (at high doses). The net consequence of CT is decreased serum Ca and P.
CT is produced in fetal thyroid; fetal and newborn CT is higher than in the mother, related to chronic fetal hypercalcemia. Recently, ablation of CT and CT gene-related peptide (CT/CGRP-null mice) produced serum Ca and placental Ca transfer identical to wild-type littermates. There was a small, nonsignificant trend toward decreased serum P, but serum Mg was reduced by almost 50%. Thus, the role of CT in fetuses is unclear.
Cord serum CT decreases with increasing gestational age. Infants less than 32 weeks’ gestation have nearly three times term cord serum CT. After birth, serum CT further increases in both preterm and term infants, peaking at 24 to 48 hours, followed by a decline to childhood values by 1 month. The physiologic importance of this increase is unclear but may relate to CT counteracting PTH bone resorptive action.
Regulation of Phosphorus Homeostasis
In contrast to regulation of Ca, the regulation of P homeostasis during the fetal and neonatal periods is less well understood. The regulation of P homeostasis involves several different hormones that act on kidney, intestine, and bone: fibroblast growth factor 23 (FGF23), PTH, and 1,25(OH) 2 D.
Placental Transport
Similar to Ca, P is higher in fetal serum compared with maternal serum, and PTHrP appears to help prevent additional elevation. Transplacental P transport is an active sodium-dependent process against concentration gradient. Inorganic P enters placental trophoblast via NaPi-IIb, a family member of sodium-dependent inorganic P transporters, critical for intestinal P transport. In a mouse model that is null for NaPi-IIb, unexpected embryonic lethality was found. NaPi-IIb is expressed in the embryonic endoderm and placental labyrinthine zone (where embryonic and maternal circulations are in closest contact), consistent with a role in placental P transfer. However, much remains to be learned about additional proteins involved in P transfer and about their regulation.
Parathyroid Hormone
Circulating P is determined by a balance between intestinal P absorption, storage in the skeleton, and P reabsorption from the kidneys. PTH synthesis and secretion are upregulated by low serum Ca and increased serum P, and downregulated by increased serum Ca and 1,25(OH) 2 D and by increased FGF23. The net effect of these actions is increased serum Ca and decreased serum P. PTH acts on the proximal tubule, where it rapidly decreases NaPi-IIa and NaPi-IIc expression and thereby leads to phosphaturia. PTH stimulates 1,25(OH) 2 D synthesis. Together with PTH, 1,25(OH) 2 D furthermore acts on bone to increase the release of Ca (and P) into ECF. P regulation therefore can be either independent of, or intimately tied to, Ca regulation.
1,25(OH) 2 D expression is upregulated by PTH and downregulated by increased serum Ca, P, and FGF23. 1,25(OH) 2 D acts through VDR/RXR dimers to stimulate intestinal P absorption, FGF23 synthesis, and secretion by osteocytes, and possibly to inhibit parathyroid PTH secretion. Its net effect is increase in serum Ca and P.
Absorbed P enters the extracellular P pool in equilibrium with bone and soft tissue. In adults with a neutral P balance, P excreted by the kidneys is equal to the net P absorbed by the intestine; in growing infants, it is less than net absorbed, owing to deposition of P in soft tissues and bone. In growing infants, P preferentially goes to soft tissue with a nitrogen-to-P (weight) ratio of 15:1 and to bone with a Ca-to-P (weight) ratio of 2.15:1. The residual P constitutes renal P load, influencing plasma and urinary P. In limited P supply, bone mineral accretion may be limited, leading to significant Ca excretion associated with very low urinary P excretion.
During P depletion, phosphaturia decreases before serum P declines through increased tubular reabsorption. Hypophosphatemic challenge results in stimulation of kidney 1,25(OH) 2 D synthesis, enhanced mobilization of P and Ca from bone, a hypophosphatemia-induced increase in tubular maximum reabsorption for P (TmP), and decreased renal P excretion. Increased circulating 1,25(OH) 2 D increases P and Ca absorption in the intestine and stimulates P and Ca mobilization from the bone. The increased flow of Ca from the bone and intestine inhibits PTH secretion and lowers P excretion. The net result is a return of serum P to normal without a change in serum Ca. FGF23 may possibly be downregulated in low serum P and may increase NaPi-II expression, leading to decreased renal P excretion.
Defense against hyperphosphatemia consists largely of a reversal of these events. The principal humoral factor is PTH. An acute increase in serum P produces a transient decrease in serum ionized Ca and stimulation of PTH, and possibly upregulates FGF23 expression, which reduces TmP/GFR by reducing renal NaPi-II expression, leading to increased renal P excretion and readjustment in serum P and Ca.
Fibroblast Growth Factor 23
FGF23, a bone derived hormone, in concert with its coreceptor Klotho, has a major role in the regulation of serum P concentration, although the mechanism by which FGF23-producing cells sense P remains to be elucidated. Synthesized in bone, FGF23 is released into the circulation and acts on the proximal tubule to enhance, within hours, urinary P excretion by reducing the expression levels of NaPi-IIa and NaPi-IIc. Furthermore, FGF23 decreases renal production of 1,25(OH) 2 D and thus reduces intestinal P absorption ( Fig. 5.2 ).

FGF23 is part of the newly recognized endocrine bone–parathyroid–kidney axis modulated by PTH, 1,25(OH) 2 D, and dietary and serum P levels. Synthesis and secretion of FGF23 by osteocytes are upregulated by increased serum 1,25(OH) 2 D and serum P and downregulated by P-regulating gene homologies to endopeptidases on the X chromosome (PHEX) and dentin matrix protein 1 (DMP1). In turn, FGF23 acts through FGF receptors, with Klotho as coreceptor, to inhibit renal P reabsorption, 1,25(OH) 2 D synthesis, and parathyroid PTH secretion. FGF23 synergizes with PTH to increase renal P excretion by reducing expression of renal NaPi-IIa and NaPi-IIc in the proximal tubules. Its net effect is reduction in serum P and 1,25(OH) 2 D, which may result in hypocalcemia.
In early neonatal mineral metabolism, the role of FGF23, a novel regulator of P and vitamin D homeostasis, has not been evaluated. Recently, circulating FGF23 levels, using an intact FGF23 ELISA(iFGF23) and a C-terminal FGF23 ELISA(cFGF23), were measured in umbilical cord samples or in term infants at 5 days of life, and compared with healthy adult controls. The iFGF23 (32 kDa) was low and the fragmented cFGF23 (18 kDa) was abundant in the cord blood compared with those in the healthy adults. By 5 days of life, the iFGF23 was increased to near adult concentration, and cFGF23 was twice adult values at cord blood and at 5 days of life. The iFGF23/cFGF23 ratio was very low due to the fragmentation of FGF23 during the early postpartum period, possibly indicating a considerable contribution to the P homeostasis in the healthy term infants.
In healthy appropriate-for-gestational-age preterm infants (median gestational age of 31.2 weeks), iFGF23 and cFGF23 concentrations were persistently elevated at 1, 3, and 5 weeks of life and at term age, double and 10 times adult norms, respectively. No infants were vitamin D deficient, and tubular reabsorption of P was normal (88% ± 8%). Persistent elevation of iFGF23 and cFGF23 was surprising. While the cFGF23 is elevated in both cord blood and at 5 days, preterms maintained iFGF23 levels that were twice adult concentrations, and cFGF23 remained 10 times higher. It is not clear why cFGF23 is more markedly elevated in preterm than in term infants. Both forms of FGF23 have short half-lives of about 1 hour, and the higher concentrations of cFGF23 may reflect lack of clearance as well as rapid production of iFGF23. Of note, cFGF23 is known to inhibit iFGF23 by activating on its receptor in conjunction with alpha Klotho. Considering the recent report of increased concentrations of Klotho in cord blood compared with adults, the elevated cFGF23 levels could possibly attenuate potent phosphaturic effects. However, the biologic significance of the elevated FGF23 in preterm infants and in term infants at 5 days of life is unclear. Elevated FGF23 concentrations are most often associated with increased phosphaturia and are seen in a number of hereditary hypophosphatemic forms of rickets; increased levels are also described in a number of other disorders. The role of FGF23 in early life remains to be established.
Renal Excretion
The kidneys are the major determinant of plasma P. Because intestinal P absorption is very efficient and fairly unregulated (only 30% is regulated by 1,25(OH) 2 D), renal P excretion is important in maintaining balance. Serum P is maintained at close to the tubular P threshold, or TmP/GFR, via an active and saturable reabsorption process.
Most filtered P is reabsorbed in the proximal tubule of the kidney through the NaPi-II. Increased P intake (e.g., from formula with a high P content) theoretically leads to rapid downregulation of NaPi-II mRNA and protein in the brush-border membrane of the proximal tubule and increased P excretion. Renal P reabsorption lies under tight hormonal control by PTH and FGF23 (which inhibits P reabsorption) and, to a lesser extent, insulin and hormones of the somatotropic–pituitary axis. In contrast, 1,25(OH) 2 D synthesis, stimulated by decreased plasma P, has an indirect effect on renal P reabsorption via intestinal P absorption and bone P mobilization, resulting in increased serum P and suppression of PTH.
FGF23 principally functions as phosphaturic factor and counterregulates 1,25(OH) 2 D production. Excess FGF23 secreted by osteocytes causes hypophosphatemia through inhibition of renal NaPi-II and suppresses 1,25(OH) 2 D through inhibition of 25OHD-1α-hydroxylase and stimulation of 24-hydroxylase, which inactivates 1,25(OH) 2 D in the proximal tubule. In contrast, deficiency of FGF23 results in hyperphosphatemia and elevated 1,25(OH) 2 D production. In theory, downregulation of FGF23 could promote relative hyperphosphatemia and a relative increase in 1,25(OH) 2 D, promoting bone mineralization during early neonatal rapid growth.
The kidneys contribute to positive P balance during growth by reabsorption of a relatively high fraction of filtered inorganic P (99% in newborns and 80% in adults). Growing infants, in particularly preterm infants, have high fractional reabsorption of P, reflecting their high P need. Age-related decrease in P reabsorption may relate to lower P needs with advancing age.
Wide variation in serum P concentrations corresponds to few direct regulatory mechanisms. PTH, which has the greatest impact on serum P, primarily responds to changes in ionized Ca, not P. P is freely filtered at the glomerulus and presents to the renal tubules in high concentrations. The renal tubule reabsorbs P in both the proximal and distal nephrons. In states of low PTH, renal tubular cells reabsorb up to 95% to 97% of filtered P. In states of high PTH, P reabsorption in the proximal and distal tubules is inhibited, resulting in high urinary P excretion. Although markedly affected by PTH in the usual state, renal tubular cells have altered PTH responsiveness in severe P deficiency or overload. Hence, P is reabsorbed when there is severe P deficiency, even in high PTH states, and P is excreted when serum P is high despite low PTH.
During early postnatal life, whereas renal P response to PTH is blunted, PTH increases tubular Ca reabsorption. Together these actions result in retention of both Ca and P in infants, which is favorable for growth. Maternal smoking during pregnancy negatively influences Ca-regulating hormones, leading to relative hypoparathyroidism in both the mother and newborn and lower PTH and 25-OHD in the smoking mother and newborn despite higher serum P.
Clinical Disorders Associated With Abnormal Calcium and Phosphorus Homeostasis
Neonatal Hypocalcemia
Definition
Hypocalcemia is generally defined as serum total Ca below 2 mmol/L (8.0 mg/dL) in term and below 1.75 mmol/L (7 mg/dL) in preterm infants or ionized Ca below 0.75 to 1.1 mmol/L (3.0–4.4 mg/dL). “Early-onset” neonatal hypocalcemia typically occurs during the first few days of life, with the lowest Ca at 24 to 48 hours, and “late-onset” hypocalcemia occurs toward the end of the first week of life.
Etiology and Pathophysiology
Early-onset neonatal hypocalcemia is commonly associated with prematurity, birth asphyxia, maternal insulin-dependent diabetes, gestational anticonvulsant exposure, and maternal hyperparathyroidism. Late-onset hypocalcemia, which is less frequent, is commonly associated with relatively high P-containing diets, disturbed maternal vitamin D metabolism, intestinal malabsorption of Ca, hypomagnesemia, and hypoparathyroidism.
Early-onset neonatal hypocalcemia represents exaggeration of normal serum Ca decrease during the first 24 to 48 hours of life; there is inadequate compensation for the sudden loss of placental Ca supply at birth (e.g., insufficient PTH release by immature parathyroids or inadequate renal response to PTH). Preterm infants may or may not exhibit the surge in PTH secretion of term infants at birth, and restricted oral Ca intake aggravates the problem. In asphyxiated infants, decreased Ca intake as a result of delayed feedings, increased endogenous P load, bicarbonate alkali therapy, and increased serum CT may contribute to the development of hypocalcemia. Hypocalcemia in infants of insulin-dependent diabetic mothers appears to be related to magnesium (Mg) insufficiency and consequent impaired PTH secretion. Mg is important in PTH secretion and action; chronic Mg deficiency causes impaired PTH secretion and PTH resistance at target organs. Transient neonatal hypoparathyroidism occurs in infants exposed to maternal hypercalcemia in utero. Intrauterine hypercalcemia suppresses fetal parathyroids and apparently impairs PTH production response to hypocalcemia after birth.
Late-onset hypocalcemia commonly results from dietary Ca and P imbalance and rarely may result from maternal hypercalcemia. Infants receiving cow’s milk–derived formulas have lower serum ionized Ca and high serum P in the first week compared with breastfed infants, related to a higher absolute P amount of formula or limited P excretion from low newborn GFR. P-containing enemas cause P overload and hypocalcemia. Late hypocalcemia may relate to resistance of immature kidneys to PTH, leading to renal P retention and hypocalcemia; biochemical features resemble pseudohypoparathyroidism (defects in the GNAS1 gene), but with normal nephrogenous cAMP responses to PTH.
Congenital hypoparathyroidism is the most significant cause of late-onset hypocalcemia that has to be treated early. Congenital hypoparathyroidism may be a part of DiGeorge triad of hypoparathyroidism; T-cell incompetence (partial or absent thymus on chest radiograph); and conotruncal heart defects or aortic arch abnormalities, which suggest 22q11 syndrome (CARCH22 or DiGeorge sequence).
Isolated hypoparathyroidism includes genetic defects that impair PTH synthesis (PTH gene defects) or secretion (CASR gene defects) or parathyroid gland development (GCMB gene defects; parathyroid agenesis). Gain-of-function (activating) mutations in the CASR gene-encoding CaSR are the most common cause of mild isolated hypoparathyroidism (autosomal dominant hypocalcemia), associated with inappropriately low or low-normal serum PTH and relative hypercalciuria.
Clinical Presentation
Neonates with hypocalcemia may be asymptomatic; the less mature the infant is, the more subtle and varied the clinical manifestations will be. Main clinical signs are jitteriness, tremors, twitching, and exaggerated startle responses or seizures (generalized or focal). Frank convulsions are seen more commonly with late hypocalcemia. Infants also may be lethargic, feed poorly, vomit, and have abdominal distension. Apnea, cyanosis, tachypnea, tachycardia, vomiting, or heart failure may also be seen. The classic signs of peripheral hyperexcitability of motor nerves (carpopedal spasm and laryngospasm) are uncommon in newborns.
Diagnosis
The diagnosis of hypocalcemia is based on serum ionized or total Ca levels, history, and physical examination; serum P, Mg and glucose, and serum pH are helpful. Functional atrioventricular block from electrocardiographic prolonged QTc interval (>0.4 second) suggests hypocalcemia.
When an infant is refractory to therapy or there are unusual findings, measurement of PTH, 25OHD, and 1,25(OH) 2 D may be useful in establishing the less common causes (e.g., primary hypoparathyroidism, malabsorption, and vitamin D metabolism disorders) of hypocalcemia. Normal to moderately elevated 1,25(OH) 2 D levels are consistent with hypoparathyroidism. Hypercalciuria (urinary Ca ≥4 mg/kg/day or urine Ca:creatinine ratio ≥0.2 [mg/mg]) associated with hypocalcemia supports low PTH states.
Prolonged hypocalcemia should prompt investigation of permanent causes, such as hypoparathyroidism. DNA analysis for causal mutations may help confirm the diagnosis in proband and relatives.
Therapeutic Approaches
Treatment of symptomatic hypocalcemia is by intravenous (IV) Ca infusion with a dosage of 30 to 75 mg/kg/day of elemental Ca, titrated to clinical and biochemical response, to maintain ionized Ca in the low-normal range. Clinical hypocalcemia signs are usually reversed rapidly by correcting serum Ca, which helps confirm the diagnosis.
During seizures (serum Ca usually <1.5 mmol/L [6 mg/dL]), emergency Ca (1–2 mL/kg of Ca gluconate 10%; ≈9–18 mg/kg of elemental Ca) is given IV over 10 minutes as heart rate is measured continuously to prevent bradycardia. If this complication occurs, Ca infusion should be discontinued temporarily. Another complication of Ca infusion is potential skin tissue injury from infusate extravasation. A follow-up bolus or intermittent infusions of Ca salts are avoided because of wide serum Ca excursions.
For less urgent purposes or for follow-up after initial seizure treatment, continuous IV administration of 75 mg/kg/day of elemental Ca will maintain normocalcemia. Afterward, stepwise IV Ca reduction may help prevent rebound hypocalcemia (75 mg/kg/day for the first day, a half dose the next day, a half dose the third day, and discontinued the fourth day). Alternatively, if oral fluids are tolerated, the same dose of Ca gluconate can be given orally, divided among four to six doses per day after initial correction. Oral Ca may not be practical in sick infants because of bowel stimulation; proprietary oral Ca preparations are hypertonic (high osmolar) and are not used in infants at risk of necrotizing enterocolitis. Vitamin D metabolites are not useful for early hypocalcemia because of variable response and side effects.
With persistent hypocalcemia, serum Mg is measured because hypomagnesemic hypocalcemia cannot be corrected until hypomagnesemia is alleviated. Hypomagnesemia (serum Mg <0.6 mmol/L [1.5 mg/dL]) is treated with Mg sulfate 50% solution (500 mg or 4 mEq/mL), 0.1 to 0.2 mL/kg, IV or intramuscular (may cause local tissue necrosis) and repeated after 12 to 24 hours. Serum Mg is obtained before each dose (one or two doses may resolve transient hypomagnesemia).
Because most causes of neonatal hypocalcemia are transient, therapy duration varies with cause; commonly, as little as 2 to 3 days for early hypocalcemia is needed. Ca supplementation is usually required for long periods in hypocalcemia from malabsorption or hypoparathyroidism.
In neonates at risk, early hypocalcemia can be prevented by early oral feeding or parenteral Ca supplementation (75 mg/kg/day of elemental Ca continuously) to maintain total Ca above 2 mmol/L (8.0 mg/dL) and ionized Ca above 1 mmol/L (4.0 mg/dL). This may help prevent hypocalcemia in sick newborns with cardiovascular compromise requiring cardiotonic drugs or pressure support.
Most asymptomatic neonatal hypocalcemia resolves spontaneously with time, but hypocalcemia has potential adverse effects on the cardiovascular and central nervous systems, so treatment may be needed. For asymptomatic ill infants and infants with severe hypocalcemia (serum total Ca <1.5 mmol/L [6.0 mg/dL] or ionized Ca <0.75 mmol/L [3 mg/dL]), either IV or oral therapy is usually required. In sick infants, judicious bicarbonate use and avoidance of respiratory alkalosis from excessive ventilation may reduce the risk of symptomatic hypocalcemia.
For late hypocalcemia, treatment goals are to reduce the P load and increase Ca absorption by using feedings with a Ca-to-P ratio of 4:1 or greater, such as use of low-P feedings (human milk or low-P formula) in conjunction with an oral Ca supplement.
Treatment of hypoparathyroidism is directed at maintaining plasma Ca to prevent symptoms without causing nephrocalcinosis. Hypoparathyroidism requires therapy with 1,25(OH) 2 D (or 1 α-hydroxyvitamin D 3 , a synthetic analog) and lifelong Ca supplementation. Infants with severe or persistent hypocalcemia may benefit from 1,25(OH) 2 D, IV or orally, 50 to 100 ng/kg/day in two or three divided doses. Close follow-up is required to monitor the serum Ca level. When serum Ca level is normalized, administration of 1,25(OH) 2 D may be discontinued to prevent hypercalcemia.
Recombinant PTH (teriparatide) has been tried as initial management of neonatal hypoparathyroidism. In infants with life-threatening seizures and persistent hypocalcemia despite aggressive management with high doses of 1,25(OH) 2 D and Ca infusion, short-term use of teriparatide (5 µg subcutaneously) can raise Ca levels faster (in <4 hours) than other commonly used methods, which take 1 day or longer. Theoretically, teriparatide is safer and is a more physiologic means of correcting acute hypoparathyroid hypocalcemia; however, there is a concern regarding the risk of osteosarcoma related to long-term exposure.
Neonatal Hypercalcemia
Definition
Neonatal hypercalcemia is serum total Ca greater than 2.75 mmol/L (11 mg/dL) or ionized Ca greater than 1.4 mM/L (5.6 mg/dL).
Etiology and Pathophysiology
Hypercalcemia is uncommon in term infants but relatively common in preterm infants. The most common causes are relative deficiency in P supply and hypophosphatemia from inappropriate parenteral nutrition (PN), with or without excessive Ca or human milk feeding in preterm infants (low P content relative to preterm needs). Iatrogenic hypercalcemia results from excessive Ca or vitamin D for hypocalcemia or during exchange transfusion. Chronic maternal exposure to excessive vitamin D or metabolites, secondary to treatment of maternal hypocalcemic disorders, may cause hypercalcemia of the mother and neonate. Chronic diuretic therapy with thiazides during pregnancy may lead to maternal, fetal, and neonatal hypercalcemia.
Other rarer causes include hyperparathyroidism (primary or secondary to maternal hypoparathyroidism) and hypercalcemia associated with subcutaneous fat necrosis, idiopathic infantile hypercalcemia, severe infantile hypophosphatasia, and a Bartter syndrome variant. Primary hyperparathyroidism is rare in neonates and children.
Elevated serum Ca in pathologic conditions with PTH or vitamin D overactivity implies increased Ca efflux into ECF from bone, intestine, or kidney. Hypophosphatemia increases circulating 1,25(OH) 2 D, which increases intestinal Ca absorption and bone resorption; Ca is not deposited in bone in the absence of P and contributes to hypercalcemia.
Homozygous inactivating mutations of CaSR produce severe hypercalcemia, termed neonatal severe primary hyperparathyroidism (NSPHT). Heterozygous inactivating mutations of CaSR produce a “benign” hypercalcemia, termed familial hypocalciuric hypercalcemia (FHH), inherited as an autosomal dominant trait with high penetrance. Mutations in Ca +2 -sensor lead to a dual defect in parathyroid cells (causing parathyroid hyperplasia) and renal tubules (causing hypocalciuria). FHH and NSHPT are associated with mutations in the CASR gene at 3q13.3-21 in nearly all affected subjects ; in some families, the disorder is linked to unknown genes on the long or short arms of chromosome 19.
Hypercalcemia associated with subcutaneous fat necrosis occurs in asphyxiated, large-for-gestational-age infants; possible mechanisms are increased prostaglandin E (PGE) activity, increased Ca release from fat and tissues, and unregulated production of 1,25(OH) 2 D from macrophages infiltrating fat necrotic lesions.
Idiopathic infantile hypercalcemia (which may be part of Williams syndrome) is associated with mutations in the elastin gene on the long arm of chromosome 7; there may be a vitamin D hyperresponsive state and blunted CT response to Ca loading. Infantile hypophosphatasia is a rare autosomal recessive disorder that may be lethal in utero or shortly after birth because of inadequate bony support of the thorax and skull. Bartter variant–related hypercalcemia is associated with polyhydramnios and prematurity; in utero hypercalcemia may result in fetal hypercalciuria and polyuria, leading to early delivery; increased serum 1,25(OH) 2 D, normal serum PTH, and increased urinary PGE 2 are present.
Clinical Presentation
Clinical features of hypercalcemia depend on the underlying disorder, age, and degree of hypercalcemia. Its onset may be at birth or delayed for weeks or months. Neonates with hypercalcemia may be asymptomatic or have serious clinical signs (especially in hyperparathyroidism) requiring urgent treatment. Infants with mild increases in serum Ca (2.65–3.25 mmol/L [11–13 mg/dL]) often have no specific signs. Mild hypercalcemia may present as feeding difficulties or poor linear growth. Unrecognized hypercalcemia can result in significant morbidity or death.
With moderate to severe hypercalcemia, nonspecific signs, such as anorexia, vomiting and constipation (rarely diarrhea), polyuria, and dehydration, may occur. Infants with chronic hypercalcemia may present with failure to thrive (poor growth). Severe hypercalcemia can affect the nervous system and cause lethargy, drowsiness, irritability, confusion, and seizure; in extreme cases, stupor and coma ensue. Thus, timely recognition and treatment of hypercalcemia are critical. In severely affected infants, hypertension, respiratory distress (caused by hypotonia and demineralization and deformation of the rib cage), nephrocalcinosis (from long-standing hypercalcemia), and band keratopathy of the limbus of the eye (rare) may be present. Associated features, such as elfin faces, cardiac murmur, and mental retardation (in Williams syndrome) and bluish-red skin indurations (in subcutaneous fat necrosis), may be present on physical examination.
Diagnosis
The diagnosis may be made incidentally on routine chemistry screening. The workup may include serum total Ca, ionized Ca, P, Mg, alkaline phosphatase, pH, total protein, creatinine, electrolytes, PTH, and 25OHD; urine Ca, P, tubular reabsorption of P, and cAMP with renal function evaluation; and chest and hand x-ray radiography, abdominal ultrasonography, ophthalmologic evaluation, and electrocardiography (i.e., shortened QT interval) to determine the effect of hypercalcemia.
Very elevated serum Ca (>3.75 mmol/L [15 mg/dL]) usually indicates primary hyperparathyroidism or P depletion in VLBW infants. To differentiate parathyroid from nonparathyroid conditions, measurements include serum P (low in hyperparathyroidism, FHH, and rickets of prematurity), percent renal tubular P reabsorption (<85% in hyperparathyroidism; high in rickets of prematurity associated with hypophosphatemia), and serum PTH (elevated in hyperparathyroidism). A very low urinary Ca/urinary creatinine ratio [U ca /U cr ] in the face of hypercalcemia suggests FHH.
The diagnosis of NSPHT is based on inappropriately normal or elevated PTH along with relative hypocalciuria in severe hypercalcemia (high Ca levels, 5–6 mmol/L [20–24 mg/dL]). Hyperparathyroidism causes erosion of bone (particularly along long bone subperiosteal margins; a “moth-eaten” appearance), which may be mistaken for rickets. In contrast to NSPHT, FHH infants usually remain asymptomatic. PTH is usually in the normal range, but inappropriately high for hypercalcemia. Urine Ca excretion is low, and nephrocalcinosis is not a problem. A family history of FHH or NSPHT in a sibling provides a strong confirmation. Care must be taken to distinguish these disorders from transient neonatal hyperparathyroidism associated with maternal hypocalcemia (e.g., in maternal pseudohypoparathyroidism or renal tubular acidosis).
DNA analysis for FHH and NSPHT is available in few laboratories and requires molecular analysis of the entire CASR gene in a proband. Relatives of the patient’s proband may be studied for genetic abnormalities and serum Ca.
Bone radiographs identify demineralization, osteolytic lesions (hyperparathyroidism), or osteosclerotic lesions (occasionally with vitamin D excess).
A maternal dietary and drug history (e.g., excessive vitamin A or D, thiazides) or history of possible mineral disturbances or polyhydramnios during pregnancy should be sought. Family screening will depend on the primary diagnosis.
Additional information concerning nephrocalcinosis from hypercalcemia or soft tissue calcification (e.g., in basal ganglia) can be obtained by ultrasonography or computed tomography. If hyperparathyroidism is diagnosed (rarely), localization of a parathyroid adenoma or hyperplasia by radionuclide scintigraphy may be useful.
Therapeutic Approaches
Therapy includes correction of specific underlying causes and removal of iatrogenic or external causes (e.g., surgical removal of hyperparathyroid glands, stopping excessive Ca or vitamin D intake). Treatment of neonatal hyperparathyroidism depends on the severity. For mild asymptomatic hypercalcemia in a thriving infant, conservative management is appropriate. For moderate to severe hypercalcemia, prompt investigation and more aggressive therapy are instituted; stopping excessive dietary Ca and vitamin D intake and maintenance of adequate hydration are mainstays; and renal Ca excretion is enhanced by loop-acting diuretics. Reduced dietary Ca intakes by low Ca formula and inhibition of bone resorption by antibone resorptive agent may be used.
For short-term treatment of acute hypercalcemic episodes (symptomatic or serum Ca >3.5 mmol/L [14 mg/dL]), expansion of the ECF with 10 to 20 mL/kg of 0.9% sodium chloride IV followed by IV 1 mg/kg of furosemide every 6 to 8 hours may be effective by increasing urinary Ca excretion. Fluid and electrolyte imbalance is avoided by monitoring of fluid balance and serum Ca, P, Mg, sodium, potassium, and osmolarity; reduced GFR from dehydration can worsen hypercalcemia.
For restriction of dietary intakes of Ca and vitamin D, a low-Ca, low–vitamin D 3 infant formula containing trace Ca amounts (<10 mg/100 kcal versus standard formula, 78 mg/100 kcal) and no vitamin D (also low iron) is available for short- to medium-term management (CalciloXD, Ross Laboratories, Columbus, Ohio); iron supplement is needed. As hypercalcemia resolves, usual formula or human milk (≈10 mg/oz of Ca) can be mixed with the CalciloXD to increase Ca intake, closely monitoring serum and urine Ca to prevent rickets or hypocalcemia.
Adjuvant therapies in acute hypercalcemia are CT, glucocorticoids, bisphosphonate, and dialysis. Minimal information is available in neonatal hypercalcemia. Symptomatic infants with nonparathyroid hypercalcemia may require long-term CT or bisphosphonates. Short-term salmon CT (4–8 IU/kg every 6 to 12 hours subcutaneously or intramuscularly), prednisone (1–2 mg/kg/day), or a combination may be useful. The hypocalcemic effect of CT (a potent inhibitor of bone resorption) is transient and abates after a few days, which is not ideal for chronic therapy; effects may be prolonged with concomitant glucocorticoids, although there is limited experience in neonates.
High-dose glucocorticoids reduce intestinal Ca absorption and may decrease bone resorption; methylprednisolone (1–2 mg/kg/day IV), hydrocortisone (10 mg/kg/day IV), or the equivalent is effective, but is not recommended for long-term use because of many undesirable side effects. Although effective in several types of hypercalcemia, glucocorticoids are relatively ineffective in patients with primary hyperparathyroidism.
Bisphosphonate (an antibone resorptive agent) may be useful to treat hypercalcemia that is PTH mediated and for subcutaneous fat necrosis. Infants with NSPHT and marked hypercalcemia should be managed aggressively. In the past, treatment was urgent subtotal parathyroidectomy; more recent options include IV bisphosphonates (pamidronate 0.5–2.0 mg/kg) with parathyroidectomy delayed until the patient is clinically stable. Bisphosphonate therapy seems safe in the short term and effective in controlling hypercalcemia even in very premature infants, allowing for planned surgery when feasible.
For severe and unremitting hypercalcemia, either hemodialysis (HD) if the patient is hemodynamically stable or peritoneal dialysis (PD) with a low-Ca dialysate (1.25 mM/L) may be helpful. To avoid iatrogenic mineral depletion, for HD or PD, supplemental P or Mg is given orally or IV, or sodium phosphate is added to PD solution (<0.75 mM); in PD, crystal formation in bags should be inspected hourly and fresh solutions changed every 8 hours.
Neonatal Hypophosphatemia
Definition
Hypophosphatemia is serum P below 1.65 mmol/L (4 mg/dL). Conventionally, hypophosphatemia is often graded as mild when serum P is below 1.45 mmol/L (<3.5 mg/dL), moderate when it is below 1.0 mmol/L (<2.5 mg/dL), and severe when it is below 0.4 mmol/L (<1.0 mg/dL).
Etiology and Pathophysiology
Hypophosphatemia occurs when there is decreased P intake (decreased intestinal absorption or increased intestinal loss) or excess renal wasting from a renal tubular defect or hyperparathyroidism. P deficiency is seen in preterm infants with rickets of prematurity resulting from inadequate Ca and P intakes.
The pathophysiologic consequences of P deficiency are attributable to both direct and indirect effects of hypophosphatemia. P deficiency may directly enhance bone resorption and decrease matrix formation and bone mineralization. When serum P decreases, renal P excretion decreases and renal 1,25(OH) 2 D production increases, which in turn increases intestinal Ca absorption and mainly stimulates bone resorption, releasing P and Ca (possibly a compensatory mechanism in an attempt to maintain serum P for essential function). The excess Ca results in hypercalcemia and hypercalciuria. Serum P remains low because P released from bone is used in intracellular metabolism. In VLBW infants, increased Ca absorbed from the gut or mobilized from the bone by 1,25(OH) 2 D may not be used for bone mineralization and leads to “excess” filtered Ca being excreted in the urine.
P deficiency is closely linked to metabolic bone disease in VLBW infants because P promotes bone formation and matrix production and limits bone resorption. In rats, P deficiency produces a histologic picture distinct from vitamin D deficiency.
In preterm infants, a P depletion syndrome occurs in infants fed human milk, with restricted enteral mineral intakes (mineral-unfortified formula), and with chronic illnesses receiving prolonged PN without mineral supplements. In the latter group, biochemical signs of extreme P deficiency are prominent, with rachitic bone changes; serum 1,25(OH) 2 D increases with increased bone resorption and turnover (increased serum bone resorption marker, cross-linked carboxyterminal telopeptide of type I collagen and osteocalcin).
Pathophysiologic results of P deficiency are inadequate supplies of energy-rich P and, in particular, inhibition of glyceraldehyde-3-phosphate dehydrogenase, which occupies a key position in glycolysis. The effect of P deficiency on energy metabolism is to reduce adenosine triphosphate (ATP) and 2,3-diphosphoglycerate, leading a shift of the oxygen-hemoglobin dissociation curve to the left, with decreased peripheral oxygen uptake and transport.
Prolonged starvation, malabsorption, and chronic diarrhea cause hypophosphatemia related to decreased intestinal absorption. A chronically malnourished patient is often in a catabolic state, associated with muscle breakdown and subsequent loss of intracellular P. When patients subsequently receive nutrition support, they may receive a P-depleted feed, especially with PN, when large volumes of carbohydrate and amino acid solutions raise endogenous P requirements, and unbalanced amino acid solutions may induce further urinary P losses. If P replacement is insufficient, then hypophosphatemia will ensue.
Diseases of vitamin D metabolism (vitamin D–dependent rickets) or renal P transport disorders (familial hypophosphatemic rickets) may lead to P deficiency in later infancy. Mutations in FGF23 are the cause of autosomal dominant hypophosphatemic rickets ; and in dentin matrix protein 1 (DMP1) are a cause of autosomal recessive hypophosphatemia. Mutations in the P-regulating gene (PHEX) occur in XLH, the most frequent form of renal P wasting. In addition, low serum P may also occur in extracellular to intracellular shifts from respiratory alkalosis. In the case of cellular shifts, total body P may not be depleted.
Clinical Presentation
P deficiency is accompanied by weakness, malaise, and anorexia. Bone pain, frequently occurring in growing children with hypophosphatemic rickets, is not present in neonates with hypophosphatemia. There are no easily recognizable symptoms. Signs of hypophosphatemia are usually only seen with moderate to severe hypophosphatemia. Severe hypophosphatemia has deleterious effects on muscular, cardiac, pulmonary, hematologic, and nervous system function, including muscle weakness, poor ventricular function, and difficulty weaning from a ventilator (poor tissue oxygenation), essentially because P depletion leads to a decrease in high-energy substrate availability and respiratory muscle function (impaired diaphragm contractility). The mechanism of muscle weakness and red blood cell dysfunction caused by hypophosphatemia may relate to the role of P in intracellular signal transduction and synthesis of ATP or creatine phosphate. Other manifestations are hemolysis; impaired platelet and white blood cell function; rhabdomyolysis; and in rare cases, neurologic disorders, peripheral neuropathy, convulsions, and coma.
Physical examination of VLBW infants with P deficiency is usually benign. Clinical evidence of osteopenia or rickets is present infrequently, and pathologic fractures of the ribs or limbs are late occurrences. The clinician is, therefore, dependent on biochemical tests and radiography to detect early bone disease.
Therapeutic Approaches
Because hypophosphatemia is the most prominent feature of P deficiency in preterm infants, extra P supplement has been given; however, hypocalcemia occurs after P supplementation alone, and about 66% of supplemented P is lost in urine. In addition, these infants are Ca deficient as well as P deficient; P-induced decreases in serum Ca may lead to secondary hyperparathyroidism; large amounts of supplemental P cannot be used and are wasted in urine. Thus, both P and Ca supplements (and not P alone) are necessary to avert hypocalcemia and to allow adequate bone mineral accretion. Recent recommendation of enteral Ca supplementation is 120 to 200 mg/kg/day, and the recommendation for P is 70 to 120 mg/kg/day with vitamin D 400 IU/day, which allows normophosphatemia and normocalciuria with normal vitamin D status.
Provision of P and Ca in total PN solution with a Ca-to-P ratio of 1.3 to 1.7 to 1 (500–600 mg of Ca/L and 400–450 mg of P/L of PN solution) allows optimal mineral retention for preterm infants, reaching intakes of Ca of 100 mg/kg/day and P of 65 mg/kg/day. Sodium glycerophosphate (1 mmol/L organic P) improve solubility in PN. P accumulation in preterm infants is correlated with a protein content: N-to-P ratio of 17 to 1 by weight (P retained = Ca retained/2.3 + N retained/17). Adequate supply of protein is also important for normal bone formation and mineralization. Considering optimal N retention of 350 to 400 mg/kg/day and provision of 100 mg/kg/day of Ca, the P supply must reach 65 mg/kg/day corresponding to a Ca-to-P ratio close to 1.5.
In hypophosphatemia with high serum Ca, supplements of 0.5 to 1.0 mM/kg/day (16–31 mg/kg/day) of elemental phosphate in divided oral doses may normalize serum P and lower serum Ca; parenteral phosphate, however, should be avoided in severe hypercalcemia (serum total Ca >3 mmol/L [12 mg/dL]) unless hypophosphatemia is severe (<1.5 mg/dL) because extraskeletal calcification theoretically may occur.
Neonatal Hyperphosphatemia
Definition
Hyperphosphatemia is a serum level greater than 3.3 mmol/L (8 mg/dL), which reflects P overload.
Etiology and Pathophysiology
Hyperphosphatemia occurs from medication errors, increased intestinal absorption, decreased renal excretion, and cellular release or rapid intracellular to extracellular shifts. Increased tissue P release is commonly seen in profound catabolic states.
In steady state, serum P is maintained primarily by the ability of the kidneys to excrete dietary P, with efficient renal excretion. However, if acute P load is given over several hours, transient hyperphosphatemia will ensue. In addition to absorption of excess P, volume contraction (caused by diarrhea) and renal insufficiency (caused by volume depletion and decreased renal perfusion) may contribute to hyperphosphatemia and hypocalcemia.
Hyperphosphatemia is frequently the result of increased parenteral unbalanced administration of Ca, P, and Mg or a medication error (sodium phosphate instead of Ca gluconate). Increased intestinal absorption is generally caused by a large oral P intake and a vitamin D overdose in preterm infants or an erroneous medical prescription (oral phosphate Joulie’s solution instead of alkaline solution) in newborn infants with renal insufficiency.
Life-threatening hyperphosphatemia occurs after inadvertent administration of a hypertonic Fleet enema (60 mL of pediatric formula containing 105.4 mEq of P and 130.7 mEq of Na) in newborn infants, causing hyperphosphatemia and hypocalcemia; an osmotically active high P concentration in the enema solution results in excess retention and toxicity. A phosphate-containing enema (Fleet Co, Lynchburg, Virginia) is particularly dangerous in renal insufficiency or bowel dysfunction (constipation), although even without predisposing factors, a P enema can result in severe toxicity if retained.
Infants receiving cow’s milk–derived formulas that contain high P (67–81 mg/dL of P) who have impaired renal excretion or hypoparathyroidism may develop hyperphosphatemia. Even in normal term infants, higher serum P and lower serum ionized Ca occur in the first week, versus breastfed infants, related to higher absolute P in formula and limited P excretion from low newborn GFR. The biochemical features of high serum P and low serum Ca can resemble those of pseudohypoparathyroidism because there may be resistance of the immature kidneys to PTH. Persistent hyperphosphatemia occurs almost exclusively in those with acute or chronic kidney disease.
Clinical Presentation
Acute hyperphosphatemia generally does not cause signs unless the patient has hypocalcemia. In patients given high bolus doses of P orally or rectally, symptomatic acute P intoxication occurs, presenting with severe life-threatening hyperphosphatemia and hypocalcemia; carpopedal spasm ; vomiting; apnea; cyanosis on mechanical ventilation; hypoactivity, severe dehydration, and shock ; depressed level of consciousness (lethargy); shallow, difficult respirations; and generalized seizure. These patients are unresponsive to multiple doses of lorazepam, but responsive only to IV Ca. Clinical signs of chronic hyperphosphatemia include ectopic mineralization of muscular and subcutaneous tissues.
Therapeutic Approaches
P intoxication is a life-threatening condition. Treatment of P-induced hypocalcemia involves increasing urinary P excretion with diuretics and hydration (isotonic solution 20 mL/kg) and administrating IV Ca in symptomatic patients (avoiding lethal cardiac instability despite concern of Ca and P deposition in the kidneys) or oral Ca as an enteral P binder (promoting fecal excretion); enteral feedings with a low-P formula or human milk; and reducing parenteral P administration.
Ca correction is required to prevent or treat potential severe adverse effects, including cardiovascular abnormalities (prolonged QT interval, specifically ST segment), tetany, and seizures. Ca chloride (IV) is generally used for acute therapy in severe cases because it contains the highest concentration of ionized Ca compared with other Ca salts. However, in patients with limited or poor venous access, IV Ca gluconate is preferred because of the potential risk of peripheral extravasation. Ca should be administered with caution and only to alleviate clinical signs related to hypocalcemic toxicity. In the presence of severe hyperphosphatemia, Ca replacement can lead to extraskeletal calcification, especially in the renal tubule.
Treatment of severe life-threatening hyperphosphatemia secondary to retention of an enema may include modalities to prevent further absorption (e.g., lavage). Additionally, dialysis or hemofiltration can be used to rapidly lower the P concentration in renally impaired oliguric patients. Because PD strongly depends on total dialysate turnover, continuous flow-through PD, a closed PD system using two sterile polyvinylpyrrolidone short-term urethral catheters (6 Fr in and 8 Fr out) manufactured in Brazil, has been successfully applied in neonatal enema-induced hyperphosphatemia (8-day-old newborn) after achieving hemodynamic stability with vigorous fluid resuscitation and vasoactive drugs.
In children with chronic renal failure, secondary hyperparathyroidism can be suppressed using mild dietary P reduction and high-dose P binders with small vitamin D supplementation. Ca carbonate is an effective P binder with no major side effects and is a drug of choice in correcting hyperphosphatemia and hyperparathyroidism in uremic children, although neonatal use of Ca carbonate has not been reported.
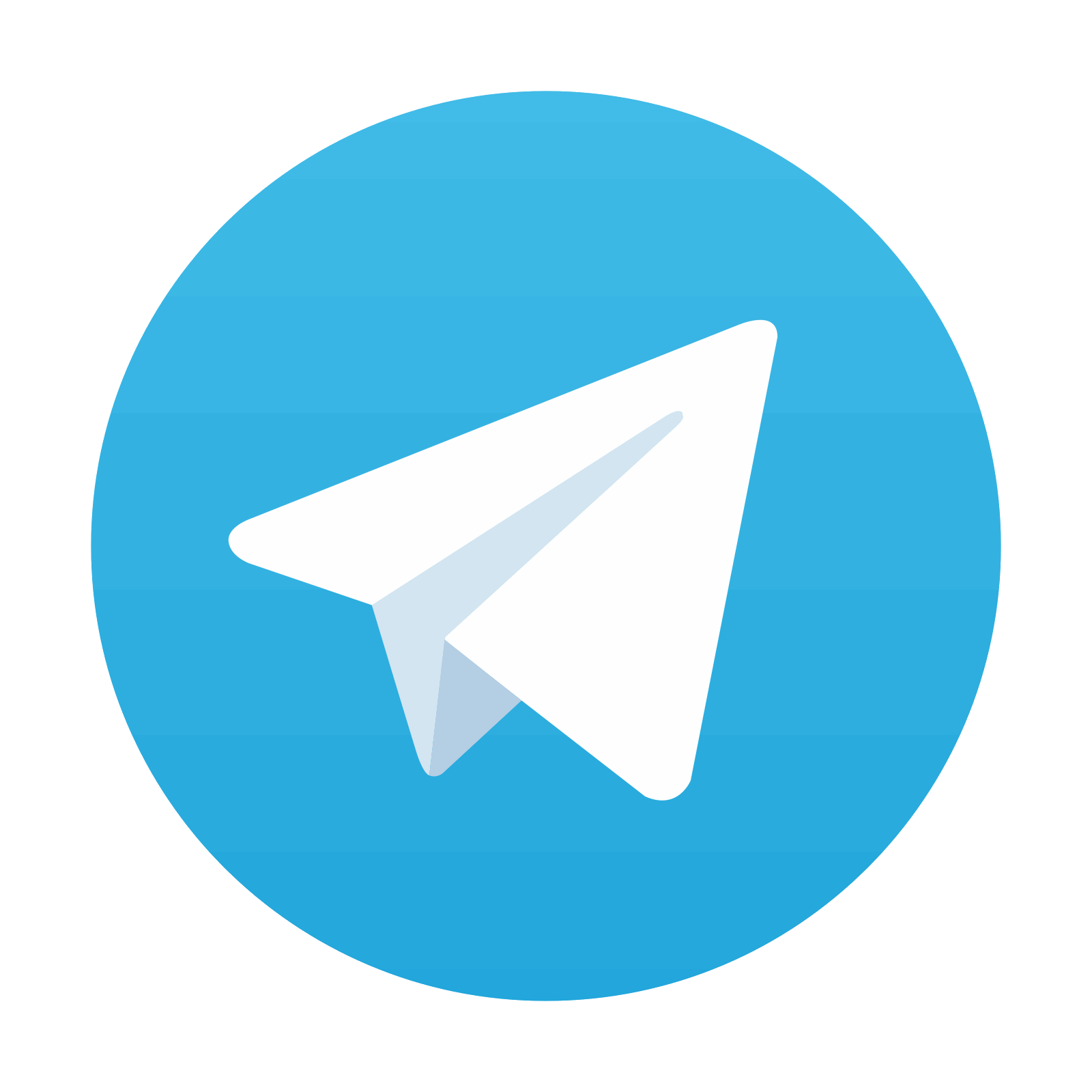
Stay updated, free articles. Join our Telegram channel

Full access? Get Clinical Tree
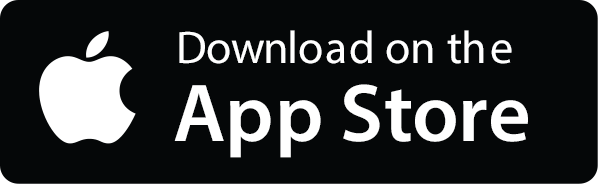
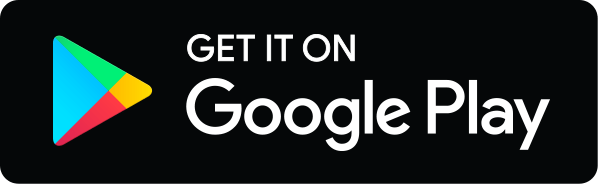