Abstract
Ultrafiltration of plasma across permselective glomerular capillaries and urine formation start around the 10th week of gestation and increase throughout prenatal and postnatal life. The rapid development of glomerular filtration rate (GFR) is the consequence of an increase in the effective filtration pressure, an increase in the filtering area and permeability of the glomerular capillaries, and a decrease in the renal vascular resistance. Vasoactive factors regulate GFR and renal blood flow, during and after maturation. Interference with the two main factors, the prostaglandins and angiotensin II, affects both the organic and the functional development of GFR. The rate of filtration can be assessed by various endogenous or exogenous glomerular markers, creatinine being the most frequently used agent. Because of the difficulty in accurately collecting urine for the assessment of the urinary clearance of the markers, derived methods without urine collection have been developed, with variable reliability. GFR can be affected by various stresses such as asphyxia, acidosis, hypotension, and hypovolemia. Nephrotoxic drugs can also impair GFR leading to renal failure. Several agents, such as loop diuretics, dopaminergic agents, or low-dose theophylline, have been used in the hope of protecting GFR; the latter agent appearing the most promising.
Keywords
maturation of GFR, assessment of GFR, markers of filtration, impairment of GFR, protection of GFR, prevention of renal insufficiency
- •
Development of Glomerular Filtration
- •
Assessment of Glomerular Filtration Rate
- •
Conditions and Factors That Impair Glomerular Filtration Rate
- •
Prevention of Oliguric States Caused by Low Glomerular Filtration Rate
Ultrafiltration of plasma across permselective capillaries is the first step in urine formation. This process starts with the development of the metanephros around the 10th week of gestation. The glomerular filtration rate (GFR) increases progressively throughout fetal and postnatal life, reaching the “adult” mature levels by 1 year of life. During the period of maturation, the function of the kidney is characterized by elevated renal vascular resistance (RVR), low arterial renal perfusion pressure, and low renal blood flow (RBF). The ultrafiltration process is maintained by a delicate balance between vasoconstrictor and counteracting vasodilator forces. This balance can be easily disturbed by various factors, resulting in a transient or permanent impairment in GFR. This chapter briefly reviews the maturation of glomerular filtration, discusses the techniques available to assess GFR in neonates, and describes factors and agents that can impair or protect maturing glomerular filtration.
Development of Glomerular Filtration
Glomerular ultrafiltration depends on the net ultrafiltration pressure, which is the difference between the hydrostatic and oncotic pressures across glomerular capillaries. The low perfusion pressure and low glomerular plasma flow account, at least in part, for the low levels of GFR present during gestation. For any given ultrafiltration pressure, GFR will depend on the rate at which plasma flows through the glomerular capillaries, as well as on the ultrafiltration coefficient (K f ). K f is a function of the total capillary surface area and of the permeability per unit of surface area.
During gestation, GFR increases in parallel with gestational age (GA), up to the end of nephrogenesis around the 35th week of gestation. This pattern of development reflects both an increase in the number of nephrons and the growth of existing nephrons. From the 35th week of gestation, the development of GFR slows down up to the time of birth ( Fig. 7.1 ). Postnatal maturation of renal function is characterized by a striking increase in GFR, the value of which doubles within the first 2 weeks of life ( Fig. 7.2 ). The velocity of this increase is somewhat slower in the most premature infants. An increase in both the net filtration pressure and the K f accounts for the large postnatal increase in GFR. The increase in the glomerular capillary surface area represents the main factor responsible for the increase in the K f . Additional maturational changes that may contribute to the postnatal maturation of GFR include an increase in pore size and glomerular hydraulic permeability and a decrease in both the afferent and efferent arteriolar resistance.




Vasoactive Factors
Several vasoactive agents and hormones modulate GFR and RBF. By acting on the arcuate arteries, interlobular arteries, and afferent and efferent arterioles, they regulate the glomerular hydrostatic pressure and the glomerular transcapillary hydraulic pressure gradient. These agents can also modify the K f by two mechanisms: a change in the capillary filtration area by contracting the mesangial cells and a change in hydraulic conductivity by decreasing the number or size (or both) of the filtration slit pores. The main vasoactive forces modulating single-nephron GFR (SNGFR) are listed in Table 7.1 . Two vasoactive systems, the renin-angiotensin system and the prostaglandins (PGs) ( Fig. 7.3 ), play key roles in maintaining GFR in the maturing kidney.
Vasoconstrictors | Vasodilators |
---|---|
Circulating hormones
| Circulating hormones
|
Paracrine + autocoïds
| Paracrine + autocoïds
|

Angiotensin II
Angiotensin II (ATII) is a very potent vasoconstrictor of the afferent and efferent arterioles, acting on two types of receptors, the AT 1 and the AT 2 receptor subtypes. The AT 1 receptors are widely distributed and appear to mediate most of the biologic effects of ATII. Although AT 2 receptors appear essential for the ureteric branching and cell proliferation, their exact role remains uncertain. ATII acts on both preglomerular and postglomerular resistance but appears to predominantly vasoconstrict the efferent arteriole, thereby increasing the glomerular capillary hydrostatic pressure while decreasing the glomerular plasma flow rate. This mechanism serves to maintain GFR when the renal perfusion pressure decreases to low levels. The action of ATII on the efferent arteriole is counterbalanced by intrarenal adenosine, an agent modulating the tubuloglomerular feedback mechanism. When both ATII and adenosine are overstimulated, their combined action results in afferent vasoconstriction.
Prostaglandins
The renal PGs are potent vasoactive metabolites of arachidonic acid. Under normal conditions, the renal PGs are present in low concentrations and exert only minor effects on the renal circulation and GFR. However, the vasodilator PGs are of major importance in protecting renal perfusion and GFR when vasoconstrictor forces are activated as, for instance, during hypotensive, hypovolemic, and sodium depletion states or during congestive heart failure. The PGs protect GFR by vasodilating the afferent arterioles and by dampening the renal vasoconstrictor effects of ATII, endothelin, and sympathetic nerve stimulation on the afferent arteriole.
Maturational Aspects of the Renin-Angiotensin and Prostaglandins Systems
Renin-Angiotensin System
Renin is found as early as the fifth week in the mesonephros and by the eighth week in the metanephros. The plasma renin concentration and activity generated by the fetal kidney are elevated. The presence of an intact functional renin-angiotensin system is a key factor for the maintenance of blood pressure and RBF during fetal life. Acting through its AT 1 receptors, ATII is indeed the promoter of the postnatal expression of postglomerular capillaries and organization of vasa recta bundles, which are necessary for development of normal RBF. Mutations in the genes encoding any of the components of the renin-angiotensin system result in severe tubular dysgenesis. The plasma renin activity further increases after birth before slowly decreasing through infancy. The factors controlling renin release (macula densa, baroreceptor, sympathetic nervous system, and hormonal mechanisms) are active in the fetus.
Elevated levels of ATII are present in the fetus and remain high in the neonatal period. Although AT 2 receptors predominate during embryonic and fetal life, they rapidly decline after birth. AT 1 receptors predominate after birth and are found in glomeruli, macula densa and mesangial cells, resistance arteries, and vasa recta. In addition to its AT 1 -mediated vasoconstrictor effects, ATII promotes growth of the renal vasculature. Blockade of the AT 1 receptors with losartan during fetal life interferes with normal renal development and results in vascular malformations, cystic dilatation of the tubules, and a decrease in the number of glomeruli. Blockade of AT 1 receptor by losartan in newborn rabbits induces a decrease in GFR without affecting RBF, thus illustrating the major role of ATII in regulating and protecting GFR in kidneys perfused at low systemic arterial pressures.
Prostaglandins
Cyclooxygenase 1 (COX-1) and 2 (COX-2) are expressed in the fetal renal vasculature, glomeruli, and collecting ducts. The COX-2 activity is highest after birth. Renal cortical COX-2–derived prostanoids, particularly PGI 2 and PGE 2 , play critical roles in maintaining blood pressure and renal function in volume-contracted states. Interference with PG synthesis during fetal life leads to renal dysgenesis with cortical dysplasia, cystic tubular dilatation, and impaired nephrogenesis. COX-2 is probably involved in this pathogenesis. Although the PGs probably do not regulate GFR in normal conditions, interference with PG synthesis may have deleterious effects in conditions associated with renal hypoperfusion.
Assessment of Glomerular Filtration Rate
Different methods using various markers have been used to assess GFR in neonates. The most common measurement of GFR is based on the concept of “clearance,” which relates the quantitative urinary excretion of a substance per unit of time to the volume of plasma that, if “cleared” completely of the same contained substance, would yield a quantity equivalent to that excreted in the urine. The clearance C of a substance x is expressed by the formula:
C = U X • V / P X
Inulin | Creatinine | Iohexol | DTPA | EDTA | Iothalamate | |
---|---|---|---|---|---|---|
Molecular weight (Da) | 5200 | 113 | 811 | 393 | 292 | 637 |
Elimination half-life (min) | 70 | 200 | 90 | 110 | 120 | 120 |
Plasma protein binding (%) | 0 | 0 | <2 | 5 | 0 | <5 |
Space of distribution | EC | TBW | EC | EC | EC | EC |
Endogenous Markers
Creatinine
Creatinine, the normal metabolite of creatine phosphate present in skeletal muscle, has a molecular weight (MW) of 113 Da. The renal excretion of endogenous creatinine is fairly similar to that of inulin in humans and several animal species. However, in addition to being filtered through the glomerulus, creatinine is secreted in part by the renal tubular cells.
Overestimation of GFR by creatinine clearance (C creat ) is usually more evident at low GFRs. As GFR decreases progressively during the course of renal disease, the renal tubular secretion of creatinine contributes an increasing fraction to urinary excretion, so that C creat may substantially exceed the actual GFR. Secretion of creatinine into the gut plays a role in this phenomenon.
Although creatinine has been used for decades, the methods available for its chemical determination are still biased by various interfering substances. The coloric method described by Jaffe is still widely used in clinical laboratories. Its major drawback is the interference by noncreatinine chromogens such as bilirubin, pyruvate, uric acid, cotrimoxazole, and cyclosporins. The use of enzymatic methods has increased the specificity of creatinine determination. Further improvement in measuring creatinine has been achieved by the use of newer techniques, such as high-performance liquid chromatography (HPLC), gas chromatography isotope dilution mass spectrometry (Gc-IDMS), and the HPLC-IDMS coupled technique. Gc-IDMS is now considered the method of choice for measuring true creatinine. It has an excellent specificity and low relative standard deviation (SD) (<0.3%). A method coupling HPLC with IDMS for the direct determination of creatinine has also been developed. The procedure is simple and speedy. It appears to offer the same advantage as the Gc-IDMS technique. Although accurate and reproducible assessment of creatinine is mandatory, the calibration of its measurement is not yet standardized to a gold standard in most places, leading to substantial variations among laboratories.
Creatinine as a Marker of Glomerular Filtration Rate in the Neonate
The handling of creatinine by immature kidneys is unique, with creatinine apparently undergoing glomerular filtration and partial tubular reabsorption. In newborn rabbits the urinary clearance of creatinine underestimates the concomitantly measured clearance of inulin with creatinine-to-inulin ratios less than 1 in the first days of life ( Fig. 7.4 ). Such ratios indicate that creatinine is actually reabsorbed at this stage of renal development. As the animals mature, the ratios increase to greater than 1, reflecting filtration and secretion of creatinine. Reabsorption of creatinine is present only in the first postnatal days and is probably explained by the passive reabsorption of the filtered creatinine across immature leaky tubules. When water is reabsorbed along the nephron, the concentration of filtered creatinine rises so that creatinine back-diffuses into the blood according to its concentration gradient, thus raising its plasma concentration. The clearance of creatinine has also been shown to underestimate true GFR in very low birth weight (VLBW) human neonates, thus suggesting the occurrence of the same phenomenon in human immature kidneys.

The use of creatinine as a marker of GFR in neonates is not hampered only by its specific handling by the immature kidney but also, as previously mentioned, by the interference of noncreatinine chromogens, sometimes leading to spurious overestimation of the true P creat .
Plasma Creatinine Concentration
The P creat concentration of the neonate is elevated at birth, reflecting the maternal P creat concentration. A near perfect equilibrium between the maternal and fetal P creat concentrations has indeed been shown to occur throughout gestation ( Fig. 7.5 ). In preterm infants the elevated P creat further increases transiently to reach a peak value between the second and fourth days of postnatal life ( Fig. 7.6 ). Peak P creat concentrations as high as 195 to 247 µmol/L in 23 to 26 weeks’ GA neonates and 99 to 140 µmol/L in 33 to 40 weeks’ GA neonates have been recorded ( Table 7.3 ). This transient increase in the VLBW neonates’ postnatal P creat concentration is probably the result of creatinine reabsorption across leaky tubules. This phenomenon accounts for the observation that when measured during the first week of life, the P creat is highest in the most premature infants. Reference ranges for P creat have been reported retrospectively in 218 appropriate-for-GA VLBW infants without risk factors for renal impairment or established from a cohort of 161 extremely premature infants followed during the first 8 weeks of life ( Fig. 7.7 ). Normal values for P creat (as measured by an enzymatic assay during the first year of life) are also available. Factors affecting P creat at birth and during the first postnatal weeks have been defined recently. Hypertensive disease of pregnancy, ibuprofen-treated patent ductus arteriosus (PDA), low Apgar scores, ventilation, and low GA were prominent factors influencing P creat at birth and later. In infants with hemodynamically significant PDA, P creat levels before ibuprofen were significantly higher than those recorded in GA-matched control participants without PDA.


Group Gestation Age (Weeks) | Birth Creatinine (µmol/L) a | Peak Plasma Creatinine (µmol/L) a | Time to Peak Plasma Creatinine (h) a |
---|---|---|---|
23–26 | 67–92 | 195–247 | 40–78 |
27–29 | 65–89 | 158–200 | 28–51 |
30–32 | 60–69 | 120–158 | 25–40 |
33–45 | 67–79 | 99–140 | 8–23 |

Estimation of Creatinine Clearance by the Length/Plasma Creatinine Ratio
The concentration of endogenous markers, such as creatinine, increases when GFR decreases. However, the increase in P creat is not linear. Several attempts have thus been made to develop reliable methods that will allow a correct estimate of C creat from its plasma concentration alone without urine collection. A formula has been developed for children, which allows an estimate of C creat (eC creat ) derived from the patient’s creatinine plasma concentration and body length:
eC creat = k • Length / P creat
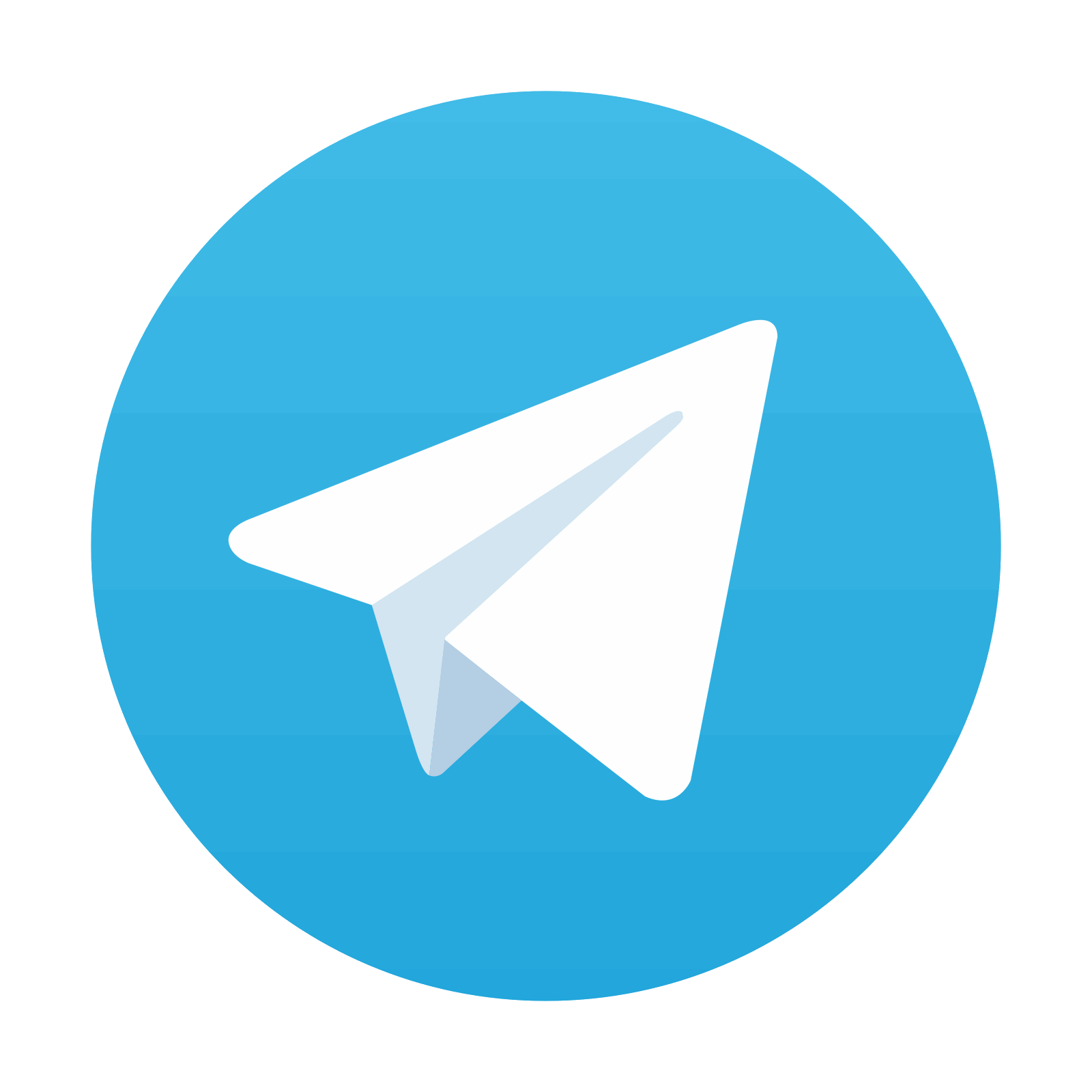
Stay updated, free articles. Join our Telegram channel

Full access? Get Clinical Tree
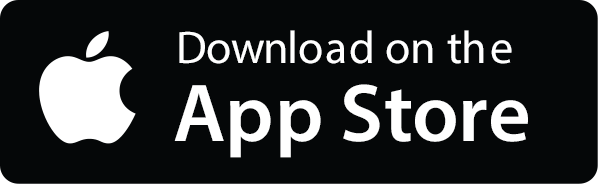
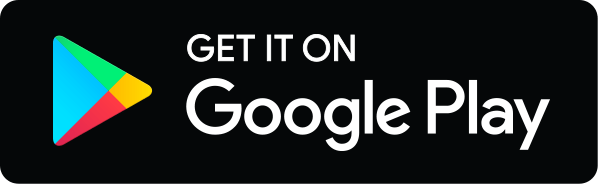