Abstract
Acute kidney injury (AKI) is very common in the neonatal period occurring in approximately 6% to 24% of newborns in the ICU. Emerging data suggest that AKI lends itself to a higher mortality independent of all other risk factors. AKI can have multiple etiologies and is classified into prerenal, intrinsic, and postrenal injury. Premature and small-for-gestational-age infants are at particular risk for the development of AKI and are also at risk for the development of hypertension, proteinuria, and chronic kidney disease (CKD) in the long term due to lower nephron mass. Renal replacement therapy in the form of peritoneal dialysis, hemodialysis, or continuous venovenous hemodialysis may be indicated for the use of endogenous and exogenous toxin removal and to maintain fluid, electrolyte, and acid-base balance.
Keywords
acute kidney injury, neonates, chronic kidney disease, biomarkers for acute kidney injury, etiology of acute kidney injury, peritoneal dialysis, hemodialysis, continuous venovenous hemodialysis
Introduction
Acute kidney injury (AKI; previously known as acute renal failure) and chronic kidney disease (CKD; previously known as chronic renal failure) in neonates is a very common problem, and there are many different causes of kidney injury in newborns ( Table 18.1 ). Although many cases of AKI can resolve with return of kidney function to normal, some causes of AKI result in permanent kidney injury that is apparent immediately. Others may result in kidney disease years after the initial insult. It is well known that kidney diseases such as kidney dysplasia, congenital abnormalities of the kidney and genitourinary tract, and cortical necrosis can lead to CKD. In contrast, it has been thought in the past that AKI caused by hypoxic ischemic and nephrotoxic insults was reversible with return of kidney function to normal. However, recent studies have demonstrated that hypoxic and ischemic insults can result in physiologic and morphologic alterations in the kidney that can lead to kidney disease at a later time. Thus, as will be discussed in more detail later, AKI in neonates from any cause is a risk factor for later development of CKD.
Prerenal Injury |
|
Intrinsic Kidney Disease |
|
Obstructive Uropathy |
|
Congenital Kidney Diseases |
|
In addition it is becoming well recognized that small-for-gestational-age and premature infants both have a lower nephron mass at birth, which in turn predisposes them to the development of CKD over time. In a study evaluating six young adults for proteinuria, renal biopsy revealed focal segmental glomerulosclerosis (FSGS). These patients were all premature infants born between 22 and 30 weeks of gestation with a mean birth weight of 1054 g and did not have any other risk factors for renal disease. In another study analyzing 5352 children aged 12 to 15, it was found that those born with very low or low birth weight had a higher risk of developing hypertension and a lower glomerular filtration rate (GFR) at later points in time.
AKI is classified into prerenal, intrinsic, and postrenal injury. In addition, the insults may be acquired in the prenatal, perinatal, and/or postnatal period. In newborns, kidney injury may have a prenatal onset in congenital diseases such as kidney dysplasia with or without obstructive uropathy and in genetic diseases such as autosomal recessive polycystic kidney disease. Newborns with congenital and genetic kidney diseases that have a prenatal onset may have stigmata of Potter syndrome caused by in utero oliguria with resultant oligohydramnios. Newborns with Potter syndrome may have life-threatening pulmonary insufficiency, a flattened nasal bridge, low-set ears, joint contractures, and other orthopedic anomalies caused by fetal constraint as a result of the oligohydramnios.
AKI and CKD may also result from the in utero exposure of the developing kidneys to agents that may interfere with nephrogenesis such as angiotensin-converting enzyme (ACE) inhibitors, angiotensin II receptor blockers (ARBs), and perhaps cyclooxygenase (COX) inhibitors. Exposure of a developing fetus to ACE inhibitors and ARBs has been associated with kidney dysfunction that is both acute and chronic, undermineralization of the calvarial bones, and in some cases fetal demise. Exposure to ACE inhibitors or ARBs is detrimental for kidney development at any point during the pregnancy; however the most damaging effects are noted with exposure during the second and third trimesters ; however AKI in newborns is also commonly acquired in the postnatal period because of hypoxic ischemic injury and toxic insults. As in older children, hospital-acquired AKI in newborns is frequently multifactorial in origin. Given that nephrogenesis proceeds through approximately 34 weeks’ gestation, ischemic or hypoxic and toxic insults in the developing kidney in a premature newborn can result not only in AKI, but also in long-term complications secondary to interrupted nephrogenesis. Whether kidney disease is congenital or acquired, it is important to appropriately manage the fluid and electrolyte imbalances and other side effects of kidney injury in newborns.
Definition
Currently, there is no uniform definition of AKI in pediatric or neonatal patients, and AKI is defined in multiple ways, but the majority of definitions of AKI in use involve a change in the serum creatinine level. In neonates, AKI is frequently defined by a change in the serum creatinine according to gestational age. AKI is characterized by an increase in the blood concentration of creatinine and nitrogenous waste products, a decrease in the GFR, and the inability of the kidney to appropriately regulate fluid and electrolyte homeostasis. However, creatinine is an inaccurate marker of kidney function in the neonate for reasons discussed below.
The definition of AKI in pediatric patients has been quite variable. A new classification system called the RIFLE criteria ( r isk for renal dysfunction, i njury to the kidney, f ailure of kidney function, l oss of kidney function, and e nd-stage kidney disease) has been proposed as a standardized classification of AKI in adults and has been adapted for pediatric patients. The pediatric RIFLE (pRIFLE) was found to better classify pediatric AKI and to reflect the course of AKI in children admitted to intensive care units (ICUs). In a study of pediatric patients of whom 36% were neonates, AKI as classified by pRIFLE criteria was shown to correlate with mechanical ventilation, metabolic acidosis, and hypoxia in the neonate. In the pRIFLE criteria, R isk is classified as a reduction in estimated creatinine clearance (eCCl) by 25% or a drop in urine output to less than 0.5 mL/kg per hour for 8 hours. I njury is classified as a drop in eCCl by 50% with urine output reduced by less than 0.5 mL/kg per hour for 16 hours. F ailure is when eCCl is reduced by 75% or eCCl is less than 35 mL/min per 1.73 m 2 with urine output being less than 0.3 mL/kg per hour for 24 hours or anuria for 12 hours. L oss is defined by the failure stage lasting for longer than 4 weeks. E nd-stage renal disease is when there is persistent failure for longer than 3 months.
Although a decrease in urine output is a common clinical manifestation of AKI, many forms of AKI are associated with normal urine output. Whereas newborns with prerenal injury, AKI caused by hypoxic/ischemic insults, or cortical necrosis are more likely to have oligo/anuria (urine output <1.0 mL/kg per hour), newborns with nephrotoxic kidney insults, including aminoglycoside nephrotoxicity and contrast nephropathy, are more likely to have AKI with normal urine output. The morbidity and mortality of nonoliguric AKI are substantially less than oliguric AKI.
Nephron formation in the fetus begins at 5 weeks of gestation with urine production starting at around 10 weeks of gestation. The fetal urine contributes to the development of amniotic fluid, and the production of urine increases with gestational age. Nephrogenesis is complete by approximately 34 to 36 weeks of gestation. Therefore, preterm infants born before 34 weeks of gestation have a lower GFR. GFR doubles in the first 2 weeks of life for the term and preterm infant secondary to increased renal blood flow, a higher mean arterial pressure and increased glomerular surface area, and permeability resulting in a subsequent drop in creatinine by about 50%.
Immediately after birth, the serum creatinine in the newborn is a reflection of maternal kidney function due to placental transfer of creatinine and cannot be used as a measure of kidney function. In full-term healthy newborns, the GFR rapidly increases and the serum creatinine declines to about 0.4 to 0.6 mg/dL at about 2 weeks of age, while the serum creatinine declines at a slower rate in premature infants. In very-low-birth-weight (VLBW) infants with a gestational age around 28 weeks, the renal function improves even more slowly compared to infants born at a gestational age around 32 weeks. It is important to note that creatinine clearance calculated using urine collections in preterm infants typically underestimates the GFR because of low creatinine excretion and tubular reabsorption of creatinine by immature renal tubules. Therefore, caution must be used when using creatinine alone as a marker for kidney injury in preterm infants due to a risk of possible over diagnosis of AKI. It is also important to note that the use of serum creatinine as a determinate of kidney insufficiency requires that the gestational age at time of birth and the postnatal age, as well as maternal factors, are taken into account.
Additional disadvantages of using serum creatinine are that it lags behind changes in GFR and varies with muscle mass, hydration status, and gender. It is clear that a change in the serum creatinine is a rough measure of changes in kidney function and better determinates of real-time kidney renal function are needed. The development, testing, and successful implementation of therapeutic strategies in AKI is going to require the development of sensitive biomarkers so that therapy can be initiated in a timely manner.
This chapter reviews biomarkers of AKI, the epidemiology of kidney injury in newborns, the common causes of AKI and CKD in newborns with a focus on hypoxic ischemic and nephrotoxic insults, management of AKI and CKD in newborns, and long-term follow-up of neonates who have had AKI.
Biomarkers for Acute Kidney Injury
Recent studies have investigated the use of early biomarkers of AKI so that AKI can be recognized before changes in the serum creatinine occur. Blood serum and urine biomarkers have been identified and are currently being studied. Some of the biomarkers under investigation include plasma and urine neutrophil gelatinase-associated lipocalin (NGAL) and cystatin C (CysC), interleukin-18 (IL-18), kidney injury molecule-1 (KIM-1), urine osteopontin, urinary epidermal growth factor (EGF), and uromodulin. NGAL has shown promise in children undergoing cardiac surgery as an early maker of AKI. Studies in premature and term infants note that urine NGAL is detectable and correlates with birth weight and gestational age; however, levels were increased in neonates with normal renal function.
Serum CysC is emerging as a promising marker of GFR. CysC is a low-molecular-weight protein that is produced in all human cells with a nucleus, remains independent of muscle mass, is filtered by the glomerulus, and is completely degraded in the tubular walls. CysC production is fairly constant from 1 to 50 years of age. Abitbol et al. found that CysC was a better biomarker than serum Cr in the assessment of GFR in premature infants. In their study, they note that for term infants using a GFR calculation utilizing the combination of CysC and Cr equation by Zappitelli et al. provided a better estimation of GFR compared to Cr or CysC alone.
McCaffrey et al. confirmed the correlation of serum CysC and serum NGAL with AKI in children admitted to the pediatric ICU (PICU) without sepsis. Hanna et al. evaluated early urinary biomarkers of AKI in preterm infants less than 32 weeks of gestation and found that infants who went on to develop AKI had higher urinary NGAL, CysC, and osteopontin and a lower urinary EGF and uromodulin. They also noted the urinary biomarkers changed 24 hours prior to the change in serum creatinine. Askenazi et al. also performed a prospective study on 113 VLBW premature infants and had similar findings. In their study, infants with AKI had higher levels of urine NGAL, CysC, osteopontin, clusterin, and alpha glutathione S-transferase and lower urine levels of EGF and uromodulin.
There is also the emerging field of metabolomics in pediatric nephrology which refers to the evaluation of metabolites and their changes in a system due to genetic or environmental stimuli. Metabolites and their patterns can serve as a signature of real-time physiologic and pathophysiologic states in the body, and hence both pattern recognition and metabolite identification can allow them to be used as biomarkers. Metabolomics certainly appears to hold promise for early disease recognition and possibly targeted intervention and treatment in the future.
Epidemiology and Incidence of Kidney Injury in Neonates
AKI in neonates is fairly common and is a major contributor to morbidity and mortality. The precise incidence and prevalence of kidney disease in the newborn are unknown in part due to the lack of a standard definition of AKI and variation in practice patterns when screening for AKI. Studies also vary greatly when assessing AKI based on cut offs for serum creatinine and urine output, making it challenging to compare multiple studies.
Although the precise incidence and prevalence of kidney disease in newborns is unknown, several studies have demonstrated that kidney injury is common in neonatal ICUs (NICUs) and PICUs. The incidence of kidney disease ranged from 6% to 24% of newborns in NICUs and is particularly common in neonates who have undergone cardiac surgery. In a prospective study by Askenazi et al., the incidence of AKI in very low birth weight infants within the first 2 weeks of life was found to be 25%.
Neonates with severe asphyxia had a high incidence of AKI; AKI is less common in neonates with moderate asphyxia, and in one study the AKI was nonoliguric, oliguric, and anuric in 60%, 25%, and 15%, respectively. Other studies have demonstrated that a very low birth weight (VLBW; <1500 g), low Apgar score, patent ductus arteriosus (PDA), and maternal administration of antibiotics and nonsteroidal antiinflammatory drugs (NSAIDs) was associated with the development of AKI. Additional studies have also shown that low Apgar score and maternal ingestion of NSAIDs is associated with decreased kidney function in preterm infants. A recent study demonstrated that AKI was diagnosed in 56% of infants with perinatal asphyxia.
Several very interesting studies have demonstrated that some newborns may have genetic risk factors for AKI. Polymorphism of the ACE gene or the angiotensin receptor gene with resultant alterations in activity of the renin-angiotensin system might play a role in the development of AKI. In studies in newborns, polymorphisms of tumor necrosis factor α (TNF-α), IL-1b, IL-6, and IL-10 genes were investigated in newborns to determine if polymorphisms of these genes would lead to a more intense inflammatory response and predispose newborns to AKI. The allelic frequency of the individual genes did not differ between newborns with AKI and those without AKI, but the carrier state of being a high TNF-α producer along with a low IL-6 producer was present in 26% of newborns who developed AKI compared with 6% of newborns who did not. The investigators suggested that the combination of these polymorphisms might lead to a greater inflammatory response and the development of AKI in neonates with infection. As described below, future therapies for AKI might involve strategies to interrupt the inflammatory response. In other studies, the incidence of ACE I/D allele genotypes or the variants of the angiotensin I receptor gene did not differ in neonates with AKI compared with neonates without AKI, but they may be associated with PDA and heart failure and indirectly contribute to CKD. AKI occurred more commonly in VLBW neonates carrying the heat shock protein 72 (1267) GG genetic variation, which is associated with low inducibility of heat shock protein 72. Given the important role of heat shock proteins in ischemic kidney injury, these findings suggest that some neonates are more susceptible to ischemic injury. Future studies of the genetic background of children at risk for AKI because of medication exposure, toxin exposure, ischemic hypoxic insults, or other insults will likely impact the management of children at risk for AKI and the management of AKI.
To better understand AKI in neonates the National Kidney Collaborative has recently been formed. The Neonatal Kidney Collaborative is a group comprised of neonatologists and nephrologists who have initiated the Assessment of Worldwide Acute Kidney injury Epidemiology in Neonates (AWAKEN) Study. This will be the largest AKI study in neonates to date and will help determine if the Kidney Disease: Improving Global Outcomes (KDIGO) AKI definition in neonates can be used as an appropriate classification tool that could be associated/correlated with length of stay, outcomes, and mortality. The study will also assess the applicability of different definitions of AKI in the neonates. Another goal of the study is to determine how the fluid balance in the neonate relates to outcomes.
Etiology of Kidney Injury in Newborns
There are many different etiologies of AKI and CKD in the neonate (see Table 18.1 ), and the most common are related to prerenal mechanisms, including hypotension, hypovolemia, hypoxemia, perinatal and postnatal asphyxia, sepsis, and congenital anomalies of the kidney and urinary tract (CAKUT).
Prerenal Injury
In prerenal injury, kidney function is decreased because of decreased kidney perfusion, but the kidney is intrinsically normal. Restoration of normal kidney perfusion results in a return of kidney function to normal; acute tubular necrosis (ATN) implies that the kidney has experienced intrinsic damage. However, the evolution of prerenal injury to intrinsic kidney injury is not sudden, and a number of compensatory mechanisms work together to maintain kidney perfusion when kidney perfusion is compromised. When kidney perfusion is decreased, the afferent arteriole relaxes its vascular tone to decrease kidney vascular resistance and maintain kidney blood flow. Decreased kidney perfusion results in increased catecholamine secretion, activation of the renin-angiotensin system, and generation of prostaglandins. During kidney hypoperfusion, the intrarenal generation of vasodilatory prostaglandins, including prostacyclin, mediates vasodilatation of the kidney microvasculature to maintain kidney perfusion. Administration of aspirin or NSAIDs can inhibit this compensatory mechanism and precipitate acute kidney insufficiency during kidney hypoperfusion. As discussed later, administration of indomethacin for closure of the PDA in premature newborns is associated with a substantial risk of kidney insufficiency. It was originally thought that selective COX-2 inhibitors would be kidney-sparing, but it has been recognized that the selective COX-2 inhibitors can adversely affect kidney hemodynamics similar to the effects of nonselective COX inhibitors. In addition, clinical use of selective COX-2 inhibitors has been associated with AKI in adult patients. Similarly, when kidney perfusion pressure is low as in renal artery stenosis, the intraglomerular pressure necessary to drive filtration is partly mediated by increased intrarenal generation of angiotensin II to increase efferent arteriolar resistance. Administration of ACE inhibitors in these conditions can eliminate the pressure gradient needed to drive filtration and precipitate AKI. Thus, administration of medications that can interfere with compensatory mechanisms to maintain kidney perfusion can precipitate AKI in certain clinical circumstances.
Prerenal injury results from kidney hypoperfusion caused by true volume contraction or from a decreased effective blood volume. Volume contraction results from hemorrhage, dehydration caused by gastrointestinal losses, salt-wasting kidney or adrenal diseases, central or nephrogenic diabetes insipidus, increased insensible losses, and in disease states associated with third-spaces losses such as sepsis, traumatized tissue, and capillary leak syndrome; decreased effective blood volume occurs when the true blood volume is normal or increased, but kidney perfusion is decreased because of diseases such as congestive heart failure and cardiac tamponade. Whether prerenal injury is caused by true volume depletion or decreased effective blood volume, correction of the underlying disturbance will return kidney function to normal.
The urine osmolality, urine sodium concentration, the fractional excretion of sodium (FENa), and renal failure index have all been proposed to be used to help differentiate prerenal injury from vasomotor nephropathy or ATN. This differentiation is based on the premise that the tubules are working appropriately in prerenal injury and are, therefore, able to conserve salt and water appropriately. In vasomotor nephropathy, the tubules have progressed to irreversible injury and are unable to appropriately conserve sodium. During prerenal injury, the tubules are able to respond to decreased kidney perfusion by appropriately conserving sodium and water such that the urine osmolality is greater than 400 to 500 mOsm/kg H 2 O, the urine sodium is less than 10 to 20 mEq/L, and the FENa is less than 1% in children. It has been noted, however, that there is an inverse correlation of FENa with gestational age. Gallini et al. studied 83 premature infants who were grouped into four categories based on gestational age of less than 27 weeks, 27 to 28 weeks, 29 to 30 weeks, and 31 to 32 weeks and observed that the lower the gestational age the higher the urinary sodium. Due to this phenomenon, it is recommended that for newborns and premature infants the corresponding values suggestive of kidney hypoperfusion are urine osmolality greater than 350 mOsm/kg H 2 O, urine sodium less than 20 to 30 mEq/L, and FENa of less than 2.5%. However, the use of these numbers to differentiate prerenal injury from ATN requires the patient to have normal tubular function initially. Although this may be the case in some pediatric patients, newborns, particularly premature newborns whose tubules are immature, may have kidney injury with urinary indices showing a higher urine sodium. The interpretation of the elevated urine sodium is challenging because based on the study by Gallini et al., this would be part of normal physiology. Therefore, it is important to consider the state of the function of the tubules before the potential onset that might precipitate vasomotor nephropathy or ATN.
Acute Ischemic Kidney Injury
Ischemic AKI (also known as ATN) can evolve from prerenal injury if the insult is severe and sufficient enough to result in vasoconstriction and ATN. Recent studies suggest that the vasculature of the kidney may play a role in acute injury and chronic injury as well, and the endothelial cell has been identified as a target of injury. Peritubular capillary blood flow has been shown to be abnormal during reperfusion, and there is also loss of normal endothelial cell function in association with distorted peritubular pericapillary morphology and function. The mechanism of cellular injury in hypoxic/ischemic AKI is not known, but alterations in endothelin (ET) or nitric oxide (NO) regulation of vascular tone, adenosine triphosphate (ATP) depletion and alterations in the cytoskeleton, changes in heat shock proteins, initiation of the inflammatory response, and the generation of reactive oxygen and nitrogen molecules may each play a role in cell injury.
NO is a vasodilator produced from endothelial NO synthase (eNOS) and NO helps regulate vascular tone and blood flow in the kidneys. Recent studies suggest that loss of normal eNOS function occurs after ischemic/hypoxic injury, which could precipitate vasoconstriction. In contrast, inducible NO synthase (iNOS) activity increases after hypoxic/ischemic injury, and iNOS can participate in the generation of reactive oxygen and nitrogen molecules. Inducible NO synthase with the generation of toxic NO metabolites, including peroxynitrate, has been shown to mediate tubular injury in animal models of AKI. ET peptides are potent vasoconstrictors that have also been shown to play a role in the pathogenesis of AKI in animal models. In animal models of AKI in rats, circulating levels of ET-1 and tissue expression of ET-1 protein levels was substantially increased, and ET(A) and ET(B) receptor gene expression was also increased after ischemic injury. ET receptor agonist for the A receptor has been shown to decrease AKI in animal models. Thus, alterations in the balance of vasoconstrictive and vasostimulatory stimuli are likely to be involved in the pathogenesis of hypoxic/ischemic AKI.
An initial response to hypoxic/ischemic AKI is ATP depletion, which leads to a number of detrimental biochemical and physiologic responses, including disruption of the normal cytoskeletal organization with loss of the apical brush border and loss of polarity with Na + , K + -ATPase localized to the apical as well as the basolateral membrane. This has been shown in several animal models of AKI and it has also been shown in human kidney allografts that loss of polarity with mislocation of Na + , K + -ATPase to apical membrane contributes to kidney dysfunction in transplanted kidneys. Reactive oxygen molecules are also generated during reperfusion and can contribute to tissue injury. Although tubular cells and endothelial cells are susceptible to injury by reactive oxygen molecules, studies have shown that endothelial cells are more sensitive to oxidant injury than tubular epithelial cells. Other studies have shown an important role for heat shock protein in modifying the kidney response to ischemic injury, as well as playing a role in promoting recovery of the cytoskeleton after AKI.
In children and neonates with multiorgan failure, the systemic inflammatory response is thought to contribute to AKI as well as other organ dysfunction by the activation of the inflammatory response, including increased production of cytokines and reactive oxygen molecules, activation of polymorphonuclear leukocytes (PMNs), and increased expression of leukocyte adhesion molecules. Reactive oxygen molecules can be generated by several mechanisms, including activated PMNs, which may cause injury by the generation of reactive oxygen molecules, including superoxide anion, hydrogen peroxide, hydroxyl radical, hypochlorous acid, peroxynitrite, or by the release of proteolytic enzymes. Myeloperoxidase from activated PMNs converts hydrogen peroxide to hypochloruous acid, which may react with amine groups to form chloramines; each of these can oxidize proteins, DNA, and lipids, resulting in substantial tissue injury. Leukocyte endothelial cell adhesion molecules have been shown to be unregulated in ATN, and administration of antiadhesion molecules can substantially decrease kidney injury in animal models of ATN. As described later, several animal models have shown future therapies for hypoxic/ischemic AKI may involve manipulation of the inflammatory response. Studies in humans with AKI have demonstrated an increased evidence of oxidation of proteins, reflecting oxidant stress.
In established ATN, the urinalysis may be unremarkable or demonstrate low-grade proteinuria and granular casts, and urine indices of tubular function demonstrate an inability to conserve sodium and water as described above. The creatinine typically increases by about 0.5 to 1.0 mg/dL (44.2–88.4 µmol/L) per day. Radiographic studies demonstrate kidneys of normal size with loss of corticomedullary differentiation, but a radionucleotide kidney scan with technetium–99-MAG3 (mercapto acetyl triglycine) or technetium–99-DTPA (diethylene-triamine-penta-acetic acid) will demonstrate normal or slightly decreased kidney blood flow with poor function and delayed accumulation of the radioisotope in the kidney parenchyma without excretion of the isotope in the collecting system ( Fig. 18.1B ).

In the past, it has been thought that the prognosis of ischemic AKI was good except in cases in which the insult was of sufficient severity to lead to vasculature injury and microthrombi formation with the subsequent development of cortical necrosis. However, recent studies demonstrate that chronic changes can occur and that such patients are at risk for later complications. As discussed later, AKI before nephrogenesis is complete may also result in disrupted nephrogenesis and reduced nephron number.
Hypothermia protocols are now frequently being used in neonates with severe hypoxic-ischemic events and have demonstrated improved neurologic outcomes. Do these protocols improve renal outcomes as well? Selewski et al. retrospectively evaluated 96 neonates who had undergone the hypothermia protocol and noted that 36 out of 96 (38%) infants had AKI. The mortality was noted to be 14% in neonates with AKI vs. 3% in neonates without AKI. In their study, the rate of AKI was the same in infants who received whole body cooling compared to infants who only underwent selective head cooling. Confirming previous studies, they noted that patients with AKI had longer days in the NICU as well as more days on mechanical ventilation. Interestingly, their study reflected a slightly lower rate of AKI compared to infants without the hypothermia protocol; however, given the small sample size, it is difficult to extrapolate these results to a larger cohort. Additional studies in this area will shed more light on the potential nephro-protective effect of the hypothermia protocols.
The recovery of the neonate and the recovery of kidney function depend on the underlying events which precipitated the ischemic/hypoxic insults. Studies also indicate that AKI contributes directly to the morbidity and mortality of neonates and children in the NICUs and PICUs. In children who recover from ATN, the kidney function returns to normal, but the length of time before recovery is quite variable. Some children begin to recover kidney function within days of the onset of kidney injury, but recovery may not occur for several weeks in other children. Return of kidney function may be accompanied by a diuretic phase with excessive urine output at a time when the tubules have begun to recover from the insult, but have not recovered sufficiently to appropriately reabsorb solute and water. When the diuretic phase occurs during recovery, close attention to fluid and electrolyte balance is very important to ensure adequate fluid management to promote recovery from ATN and prevent additional kidney injury. As described below, long-term follow-up of newborns with AKI is warranted to evaluate for late complications.
Nephrotoxic Kidney Injury
Nephrotoxic AKI may result from the administration of a number of different medications as well as from indigenous compounds such as hemoglobinuria or myoglobinuria. Nephrotoxic AKI in newborns is commonly associated with aminoglycoside antibiotics, NSAIDs, intravascular contrast media, and amphotericin B; other medications have been implicated less commonly. It is important to note that AKI secondary to nephrotoxic medications is potentially avoidable and at minimum, the degree of AKI is modifiable. While a single nephrotoxic medication increases the risk of AKI, the use of multiple nephrotoxic medications increases the risk exponentially more. In addition, concomitant factors such as neonatal age, acuity of illness, and dosage and duration of medications all play a role in the degree of resulting AKI. In a recent study by Rhone et al. of nephrotoxic medication exposure in very low birth weight infants, the authors found that exposure to one or more nephrotoxic medications occurred in 87% of the infants. The most common exposures were gentamicin (86%), indomethacin (43%), and vancomycin (25%). They also noted that the greatest exposure occurred in the smallest and most immature infants as well as those suffering from AKI. Below we will discuss some of the main drugs causing AKI in the neonate.
Aminoglycosides: Aminoglycosides remain one of the most commonly prescribed antibiotics in the NICU given their efficacy against gram-negative bacteria. They are excreted in the urine and 5% to 10% of the drug accumulates in the renal cortex. High concentrations of aminoglycosides can damage the proximal tubules. Aminoglycoside nephrotoxicity usually presents with nonoliguric AKI with a urinalysis showing minimal urinary abnormalities. The incidence of aminoglycoside antibiotic nephrotoxicity is related to the dose and duration of the antibiotic therapy, as well as the level of kidney function before the initiation of aminoglycoside therapy. The etiology of aminoglycoside nephrotoxicity is thought to be related to the lysosomal dysfunction of proximal tubules and is reversible once the aminoglycoside antibiotics have been discontinued. However, after the aminoglycoside is discontinued, the serum creatinine may continue to increase for several days because of ongoing tubular injury from continued high parenchymal levels of the aminoglycoside.
Angiotensin Converting Enzyme Inhibitors: The renin-angiotensin-system (RAS) plays a significant role in renal development, and infants exposed prenatally to ACE inhibitors and angiotensin receptor blockers have been found to have profound renal function abnormalities. Interruption of the RAS can result in abnormal renal development, oligohydramnios, renal failure, and pulmonary hypoplasia. Exposure in the second and third trimesters has been found to cause more severe renal abnormalities compared to exposure in the first trimester. Outside of the genitourinary system, ACE inhibitors can also cause central nervous system abnormalities, sensorineural hearing loss, and cardiac abnormalities. Typically, prenatal exposure to ACE inhibitors and ARBs tends to happen in women with absent or late prenatal care. Given the harmful effects of these agents on kidney development, it may be prudent to limit the use of ACE inhibitors in neonates less than 34 weeks of gestation when nephron formation may potentially be ongoing. The use of ACE inhibitors in neonates can also result in AKI, which reassuringly is reversible with discontinuation of the medication. Careful monitoring of intravascular volume and avoidance of simultaneous nephrotoxic medications should be done whenever possible to lower the risk of AKI.
NSAIDs: NSAIDs may also precipitate AKI by their effect on intrarenal hemodynamics. Neonates have high levels of circulating prostaglandins which are involved in maintaining arteriolar vasodilation, renal blood flow, and renal water clearance. Inhibition of prostaglandins, which occurs with NSAIDs, results in a decrease in renal blood flow and reduction in GFR. Indomethacin therapy to promote closure of PDAs in premature neonates is associated with kidney dysfunction, including a 56% reduction in urinary flow rate, a 27% reduction in GFR, and a 66% reduction in free water clearance. Other physiologic alterations after administration of indomethacin and ibuprofen include a decrease in urinary ET-1 and arginine vasopressin, along with a reduction in urinary sodium excretion and FENa. Alterations in kidney function occur in approximately 40% of premature newborns who have received indomethacin, and such alterations are usually reversible. In a large study of more than 2500 premature newborns treated with indomethacin to promote closure of the PDA, infants with preexisting kidney and electrolyte abnormalities and infants whose mothers had received indomethacin tocolysis or who had chorioamnionitis were at significantly increased risk for the development of kidney impairment.
Vancomycin: Vancomycin has been implicated in nephrotoxicity in the neonates; however, some recent studies suggest that monotherapy with appropriate trough levels may not cause AKI. The risk factors for the development of AKI in the setting of vancomycin use appear to be high trough levels, additional nephrotoxin use (such as NSAIDs), positive blood cultures, PDA, low birth weight, and severity of illness. The exact mechanism of the injury remains unclear, but is likely related to oxidative injury and stress at the level of the proximal tubule based on animal models.
Vascular Injury
Renal artery thrombosis and renal vein thrombosis will result in AKI if bilateral or if either occurs in a solitary kidney. Renal artery thrombosis is strongly associated with an umbilical artery line and a PDA. In the setting of a renal arterial thrombosis, maternal anticardiolipin antibody and maternal lupus anticoagulant levels should be checked, and the neonate should be evaluated further to determine if prothrombotic disorders are present. In addition to AKI, children may demonstrate hypertension, gross or microscopic hematuria, thrombocytopenia, and oliguria. In renal artery thrombosis, the initial ultrasound may appear normal or demonstrate minor abnormalities, but a renal scan will demonstrate little to no blood flow. The risk factors for renal vein thrombosis include birth asphyxia, maternal diabetes, volume contraction, disorders of coagulation, decreased vascular blood flow, increased blood viscosity, and increased serum osmolality. In renal vein thrombosis, the ultrasound demonstrates an enlarged, swollen kidney, but the renal scan typically demonstrates decreased blood flow and function. Therapy should be aimed at limiting extension of the clot by removal of the umbilical arterial catheter, and anticoagulate or fibrinolytic therapy can be considered, particularly if the clot is large.
Cortical necrosis is associated with hypoxic/ischemic insults due to perinatal anoxia, placenta abruption, and twin-twin or twin-maternal transfusions with resultant activation of the coagulation cascade. Interestingly, intrauterine laser treatment in 18 sets of twins with twin-twin transfusion resulted in no long-term kidney impairment despite severe alterations of kidney function, including anuria and polyuria before the laser treatment. Newborns with cortical necrosis usually have gross or microscopic hematuria and oliguria and may have hypertension as well. In addition to laboratory features of an elevated blood urea nitrogen (BUN) and creatinine, thrombocytopenia may also be present due to the microvascular injury. Radiographic features include a normal kidney ultrasound scan in the early phase, while ultrasound scans in the later phases may show that the kidney has undergone atrophy and has substantially decreased in size. A radionucleotide renal scan will show decreased to no perfusion with delayed or no function (see Fig. 18.1B ), in contrast to delayed uptake of the radioisotope, which is observed in ATN (see Fig. 18.1A ). The prognosis of cortical necrosis is worse than that of ATN. Children with cortical necrosis may have partial recovery or no recovery at all. Typically, children with cortical necrosis will need short- or long-term dialysis therapy, but children who do recover sufficient renal function are at risk for the late development of CKD as described below.
Congenital Abnormalities of the Kidney and Genitourinary Tract
Obstruction of the urinary tract can cause AKI if the obstruction occurs in a solitary kidney, if it involves the ureters bilaterally, or if there is urethral obstruction. Obstruction can result from congenital malformations such as posterior urethral valves, bilateral ureteropelvic junction (UPJ) obstruction, or bilateral obstructive ureteroceles. Acquired urinary tract obstruction can result from passage of kidney stones or, rarely, tumors. It is important to evaluate for obstruction because the management is to promptly relieve the obstruction.
CAKUT represents the most common cause of CKD in the neonate. Based on the North American Pediatrics Renal Trials and Collaborative Studies report in 2014, the most common cause of underlying end stage renal disease in children receiving a kidney transplant was renal aplasia/hypoplasia/dysplasia accounting for 15.8% of the cases, followed closely by obstructive uropathy being the cause in 15.3% of the children. Renal dysplasia is believed to arise either from defects in the differentiation of the renal parenchyma or due to events secondary to an obstruction of the lower urinary tract as seen in posterior urethral valves, UPJ obstruction, bilateral ureteroceles, or vesicoureteric reflux. The complex array of underlying causes resulting in abnormal kidney formation is not entirely understood and most likely involves genetic, epigenetic, and environmental factors merging together to manifest in a certain phenotype. However, progress is currently being made to identify molecular signals and patterning pathways that contribute to renal dysplasia. Some of the genes that have so far been implicated in renal dysplasia are Pax2 (renal coloboma syndrome), Hnf1beta (renal cysts and diabetes syndrome), Sall1 (Townes-Brocks syndrome), Six2 (CAKUT), Wt1 (Wilms tumor and renal agenesis), Ret (CAKUT), Eya1 (brachio-oto-renal syndrome), miR17~92 (Fiengold syndrome), and Fgf9/Fgf20 (CAKUT). In addition, investigators have identified genetic alterations in more than 160 rare kidney diseases.
Medical Management of Acute Kidney Injury in Neonates
Preventive Measures
It is thought that prerenal injury is the most common cause of AKI in neonates in a resource-poor setting, and since dialytic resources were scarce, the mortality rate was high from prerenal failure. Thus, on a global scale, the prevention of AKI in the neonate with adequate hydration is likely to have a larger impact on mortality than other measures.
Intravenous (IV) infusion of theophylline in severely asphyxiated neonates given within the first hour after birth was associated with improved fluid balance, improved creatinine clearance, and reduced serum creatinine levels with no effects on neurologic and respiratory complications. Other studies in asphyxiated neonates also demonstrated improved kidney function and decreased excretion of β-2 microglobulin in the neonates given theophylline within 1 hour of birth. However, the clinical significance of the improved kidney function was not clear, and the incidence of persistent pulmonary hypertension was higher in the neonates who had received theophylline group. The beneficial effects of theophylline are likely to be mediated by its adenosine antagonistic properties. Additional studies are needed to determine the significance of these findings and the potential side effects of theophylline.
After intrinsic kidney injury has become established, management of the metabolic complications of AKI involves appropriate monitoring of fluid balance, electrolyte status, acid-base balance, nutrition, and the initiation of renal replacement therapy when appropriate. Diuretic therapy to stimulate urine output eases management of AKI, but the conversion of oliguric to nonoliguric AKI has not been shown to alter the course of AKI. Diuretic therapy has potential theoretical mechanisms to prevent, limit, or improve kidney function. Mannitol (0.5–1.0 g/kg over several minutes) may increase intratubular urine flow to limit tubular obstruction and may limit cell damage by prevention of swelling or by acting as a scavenger of free radicals or reactive oxygen molecules. Lasix (1–5 mg/kg per dose) also increases urine flow rate to decrease intratubular obstruction. When using mannitol in children or neonates with AKI, a lack of response to therapy can precipitate congestive heart failure, particularly if the child’s intravascular volume is expanded before mannitol infusion; caution should be used when considering mannitol therapy. In addition, lack of excretion of mannitol may also result in substantial hyperosmolality. Similarly, administration of high doses of furosemide in kidney injury has been associated with ototoxicity. When using diuretic therapy in children with AKI, potential risks and benefits need to be considered. When the neonate is unresponsive to therapy, administering continued high doses of diuretics is not justified and unlikely to be beneficial to the neonate. In neonates who do respond to therapy, continuous infusions may be more effective and may be associated with less toxicity than bolus administration.
The use of “renal”-dose dopamine (0.5–3.5 µg/kg per minute) to improve kidney perfusion after an ischemic insult in NICUs and PICUs was practiced in the past, but has now fallen out of favor. Although dopamine increases kidney blood flow by promoting vasodilatation and may improve urine output by promoting natriuresis, no definitive studies have demonstrated that low-dose dopamine is effective in decreasing the need for dialysis or improving survival in patients with AKI. In fact, a placebo-controlled randomized study of low-dose dopamine in adult patients demonstrated that low-dose dopamine was not beneficial and did not confer clinically significant protection from kidney dysfunction. Other studies have demonstrated that renal-dose dopamine is not effective in the therapy of AKI, and one study demonstrated that low-dose dopamine worsened kidney perfusion and kidney function.
Fenoldopam is a potent short-acting selective dopamine-1 receptor agonist that decreases vascular resistance while increasing kidney blood flow. A meta-analysis of 16 trials of fenoldopam in adults concluded that therapy with fenoldopam decreased the incidence of AKI, decreased the need for renal replacement therapy, decreased the length of ICU stay, and decreased death from any cause. Fenoldopam has been used in a few children with AKI, including two children receiving therapy with a ventricular-assist device as a bridge to cardiac transplantation; therapy with fenoldopam was thought to avoid the need for renal replacement therapy in one child. Yoder et al. retrospectively studied the effect of low dose fenoldopam on urine output, blood pressure, renal function, and electrolyte balance in neonates and found that fenoldopam did not improve urine output or renal function; however no adverse cardiac consequences were noted. Ricci et al. performed a prospective controlled trial in newborn infants on cardiopulmonary bypass to determine if the use of fenoldopam in neonates on conventional diuretics improved renal function. Similar to the findings by Yoder et al., they did not find a reduction in AKI or improvement in urine output. Additional studies utilizing fenoldopam need to be performed in children and neonates with AKI.
Electrolyte Management
Mild hyponatremia is very common in AKI and may be attributable to hyponatremic dehydration, but fluid overload with dilutional hyponatremia is much more common. If the serum sodium is greater than 120 mEq/L, fluid restriction or water removal by dialytic therapy will correct the serum sodium. However, if the serum sodium is less than 120 mEq/L, the neonate is at higher risk for seizures from hyponatremia, and correction to a sodium level of approximately 125 mEq/L with hypertonic saline should be considered.
The kidney tightly regulates potassium balance and excretes approximately 90% of dietary potassium intake, hyperkalemia is a common and potentially life-threatening electrolyte abnormality in AKI in neonates. The serum potassium level may be falsely elevated if the technique of the blood drawing is traumatic or if the specimen is hemolyzed. Hyperkalemia results in disturbances of cardiac rhythm by its depolarizing effect on the cardiac conduction pathways. The concentration of serum potassium that results in arrhythmia is dependent upon the acid-base balance and the other serum electrolytes. Hypocalcemia, which is common in kidney injury, exacerbates the adverse effects of the serum potassium on cardiac conduction pathways. Tall peaked T waves are the first manifestation of cardiotoxicity, and prolongation of the PR interval, flattening of P waves, and widening of QRS complexes are later abnormalities. Severe hyperkalemia will eventually lead to ventricular tachycardia and fibrillation and requires prompt therapy with sodium bicarbonate, IV glucose and insulin, IV calcium gluconate, and albuterol. Albuterol infusions of 400 µg given every 2 hours as needed have been shown to rapidly lower serum potassium levels. All of these therapies are temporizing measures and do not remove potassium from the body. Kayexalate given orally per nasogastric tube or per rectum will exchange sodium for potassium in the gastrointestinal tract and result in potassium removal. Complications of Kayexalate therapy include possible hypernatremia, sodium retention, and constipation. In addition, Kayexalate therapy has been associated with colonic necrosis. Depending upon the degree of hyperkalemia and the need for correction of other metabolic derangements in AKI, hyperkalemia frequently requires the initiation of dialysis or hemofiltration.
The kidney excretes acids generated in the body by diet and intermediary metabolism; therefore acidosis is very common in AKI. Severe acidosis can be treated with IV or oral sodium bicarbonate, oral sodium citrate solutions, or dialysis therapy. When considering treatment of acidosis, it is important to consider the serum ionized calcium level. Under normal circumstances, approximately half the total calcium is protein bound, and the other half is free and in the ionized form, which is what determines the transmembrane potential and electrochemical gradient. Hypocalcemia is common in AKI, and acidosis increases the fraction of total calcium to the ionized form. Treatment of acidosis can then shift the ionized calcium to the more normal ratio, decreasing the amount of ionized calcium and precipitating tetany or seizures.
The kidney excretes a large amount of ingested phosphorus, therefore hyperphosphatemia is a very common electrolyte abnormality noted during AKI. Hyperphosphatemia should be treated with dietary phosphorus restriction and with oral calcium carbonate or other calcium compounds to bind phosphorus and prevent gastrointestinal absorption of phosphorus. Given that most neonates with AKI have hypocalcemia, the use of calcium-containing phosphate binders provides a source of calcium as well as phosphate-binding capacity.
In many instances AKI is associated with marked catabolism, and malnutrition can develop rapidly, leading to delayed recovery from AKI. Prompt and proper nutrition is essential in the management of the newborn with AKI. If the gastrointestinal tract is intact and functional, enteral feedings with formula (PM 60/40) should be instituted as soon as possible. If the newborn is oligo/anuric and sufficient calories cannot be achieved while maintaining appropriate fluid balance, the earlier initiation of dialysis should be instituted.
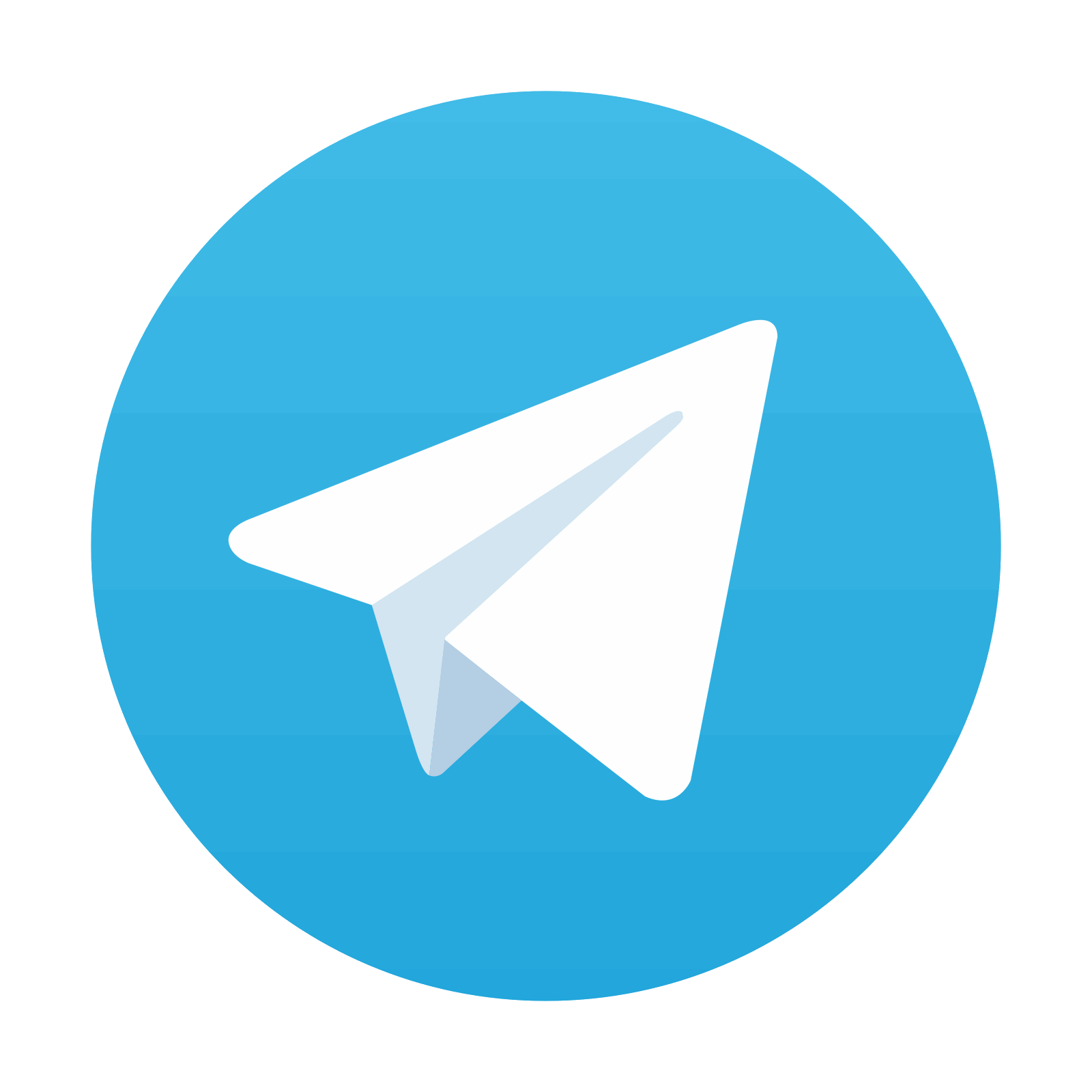
Stay updated, free articles. Join our Telegram channel

Full access? Get Clinical Tree
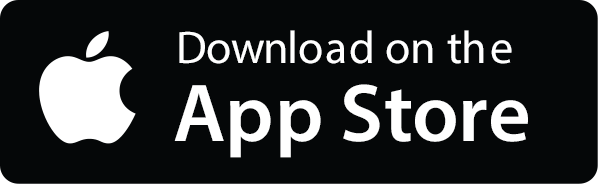
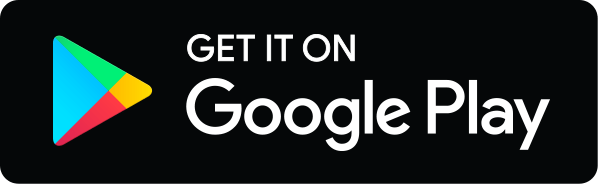