Abstract
Hereditary tubular transport disorders comprise a group of diseases that usually present in the neonatal period, lead to profound derangements in the homeostasis of electrolytes, minerals, or organic solutes in the body, and can be associated with significant morbidity. In the past two decades, remarkable progress has been made in our understanding of the molecular pathogenesis of hereditary tubulopathies. Molecular genetics and molecular biology studies have led to the identification of numerous tubular disease-causing mutations, have provided important insight into the defective molecular mechanisms underlying various tubulopathies, and have greatly increased our understanding of the physiology of renal tubular transport.
This chapter summarizes the general characteristics of hereditary tubular transport disorders, reviews the molecular pathophysiology and genetic aspects of the diseases, describes the clinical feature of the tubulopathies, and briefly summarizes their therapy. The focus of this chapter is on isolated tubulopathies involving an impairment in a single tubular function, which results from primary gene defects in transporters or channels operating along the renal tubule.
Despite the exciting progress made, numerous issues remain unsettled and warrant additional research on the molecular mechanisms and functional defects underlying the impaired transport in various tubulopathies. These studies may significantly improve our understanding of the mechanisms underlying renal salt homeostasis, urinary mineral excretion, and blood pressure regulation in health and disease. The identification of the molecular defects in inherited tubulopathies may provide a basis for future design of targeted therapeutic interventions and, possibly, strategies for gene therapy of these complex disorders.
Keywords
tubular epithelium, membrane transport, renal solute handling, acid-base balance, gene expression, mutation, blood pressure
- •
Proximal Tubule
- •
Loop of Henle
- •
Distal Convoluted Tubule
- •
Collecting Duct
- •
Conclusion
Hereditary tubular transport disorders comprise a group of diseases that lead to profound derangements in the homeostasis of electrolytes, minerals, or organic solutes in the body and can be associated with significant morbidity.
For decades, the study of inherited tubular transport disorders has focused on the physiologic and metabolic alterations leading to impaired solute handling by the tubular epithelial cell. Over the past two decades, the breakthrough in molecular biology and molecular genetics has provided the tools to investigate hereditary tubulopathies at the molecular level. As a result, exciting discoveries have been made, and the underlying molecular defects in many of these disorders have been defined. The molecular study of hereditary tubulopathies has been important not only in clarifying the genetic basis of these disorders but also in providing new and important insight into the function of specific transport proteins and into the physiology of renal tubular reclamation of solutes.
Generally, tubular transport disorders are subdivided into two large groups: (1) primary isolated tubulopathies, which are mostly hereditary and involve an impairment in a single tubular function; and (2) generalized tubulopathies, which are hereditary or acquired and are caused by complex tubular derangements involving more than one transport system. A variety of primary inherited tubulopathies alter specific renal epithelial transport functions. In most instances, the change in transport function leads to the loss of an essential substance in the urine and either impaired homeostasis of this substance in the body (as in renal tubular acidosis [RTA] or Bartter syndrome) or precipitation of the substance in the kidney (as in cystinuria or hypercalciuria). In some of these disorders, however, the defect in tubular function leads to accumulation of a substance in the body (as in pseudohypoaldosteronism type II).
Hereditary tubulopathies can affect children at all ages, but usually, children with these disorders present in the neonatal period or in the first year of life. Clinical manifestations of hereditary tubulopathies are commonly nonspecific and may include failure to thrive, stunted growth, poor feeding, recurrent vomiting, diarrhea, constipation, polyuria, polydipsia, or recurrent febrile episodes. In some instances, however, more specific manifestations such as rickets, urolithiasis, or hypertension aid in the diagnosis of a specific tubulopathy. In most of these disorders, the principle of therapy is replacement of the substance lost in the urine or prevention of precipitation of the substance in the kidney. Some of the tubulopathies (e.g., isolated glycosuria) are benign and require no therapy. In addition to a detailed history and careful examination of the child, simultaneous and accurate assessment of the serum and urine concentration of the substance involved in the tubulopathy holds the key to the correct diagnosis. Renal ultrasonography and bone radiography are helpful studies in most tubulopathies.
This chapter summarizes the general characteristics of hereditary tubular transport disorders, reviews the molecular pathophysiology and genetic aspects of the diseases, describes the clinical feature of the tubulopathies, and briefly summarizes their therapy. The focus of this chapter is on disorders resulting from primary gene defects in transporters or channels operating along the renal tubule. Some tubular transport disorders secondary to defects in receptors (e.g., as Ca 2+ -sensing receptor or antidiuretic hormone receptor), enzymes (e.g., with no K [lysine] serine-threonine protein kinases, WNKs), or regulatory proteins (e.g., melanoma-associated antigen D2, MAGE-D2) resulting in isolated tubulopathies are also discussed. Generalized tubulopathies involving several transport systems (e.g., Fanconi syndrome) or resulting from mitochondrial cytopathies are not discussed or only briefly mentioned. Inherited tubular disorders of calcium, magnesium, and phosphorus are discussed in Chapter 20 . In Tables 19.1 through 19.4 , the disorders reviewed are summarized and grouped by the nephron segment affected.
Disorder | Defective Gene | Locus | Defective Protein | Mode of Inheritance | Localization of Defect | Clinical Features | OMIM Number a |
---|---|---|---|---|---|---|---|
Isolated proximal RTA (type 2) | SLC4A4 | 4q21 | Na + -HCO 3 − cotransporter, NBC1 | AR | Basolateral | Normal anion gap metabolic acidosis, failure to thrive, hypokalemia, polyuria, polydipsia, dehydration, muscle weakness, ocular abnormalities | 604278 |
Classic cystinuria | |||||||
| SLC3A1 SLC7A9 | 2p163 19q13.1 | rBAT b 0,+ AT | AR Incomplete AR | Luminal Luminal | Urinary stones, obstruction, infection | 220100 |
Lysinuric protein intolerance | SLC7A7 | 14q11 | y + LAT-1 | AR | Basolateral | Failure to thrive, protein intolerance, vomiting, hypotonia, hyperammonemia, seizures, coma | 222700 |
Hartnup disease | SLC6A19 | 5p15.33 | AA transport system B 0 | AR | Luminal | Skin rash, cerebellar ataxia, psychiatric illnesses | 234500 |
Isolated hereditary glycosuria | SLC5A2 | 16p11.2 | SGLT2 | AR | Luminal | None | 233100 |
Fanconi-Bickel syndrome | SLC2A2 | 3q26.1 | GLUT2 | AR | Basolateral | Failure to thrive, proximal tubulopathy, hepatomegaly, fasting hypoglycemia, postprandial hyperglycemia | 227810 |
a Online Mendelian Inheritance in Man (database at http://www-ncbi-nlm-nih-gov.easyaccess2.lib.cuhk.edu.hk/Omim ).
Disorder | Defective Gene | Locus | Defective Protein | Localization of Defect | Mode of Inheritance | Clinical Features | OMIM Number a |
---|---|---|---|---|---|---|---|
Antenatal Bartter syndrome (type I) | SLC12A1 | 15q21 | Na + -K + -2Cl − cotransporter, NKCC2 | Luminal | AR | Renal salt wasting, hypokalemia, hypochloremic metabolic alkalosis, failure to thrive, polyuria, dehydration, muscle weakness, hypercalciuria | 601678 |
Antenatal Bartter syndrome (type II) | KCNJ1 | 11q24 | K + channel, ROMK | Luminal | AR | Renal salt wasting, hypokalemia, hypochloremic metabolic alkalosis, failure to thrive, polyuria, dehydration, muscle weakness, hypercalciuria | 241200 |
Classic Bartter syndrome (type III) | CLCNKB | 1p36 | Cl − channel, ClC-Kb | Basolateral | AR | Renal salt wasting, hypokalemia, hypochloremic metabolic alkalosis, failure to thrive, polyuria, dehydration, muscle weakness, ± hypercalciuria | 602023 |
Bartter syndrome with deafness (type IV) | BSND | 1p31 | Barttin (β subunit of ClC-Ka/ClC-KB) | Basolateral | AR | Renal salt wasting, hypokalemia, hypochloremic metabolic alkalosis, failure to thrive, polyuria, dehydration, muscle weakness, ± hypercalciuria, chronic renal failure, sensorineural deafness | 602522 |
Autosomal dominant hypocalcemia with Bartter syndrome (type V) | CASR | 3q21 | CaSR | Basolateral | AD | Renal salt wasting, hypokalemia, hypochloremic metabolic alkalosis, failure to thrive, polyuria, dehydration, muscle weakness, hypercalciuria | 601198 |
Transient antenatal Bartter syndrome | MAGED2 | Xp11 | MAGE-D2 | Luminal | X-linked | Severe polyhydramnios, transient renal salt wasting, hypokalemia, hypochloremic metabolic alkalosis, polyuria, dehydration, hypercalciuria | 300971 |
a Online Mendelian Inheritance in Man (database at http://www.ncbi.nlm.hih.gov/Omim ).
Disorder | Defective Gene | Locus | Defective Protein | Localization of Defect | Mode of Inheritance | Clinical Features | OMIM Number a |
---|---|---|---|---|---|---|---|
Gitelman syndrome | SLC12A3 CLCNKB | 16q13 1p36 | Na + -Cl − cotransporter, TSC (NCCT) Cl − channel, ClC-Kb | Luminal Basolateral | AR | Mild renal salt wasting, hypokalemia, hypochloremic, metabolic alkalosis, muscle weakness, tetany, hypomagnesemia, hypocalciuria | 263800 |
EAST, SESAME syndrome | KCNJ10 | 1q23 | K + channel, Kir4.1 | Basolateral | AR | Epilepsy, ataxia, sensorineural deafness, Gitelman-like tubulopathy | 612780 |
Pseudohypoaldosteronism type 2 (Gordon syndrome) | WNK4 WNK1 KLHL3 CUL3 | 17q21 12p13 5q31 2q36 | WNK4 WNK1 Kelch-like 3 Cullin 3 | Cytoplasmic/luminal | AD | Thiazide-sensitive hypertension, hyperkalemia, hyperchloremic metabolic acidosis | 601844 605232 605775 614496 |
a Online Mendelian Inheritance in Man (database at http://www.ncbi.nlm.hih.gov/Omim ).
Disorder | Defective Gene | Locus | Defective Protein | Cell Type Involved | Localization of Defect | Clinical Features | OMIM Number a |
---|---|---|---|---|---|---|---|
Distal RTA (Type 1) | |||||||
AD distal RTA | SLC4A1 | 17q21-22 | AE1 | α intercalated | Basolateral | Mild metabolic acidosis, hypokalemia, hypercalciuria, hypocitraturia, nephrolithiasis, nephrocalcinosis, rickets/osteomalacia | 179800 |
AR distal RTA | SLC4A1 | 17q21-22 | AE1 | α intercalated | Basolateral | Metabolic acidosis, hemolytic anemia (Southeast Asia only) | 602722 |
AR distal RTA with deafness | ATP6V1B1 | 2p13 | B 1 subunit of H + -ATPase | α intercalated | Luminal | Early metabolic acidosis, nephrocalcinosis, vomiting, dehydration, growth retardation, rickets, bilateral sensorineural hearing loss | 267300 |
AR distal RTA without or with late onset deafness | ATP6VOA4 | 7q33 | a4 subunit of H + -ATPase | α intercalated | Luminal | Early metabolic acidosis, nephrocalcinosis, vomiting, dehydration, growth retardation, rickets, late onset sensorineural hearing loss or normal hearing | 602772 |
Mixed proximal and distal RTA (type 3) | CA2 | 8q22 | Carbonic anhydrase II | Proximal tubule and α intercalated | Cytoplasm | Metabolic acidosis, hypokalemia, osteopetrosis, blindness, deafness, early nephrocalcinosis | 259730 |
RTA Type 4 | |||||||
AD pseudohypoaldosteronism type 1 | NR3C2 | 4q31 | Mineralocorticoid receptor | Principal | Cytoplasm | Mild hyponatremia, hyperkalemia, metabolic acidosis | 177735 |
AR pseudohypoaldosteronism type 1 | SCNN1A SCNN1B SCNN1C | 12p13,1 16p13 16p12 | Na + channel ENaC (α,β,γ subunits) | Principal | Luminal | Neonatal salt wasting, severe dehydration, hyperkalemic metabolic acidosis, hyponatremia, respiratory disease | 264350 |
Nephrogenic Diabetes Insipidus | |||||||
X-linked NDI | AVPR2 | Xq28 | Vasopressin type-2 receptor, V2R | Principal | Basolateral | Polydipsia, polyuria, hyposthenuria, hypernatremic dehydration, failure to thrive, neurologic deficit | 304800 |
AR or AD NDI | AQP2 | 12q13 | H 2 O channel, AQP2 | Principal | Luminal | Polydipsia, polyuria, hyposthenuria, hypernatremic dehydration, failure to thrive, neurologic deficit | 125800 |
Nephrogenic syndrome of inappropriate diuresis | AVPR2 | Xq28 | Vasopressin type-2 receptor, V2R | Principal | Basolateral | Hyponatremia, seizures, hypertonic urine | 300539 |
a Online Mendelian Inheritance in Man (database at http://www.ncbi.nlm.hih.gov/Omim ).
Proximal Tubule
Proximal Renal Tubular Acidosis
General Characteristics
Proximal renal tubular acidosis (pRTA; RTA type 2) is characterized by normal anion gap, hyperchloremic metabolic acidosis caused by impaired capacity of the proximal tubule to reabsorb HCO 3 − ( Fig. 19.1 ). Thus, at normal plasma HCO 3 − concentration, large amounts of HCO 3 − (>15% of the filtered load) escape proximal reabsorption and reach the distal tubule. This load overwhelms the limited capacity of the distal tubule to reabsorb HCO 3 − , substantial bicarbonaturia occurs, urine pH increases, net acid secretion ceases, and metabolic acidosis develops. This HCO 3 − wasting is a transient phenomenon, and when the serum HCO 3 − level stabilizes in the acidemia range, the smaller amounts of HCO 3 − lost in the proximal tubule are completely reabsorbed by the distal tubule, and urine pH decreases to less than 5.5. In pRTA, serum K + level is usually diminished. Hypokalemia develops because increased delivery of Na + to the distal nephron results in enhanced secretion of K + in the principal cell of the cortical collecting duct (CCD), and mild volume depletion secondary to Na + loss results in secondary hyperaldosteronism that increases K + secretion.

pRTA occurs either as a manifestation of a generalized proximal tubular dysfunction (Fanconi syndrome) or as an isolated entity. Inheritance of isolated pRTA is autosomal recessive and occurs consistently in association with ocular abnormalities, including glaucoma, band keratopathy, and cataracts (see Table 19.1 and Fig. 19.1 ). Additional manifestations include short stature, calcification of the basal ganglia, and mental retardation (see later discussion).
Molecular Pathophysiology
Normally, most (80%–90%) of the filtered load of HCO 3 − is reabsorbed in the proximal tubule. Several membrane transport proteins participate in acid–base handling in the proximal tubule (see Fig. 19.1 ). H + and HCO 3 − are formed in the proximal tubular cell as a result of the action of intracellular carbonic anhydrase II (CAII). H + efflux from cell to lumen occurs via the apical Na + /H + exchanger (NHE3) and to a small extent via the apical H + -ATPase pump. HCO 3 − exit to blood is mediated by the basolateral membrane Na + -HCO 3 − cotransporter (NBC1).
The Na + /HCO 3 − cotransporter NBC1 (see Fig. 19.1 ), encoded by the SLC4A4 gene located on chromosome 4q21, has been implicated in autosomal recessive proximal RTA. NBC1 belongs to the HCO 3 − transporter superfamily, to which the Cl − /HCO 3 − exchanger also belongs. Igarashi et al. identified two homozygous missense mutations in kidney NBC1 in two individuals with autosomal recessive pRTA and ocular abnormalities (see Table 19.1 ). Both patients had cataracts, glaucoma, and band keratopathy. A number of other mutations have subsequently been described. In addition to reduced functional activity, defects of intracellular trafficking have been demonstrated for some of these mutations. Severe isolated proximal RTA was recently described in NBC1 W561X knock-in mouse. It is possible that defective corneal NBC1 function in patients with isolated pRTA results in impaired HCO 3 − transport, which in turn leads to abnormal calcium carbonate deposition in the cornea and band keratopathy.
Clinical Features
The most prominent clinical feature of pRTA is failure to thrive. Other manifestations, which are related to untreated hypokalemia, include polyuria, polydipsia, dehydration, vomiting, anorexia, constipation, and muscle weakness (see Table 19.1 ). Hypercalciuria, nephrocalcinosis, and nephrolithiasis typically are not observed. Metabolic bone disease usually occurs in patients with Fanconi syndrome and is attributed to hypophosphatemia and impaired vitamin D metabolism, but may also be induced by the bone Ca 2+ -depleting effect of chronic acidosis in isolated pRTA.
The diagnosis of pRTA is usually straightforward and can be based on several simple laboratory data. These include (1) normal anion gap, hyperchloremic metabolic acidosis; (2) hypokalemia; (3) low urine pH during acidemia; and (4) a negative urinary anion gap (calculated as [Na + ] + [K + ] − [Cl − ]) indicating substantial urinary ammonium concentration, in the absence of extrarenal losses of HCO 3 − such as gastroenteritis (as opposed to the positive urinary anion gap in distal RTA; see later discussion). Although usually not necessary, demonstration of increased (>15%) fractional excretion of HCO 3 − by the HCO 3 − titration curve can support the diagnosis. Children with pRTA require large doses of alkali (up to 20 mEq/kg per day). Hypokalemia should be treated by correcting hypovolemia and by using KCl supplements.
Hereditary Aminoacidurias
Only negligible amounts of amino acids are normally present in the final urine, reflecting very efficient reabsorption mechanisms for these organic solutes in the proximal tubule. Aminoacidurias are a group of disorders in which a single amino acid or a group of amino acids are excreted in excess amounts in the urine. The defective tubular reabsorption is assumed to result from a genetic defect in a specific transport system that directs the reabsorption of these amino acids under normal conditions. Some of these disorders also involve a similar transport abnormality in the intestine. As opposed to inborn errors of amino acid metabolism, in which plasma levels of amino acids are elevated resulting in overflow aminoaciduria, plasma levels of amino acids in hereditary aminoacidurias are largely normal. The aminoacidurias are generally categorized into five major groups according to the group-specific transport pathway presumed to be affected. Discussed in this chapter are the cationic aminoacidurias, classic cystinuria, and lysinuric protein intolerance (LPI), as well as the neutral aminoaciduria, Hartnup disease (see Table 19.1 and Fig. 19.1 ). The genetic defects in these three membrane transport disorders, which are associated with significant morbidity, have been identified.
Classic Cystinuria
General Characteristics
Cystinuria is a disorder of amino acid transport characterized by excessive urinary excretion of cystine and the dibasic amino acids lysine, arginine, and ornithine. The pathogenic mechanism of cystinuria is defective transepithelial transport of these amino acids in the proximal tubule and the small intestine. The high-affinity, low-capacity amino acid transport system shared by cystine and the dibasic amino acids (i.e., b 0,+ ; Fig. 19.2 ) is defective in classic cystinuria.

The very low solubility of cystine in the urine results in cystine stone formation in homozygous patients. Urinary cystine calculi may produce considerable morbidity, including urinary obstruction; colic; infection; and in severe cases, loss of kidney function. Cystinuria accounts for 1% to 2% of all urolithiasis and 6% to 8% of urolithiasis in children.
Classic cystinuria is inherited in an autosomal recessive fashion. It is a common disorder with an overall prevalence of 1 in 7000 to 1 in 15,000 and an estimated gene frequency of 0.01. A very high prevalence, 1 in 2500, is observed in Israeli Jews of Libyan origin.
Cystinuria has been classified into three phenotypes based on the degree of intestinal uptake of cystine by homozygotes and the level of urinary dibasic amino acids in heterozygotes. Type I cystinuria is inherited as an autosomal recessive trait, and obligate heterozygotes have normal urinary amino acid profiles. In contrast, obligate heterozygotes for type II and type III cystinuria show various degrees of hyperexcretion of cystine and dibasic amino acids in the urine. In addition, genetic compounds of cystinuria, such as types I/III, can occur.
Molecular Pathophysiology
Type I cystinuria is caused by mutations in the gene SLCA3A1 localized to chromosome 2p21. The gene encodes a protein termed rBAT, which constitutes the heavy subunit of the proximal tubular, brush-border membrane-bound, heteromeric dibasic amino acid transporter b 0,+ (see Fig. 19.2 ). To date, more than 130 different rBAT mutations have been reported in patients with type I cystinuria. These mutations include nonsense, missense, splice site, frameshift mutations, and large deletions. Cystinuria types II and III are caused by mutations in the gene SLC7A9, which is localized to chromosome 19q13.1 and encodes the b 0,+ AT protein that constitutes the light subunit of the heteromeric amino acid transporter b 0,+ (see Fig. 19.2 ). To date, more than 95 different mutations have been identified in nontype I cystinuria patients. Recently, the International Cystinuria Consortium (ICC) has introduced an additional classification of cystinuria subtypes based on genotype rather than phenotype. This new classification includes: Type A—due to two mutations on SLCA3A1 on chromosome 2; Type B—due to two mutations on SLC7A9 on chromosome 19; and Type AB—due to one mutation on each SLCA3A1 and SLC7A9 (compound heterozygote).
Clinical Features
The simplest diagnostic test in cystinuria is the microscopic examination of the urinary sediment of a freshly voided morning urine. The presence of typical flat hexagonal cystine crystals is diagnostic. The best screening procedure is the cyanide–nitroprusside test. The definite test is a measurement of cystine and the dibasic amino acids concentrations by ion exchange chromatography.
Cystine stones are radiopaque because of the density of the sulfur molecule, and on radiography, they appear smooth. Cystine also may act as nidus for calcium oxalate, so mixed stones may be found.
The disease usually presents with renal colic. Occasionally, infection, hypertension, or renal failure may be the first manifestation (see Table 19.1 ). Most patients have recurrent stone formation. Cystinuric patients who receive a kidney transplant have normal urinary cystine and dibasic amino acid excretion after transplantation.
Treatment
Cystine crystalluria occurs when the cystine content of the urine exceeds 300 mg/L at a pH of 4.5 to 7.0. Cystine solubility increases sharply at a urine pH above 7.0. The major therapeutic approaches to cystinuria are designed to increase the solubility of cystine, reduce excretion cystine, and convert cystine to more soluble compounds. Therapies used in the management of cystinuria include the following:
- 1.
Increased oral fluid intake to increase urine volume and cystine solubility. Because patients with cystinuria excrete 0.5 to 1.0 g/day of cystine, intake of at least twice normal maintenance fluid volume for age could be required to keep the urinary cystine concentration below 300 mg/L.
- 2.
Oral alkali in addition to high fluid intake to further increase cystine solubility in the urine. A urine pH of 7.5 to 8.0 can be maintained by the provision of 1 to 2 mEq/kg per day of bicarbonate or citrate in divided doses. Because high sodium intake increases cystine excretion, potassium citrate is preferred. Because urine alkalinization may result in formation of mixed Ca 2+ -containing stones, adherence to high fluid intake is crucial.
- 3.
Na + restriction to reduce cystine excretion. Dietary Na + restriction is recommended in patients with cystinuria because urinary excretion of cystine and dibasic amino acids correlates with urinary Na + excretion.
- 4.
Pharmacologic therapy to increase cystine solubility and decrease cystine excretion. The sulfhydryl-binding compound d -penicillamine (β-dimethylcysteine) leads to the formation of the mixed disulfide penicillamine–cysteine after a disulfide exchange reaction. This mixed disulfide is far more water soluble than cystine. Unfortunately, penicillamine produces serious side effects, including rashes, fever, arthralgia, nephrotic syndrome, pancytopenia, and loss of taste. Mercaptopropionyl glycine (MPG), another agent undergoing a disulfide exchange reaction, is as effective as d -penicillamine in the treatment of patients with cystinuria. Because of the lower toxicity of MPG, this compound is the pharmacologic agent of choice in the therapy of cystinuria. It has been proposed that meso-1,3 dimercaptosuccinic acid (DMSA), an additional compound forming disulfide linkage with cysteine, might also be a useful therapeutic agent in those with cystinuria. Recently, α-lipoic acid has been shown to increase urinary cystine solubility and to prevent cystine urolithiasis in a mouse model of cystinuria. This widely used nutritional supplement with few adverse side effects is an attractive candidate for assessment in individuals with cystinuria.
- 5.
Urologic procedures that have been used to treat cysteine stones include chemolysis of stones by irrigation through a percutaneous nephrostomy, extracorporeal shockwave lithotripsy, and lithotomy.
Lysinuric Protein Intolerance
General Characteristics
LPI is a rare autosomal recessive disorder characterized by excessive urinary excretion of dibasic amino acids (especially lysine), normal cystine excretion, and poor intestinal absorption of dibasic amino acids. Plasma values of dibasic amino acids are subnormal. The disease is relatively common in Finland, where the prevalence of the disease is 1 in 60,000, and in Italy. Homozygous patients show massive dibasic aminoaciduria, as well as hyperammonemia, after a protein overload. The clinical manifestations in homozygotes for LPI are protein malnutrition and postprandial hyperammonemia. They include failure to thrive, marked protein intolerance, vomiting, diarrhea, hepatosplenomegaly, muscle hypotonia, interstitial lung disease, osteoporosis, seizures, and coma (see Table 19.1 ).
Molecular Pathophysiology
The pathogenic mechanism of LPI appears to be defective, high-affinity, dibasic amino acid transport system y + L (see Fig. 19.2 ) at the basolateral membrane of renal and intestinal epithelial cells, resulting in impaired efflux of these amino acids from cell to interstitium. Nonepithelial cells such as hepatocytes, granulocytes, and cultures akin fibroblasts from patients with LPI also show impaired transport of dibasic amino acids. The defective hepatic transport of dibasic amino acids is associated with disturbances in the urea cycle and consequent hyperammonemia.
LPI is caused by mutations in the gene SLC7A7 encoding y + LAT-1, a member of the family of light subunits that combine with 4F2hc (a heavy subunit) to form heteromeric amino acid transporters. The 4F2hc/y + LAT-1 transporter has been shown to have the activity of amino acid transport system y + L that is responsible for the efflux of basic amino acids at the basolateral plasma membrane of epithelial cells (see Fig. 19.2 ). The SLC7A7 gene is localized to chromosome 14q11-13. To date, approximately 50 SLC7A7 mutations, spread along the entire gene, have been found in LPI patients from different ethnic groups.
Treatment
Therapy of patients with LPI consists of protein restriction to prevent hyperammonemia, as well as oral supplements of arginine, ornithine, and (most important) citrulline. Administration of the latter amino acid, which corrects the hepatic deficiency in ornithine and arginine, results in clinical improvement and catch-up growth.
Hartnup Disease
General Characteristics
Hartnup disease, which may have afflicted Julius Caesar and his family, was first recognized in two siblings in England in 1956. This disease is characterized by intestinal malabsorption and massive aminoaciduria of the neutral monoamino monocarboxylic amino acids alanine, serine, threonine, valine, leucine, isoleucine, phenylalanine, tyrosine, tryptophan, histidine, glutamine, and asparagine (see Table 19.1 ). Most patients also have increased excretion of indolic compounds that originate in the gut from bacterial degradation of tryptophan. Transport of other neutral amino acids, including cystine, imino acids, glycine, and β-amino acids, is unaffected. The disease is inherited as an autosomal recessive trait and has an estimated incidence of 1 in 20,000 live births. Heterozygotes have normal urinary acid excretion under physiologic conditions. Clinical features in homozygotes may include photosensitive rash, cerebellar ataxia, and a variety of psychiatric manifestations resembling the features of pellagra (see Table 19.1 ). These pellagra-like manifestations are primarily caused by intestinal malabsorption and urinary loss of tryptophan, an amino acid that is required for niacin synthesis. The diagnosis should be suspected in any patient with pellagra who has no history of niacin or nicotinamide deficiency and should be made by chromatographic analysis of the urine.
Molecular Pathophysiology
A defect in the broad-specificity neutral, α-amino acid transport mechanism in the renal and intestinal brush-border membrane was presumed to be the pathogenic mechanism underlying this disorder. The transport characteristics and the epithelial distribution of the Na + -dependent neutral amino acid transport B o (see Fig. 19.2 ) have led to the conclusion that this transporter is the defective one in Hartnup disorder. In 2001, the gene responsible for Hartnup disease was localized to chromosome 5p15. Subsequently, Bröer’s group cloned from the syntenic region in the mouse the B o AT1 (SLC6A19) gene. This SLC6 gene encodes a Na + -dependent neutral amino acid transporter, which is expressed in the brush-border membrane of the early proximal tubule and the intestine and corresponds to the B o transport system.
In 2004, two groups cloned the human SLC6A19 gene from chromosome 5p15 and have found several mutations in this gene in British, Japanese, and Australian patients with Hartnup disease, thereby identifying SLC6A19 as the disease-causing gene. To date, a total of 21 mutations have been identified that cause Hartnup disorder. Interestingly, it has been demonstrated that most of the probands with Hartnup disease analyzed so far display allelic heterogeneity in that they are compound heterozygotes for the SLC6A19 mutation.
Treatment
Patients with Hartnup disease respond well to oral therapy with 40 to 100 mg/day of nicotinamide. Oral administration of tryptophan ethyl ester, a lipid-soluble form of tryptophan, has been shown to increase serum tryptophan and reverse clinical symptoms in patients with Hartnup disease.
Hereditary Glycosurias
Glycosurias are a group of disorders in which specific defects in glucose transporters in the renal tubule result in excretion of significant quantities of glucose in the urine. This group includes hereditary isolated glycosuria and Fanconi-Bickel syndrome (see Table 19.1 and Fig. 19.3 ).

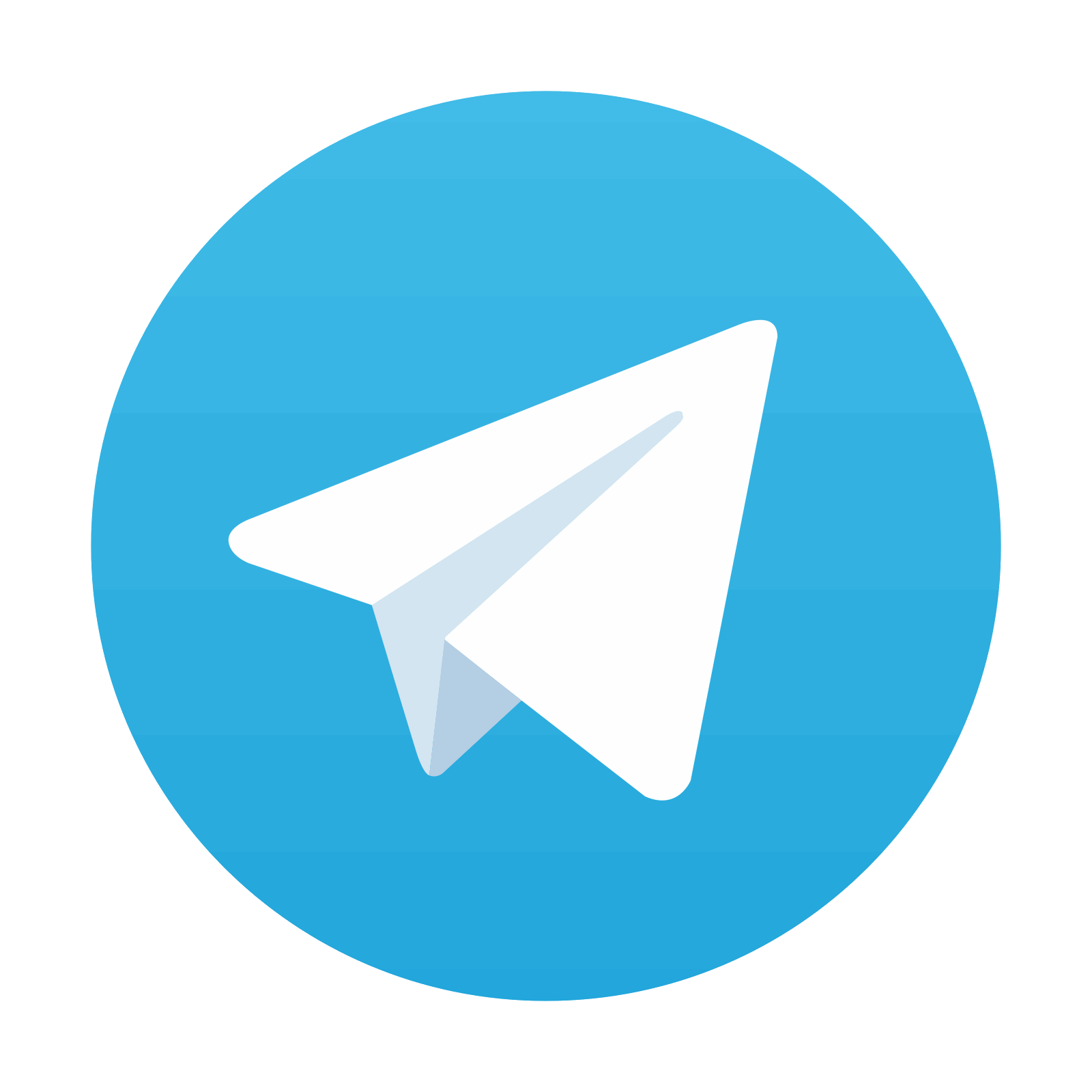
Stay updated, free articles. Join our Telegram channel

Full access? Get Clinical Tree
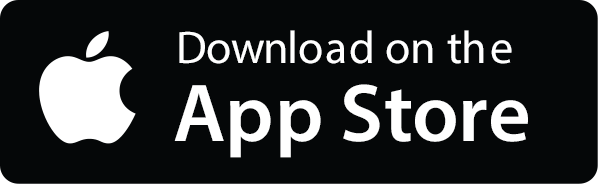
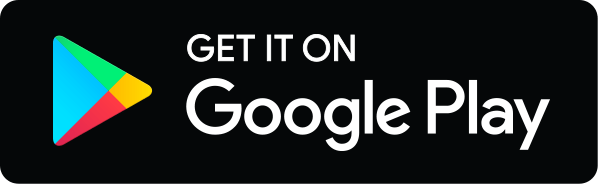
