Karl L. Womer
Immunologic Principles in Kidney Transplantation
Important to an effective immune response is the ability of T cells to recognize a wide variety of nonself antigens, which allows for restrained immune activation and subsequent antigen-specific killing. This task is accomplished through the generation of a diverse repertoire of T cells in a single individual with specificity for an enormous number of potential foreign antigens presented as peptides on the surface of major histocompatibility complex (MHC) molecules. Variations in MHC structure from individual to individual increase the variety of peptides that can be presented to T cells, which protects the species as a whole by ensuring adequate T cell responses to a given foreign organism in at least one member of the population. Although slight, these MHC polymorphisms are recognized as foreign after kidney transplantation between non–genetically identical humans and induce alloresponses that in the absence of immunosuppression result in rejection of the allograft (see Box 100-1 for graft terminology). In this chapter, basic immunologic principles important to the field of kidney transplantation are reviewed.
Ischemia-Reperfusion Injury
The immunologic responses after kidney transplantation occur in well-defined stages,1 as depicted in Figure 100-1. Initial insults to the graft during donor organ procurement and subsequent transplantation into the recipient are referred to as ischemia-reperfusion injury (IRI). The pathogenesis of IRI involves biochemical, cellular, vascular endothelial, and tissue-specific factors, with inflammation as a common feature.2 Acute ischemia leads to tissue damage and endothelial cell activation that initiates the innate or antigen-nonspecific immune response. Innate immunity occurs rapidly, with limited specificity, and without memory and includes both cellular elements (neutrophils, macrophages, dendritic cells [DCs], and natural killer [NK] cells) and molecular components (Toll-like receptors [TLRs], complement proteins, chemokines, and cytokines, among others).
Toll-like receptors are expressed by a number of cells, including monocytes and macrophages, lymphocytes, DCs, NK cells, neutrophils, and epithelial and endothelial cells. Engagement of TLRs by endogenous molecules called danger-associated molecular patterns (DAMPs) that are released by cells during IRI leads to release of cytokines, including tumor necrosis factor α (TNF-α) and interleukin (IL)–6. These proinflammatory mediators induce tubular epithelial cell production of the chemokine CXCL8 (IL-8), which attracts neutrophils by activation of their surface chemokine receptor, CXCR2. Neutrophil accumulation is the prime cellular mediator of microvascular plugging and local tissue destruction in IRI. In the next phase, monocyte or macrophage infiltration occurs and likely contributes to extension of early injury as well as repair.3 These events contribute to delayed graft function and amplify the adaptive or antigen-specific phase of transplantation immunity (T cells and antibody-producing B cells) that can negatively affect long-term graft survival. NK cells likely also function as a bridge between innate and adaptive immunity in IRI, in part through bidirectional crosstalk between NK cells and DCs that plays a relevant role in the mechanisms leading to activation and maturation of DCs.4 Likewise, whereas activation of the complement system plays an important role in IRI as a manifestation of the innate immune system, complement may also regulate adaptive immune responses. T and B cells constitute the primary arms of the adaptive immune response, but they also play an important role in the acute and possibly healing phases of IRI.2 The immunologic events described here are not specific to allografts, as they also occur in syngeneic grafts during IRI.
Antigen Presentation
Antigen-Presenting Cells
Antigen-presenting cells (APCs) are specialized cells capable of activating T cells. Antigen is endocytosed by APCs and then displayed by MHC molecules on their surface. T cells recognize and interact with the antigen-MHC complex to become activated. DCs, macrophages, and B cells are all considered “professional APCs,” although DCs are the most potent at antigen presentation. Alloimmune responses are initiated by activation of APCs (mostly DCs) through innate immune recognition systems, as described previously. DCs are highly versatile cells, determining whether the environment indicates that antigen should lead to an immune response or alternatively to tolerance.5 In the graft and surrounding tissues after transplantation, DCs of either donor or host origin become activated and move to T cell areas of secondary lymphoid organs (SLOs).
The trafficking pattern of naive T cells is restricted to SLOs, such as the lymph node and spleen, but also possibly to tertiary lymphoid structures formed in tissues after inflammation, including those in allografts.6 They traverse from blood to lymphoid organs, where they pass through the T cell areas and become activated on encounter with donor antigen (alloantigen) presented by activated DCs in the context of MHC molecules. Thus, the movement of DCs and naive T cells is coordinated to bring them into contact in the T cell areas of SLOs, which appears essential for effective priming.7 Once activated, T cells are able to leave SLOs via lymph vessels to enter the blood and ultimately peripheral tissues, particularly into sites of inflammation. B cells are activated when antigen engages their antigen receptors, initially in the border of T cell and B cell areas of SLOs, where helper T cell function is provided.8
Antigen experienced memory cells may be activated by other APCs, such as graft endothelium.9 Patients normally have no preexisting immunoreactivity to alloantigen, unless they have been exposed to alloantigen through pregnancy, blood transfusion, or prior transplantation. However, microbial antigens that cross-react with alloantigens (molecular or antigenic mimicry) can lead to the generation of alloantigen-specific memory cells through a process termed heterologous immunity.10
T Cell Ontogeny and Major Histocompatibility Complex Specificity
Allografts induce alloimmune responses because of the recognition of nonself antigens (e.g., MHC) from the graft by recipient T cells. During T cell ontogeny during embryogenesis, multilineage bone marrow precursors migrate to the thymus, where ultimate rearrangement of the T cell receptor (TCR) gene occurs, resulting in irrevocable T cell commitment. TCR gene rearrangement is random and ensures that a diverse repertoire of T cells exists to be able to respond to the enormous number of potential foreign antigens.11 The mature T cell repertoire is determined in the thymus by two processes, positive and negative selection. Positive selection is dependent on a certain degree of antigen-specific T cell affinity to self-MHC molecules expressed on thymic cortical epithelial cells. This process ensures that mature T cells will interact effectively with MHC to allow recognition of foreign antigen in the context of self-MHC. Negative selection then occurs by deletion of T cells with excessively high affinity for self-peptide and MHC, thereby preventing release of high-affinity T cells with autoimmune potential.
Mature T cells expressing their clone-specific TCRs then exit the thymus as either CD4 or CD8 T cells. The TCRs of CD4 T cells (also called helper T cells) are selected to interact with class II MHC molecules, whereas the TCRs of CD8 T cells (precursors of cytotoxic T lymphocytes or CTLs) interact with class I MHC molecules. A fundamental principle of immunology is that T cells do not recognize intact foreign proteins directly, but instead as peptides presented by self-MHC on APCs. However, allelic variation among MHC molecules from individual to individual is quite small, resulting in similarities between donor and recipient MHC structure. Thus, unique to the transplant setting, recipient TCRs have a strong affinity for intact donor MHC molecules and can recognize them directly, which explains in large part the high proportion of T cells responding to alloantigen.12 In fact, only 1/105 to 1/106 T cells will respond to any given nominal antigen (e.g., peptide derived from tetanus toxin or influenza hemagglutinin). However, the frequency of T cells that respond to foreign MHC molecules (alloantigens) is much higher (up to 5% to 10% of all T cells).
Pathways of Allorecognition
Recipient T cells may encounter alloantigen by either the direct or indirect pathways of allorecognition (Fig. 100-2). As previously alluded to, direct antigen presentation involves recipient T cell recognition of donor MHC peptides in the peptide groove of intact donor MHC molecules on the surface of donor APCs. By this mechanism, donor APCs migrate from the graft to recipient lymphoid organs and activate alloreactive recipient T cells to initiate the alloimmune response. It has been suggested that the direct pathway may be particularly active early after transplantation, when a large number of donor APCs are present in the allograft. However, direct antigen presentation may also occur later when recipient T cells recognize intact donor MHC molecules on cells of the graft (e.g., endothelium). The best evidence of direct allorecognition is the strong in vitro response generated in the mixed lymphocyte reaction (MLR), whereby lymphocytes are cultured with allogeneic APCs. B cells also recognize intact donor MHC antigen via their B cell receptors (BCRs).
Indirect antigen recognition is the physiologic mechanism of foreign antigen presentation. Foreign antigen is taken up by APCs, processed intracellularly, and then presented as peptides on MHC molecules. During indirect allorecognition, donor MHC molecules are shed from the graft and processed by recipient APCs, where they are presented as peptides to recipient T cells in the context of recipient MHC molecules.13 Because donor MHC molecules are continually shed from the graft and presented by recipient APCs, indirect allorecognition may play a larger role in the late alloresponse, including chronic rejection. However, the relative contribution of direct versus indirect allorecognition to the alloresponse at different time points after transplantation remains the subject of debate.
Major Histocompatibility Complex
Class I and II MHC molecules are designed for presentation of antigen from different sources for different purposes (Fig. 100-3). The class I system is designed to sample cytosolic proteins to detect tumors or intracellular pathogens, such as virus and intracellular bacteria. Class I molecules are recognized by CD8 T cells and thus provide a surveillance mechanism to target infected or malignant cells for destruction by CTLs. The class II system is designed to sample extracellular proteins that have been taken up and processed by APCs. Class II molecules are recognized by CD4 T helper cells and allow for the generation of immune responses to invading pathogens that are phagocytosed by APCs. Cross-presentation is a process by which certain APCs take up, process, and present extracellular antigen on class I molecules to CD8 T cells.14 This mechanism is necessary for immunity against tumors and viruses that do not infect APCs. MHC products also play other important roles, including the positive and negative selection of developing T cells described earlier, stimulation of naive and memory T cells that is necessary for their survival (homeostatic proliferation), the induction of T cell tolerance and anergy, and interaction with NK cells and other inhibitory and activating receptors.
The MHC gene locus (in humans, human leukocyte antigen [HLA]) maps to a 3.5-million–base-pair region on the short arm of chromosome 6 and is divided into three regions: the class II region, the class III region, and the class I region. Only the class I and II regions encode proteins involved in antigen presentation. The key MHC genes are the class I genes (HLA-A, HLA-B, and HLA-C) and the class II genes (HLA-DP, HLA-DQ, and HLA-DR). The class I and II proteins share overall structural homology but are functionally different.15 The molecular structure of MHC class I and II proteins is presented in Figure 100-3.
Class I proteins are expressed on virtually all nucleated cells, although the amount expressed varies. Class II proteins have a more restricted cell distribution, generally limited to bone marrow–derived professional APCs, including DCs, B cells, macrophages, and Langerhans cells, but also other cells, including renal parenchymal and endothelial cells. Both class I and II antigens can be induced on a variety of cells by interferon-γ (IFN-γ) in synergy with other cytokines during rejection episodes.
Human Leukocyte Antigen Typing and Transplantation
Major histocompatibility complex class I and II genes are highly polymorphic in the regions that encode the peptide-binding groove. These polymorphisms help to ensure survival of the species by increasing the variety of peptides that can be presented to T cells. Thus, MHC polymorphisms decrease the chance of encountering pathogens that induce poor immune responses within a population and therefore could lead to the demise of the species.16 However, it is also these polymorphisms that predispose to allograft rejection, because the antigen presenting structures of one individual are regarded as foreign by another nonidentical individual.
Originally, polymorphisms were defined by HLA serologic (antibody) typing using sera from multiparous women or persons who had received blood transfusions. The development of molecular biology techniques (polymerase chain reaction [PCR] sequencing) has allowed the analysis of the HLA allelic sequence diversity at the DNA level. By DNA typing, many more polymorphisms (alleles) have been identified: currently 2188 for HLA-A, 2862 for HLA-B, 1746 for HLA-C, and 1393 for HLA-DR, with an increasing number of sequences added each year. The most currently updated source for HLA alleles can be identified at the website www.ebi.ac.uk/imgt/hla. Although PCR techniques allow for rapid DNA sequence-based typing of human populations, serologic methods are still often used for identifying HLA antigens in kidney transplantation.
Human Leukocyte Antigen Inheritance
Human leukocyte antigen genes are inherited in a Mendelian co-dominant fashion, meaning that a copy of each HLA gene (i.e., one haplotype) is inherited from each parent and expressed as antigens. HLA typing identifies the specific alleles carried by a person. The term HLA matching means assigning a donor kidney to a recipient with as few mismatches as possible. In kidney transplantation, efforts are made to match HLA-A, HLA-B, and HLA-DR genes and proteins. It can be predicted that siblings from the same set of parents will have a 25% chance of having zero mismatches, a 50% chance of one haplotype mismatch, and a 25% chance of two haplotype mismatches (Fig. 100-4).
Non–Major Histocompatibility Complex Antigens
Minor histocompatibility antigens are normal proteins that are themselves polymorphic within a given species. Even when a transplant donor and recipient are identical with regard to MHC genes, amino acid differences in these minor proteins can lead to rejection. Minor antigens are encoded by a large number of chromosomes and are presented only as peptides in the context of recipient MHC (indirect allorecognition). Minor antigens are responsible for the need for immunosuppression after donation between HLA matched nonidentical twin siblings. The prototypic minor histocompatibility antigen, the male or H-Y antigen, is derived from a group of proteins encoded on the Y chromosome. Alloresponses to this antigen are responsible for rejection of male mouse skin grafts by otherwise identical female recipients and may explain observations of reduced long-term graft survival in human male-to-female donations.
Major histocompatibility complex I-related chain A (MICA) antigens are surface glycoproteins with functions related to innate immunity. Exposure to allogeneic MICA during transplantation can elicit antibody formation.17 ABO blood group glycolipids expressed on endothelial and red blood cells are other notable non-MHC antigens. Finally, immune responses to autoantigens have been associated with allograft damage.18
T Cell Activation
T cells are required for rejection, because animals experimentally deprived of T cells through mutation or genetic manipulation are unable to reject allografts. Alloreactive T cells can be found in the naive and memory T cell populations, but both require recognition of nonself MHC molecules to become activated. Reactions mediated by naive T cells take longer to develop than those mediated by memory T cells, which can be generated more quickly and with higher numbers of cells (secondary response). During allograft rejection, both populations are activated simultaneously.
T Cell Receptor
Each T cell bears about 30,000 identical antigen-receptor molecules. Each receptor consists of two different polypeptide chains, termed the TCR α and β chains, which are linked by a disulfide bond. The genes encoding the TCR chains are members of the immunoglobulin (Ig) supergene family, which are found on B, T, and NK cells. The α:β heterodimers are very similar in structure to the Fab fragment of an Ig molecule and account for antigen recognition by most T cells (Fig. 100-5). In contrast to the Ig receptors of B cells, TCRs do not recognize antigen in its native state, but instead recognize a composite ligand of a peptide bound to an MHC molecule. A minority of T cells bears an alternative, but structurally similar, receptor composed of a different polypeptide heterodimer (γ:δ).
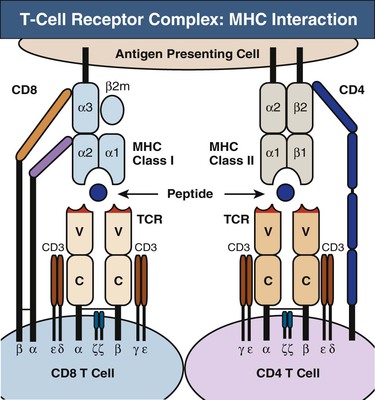
CD4 and CD8 Coreceptors
T cells fall into two major classes with different effector functions, distinguished by the expression of the cell-surface proteins CD4 and CD8 (see Fig. 100-5). Both CD4 and CD8 have a cytoplasmic tail that can associate with signaling proteins important in T cell activation. CD4 and CD8 binding to MHC are required to make an effective response. Thus, these molecules are called coreceptors.
T Cell Receptor Engagement of Antigen: Signal 1
The TCR α:β heterodimer recognizes and binds its peptide-MHC ligand19 but cannot signal to the cell that antigen has bound. In the functional receptor complex, α:β heterodimers are associated with a complex of four other signaling chains (two ε, one δ, one γ) collectively called CD3 (see Fig. 100-5). Engagement of the TCR by MHC, along with the other required receptor engagements, initiates the signaling process from the CD3 complex, leading to cell proliferation and differentiation.20
T Cell Costimulation: Signal 2
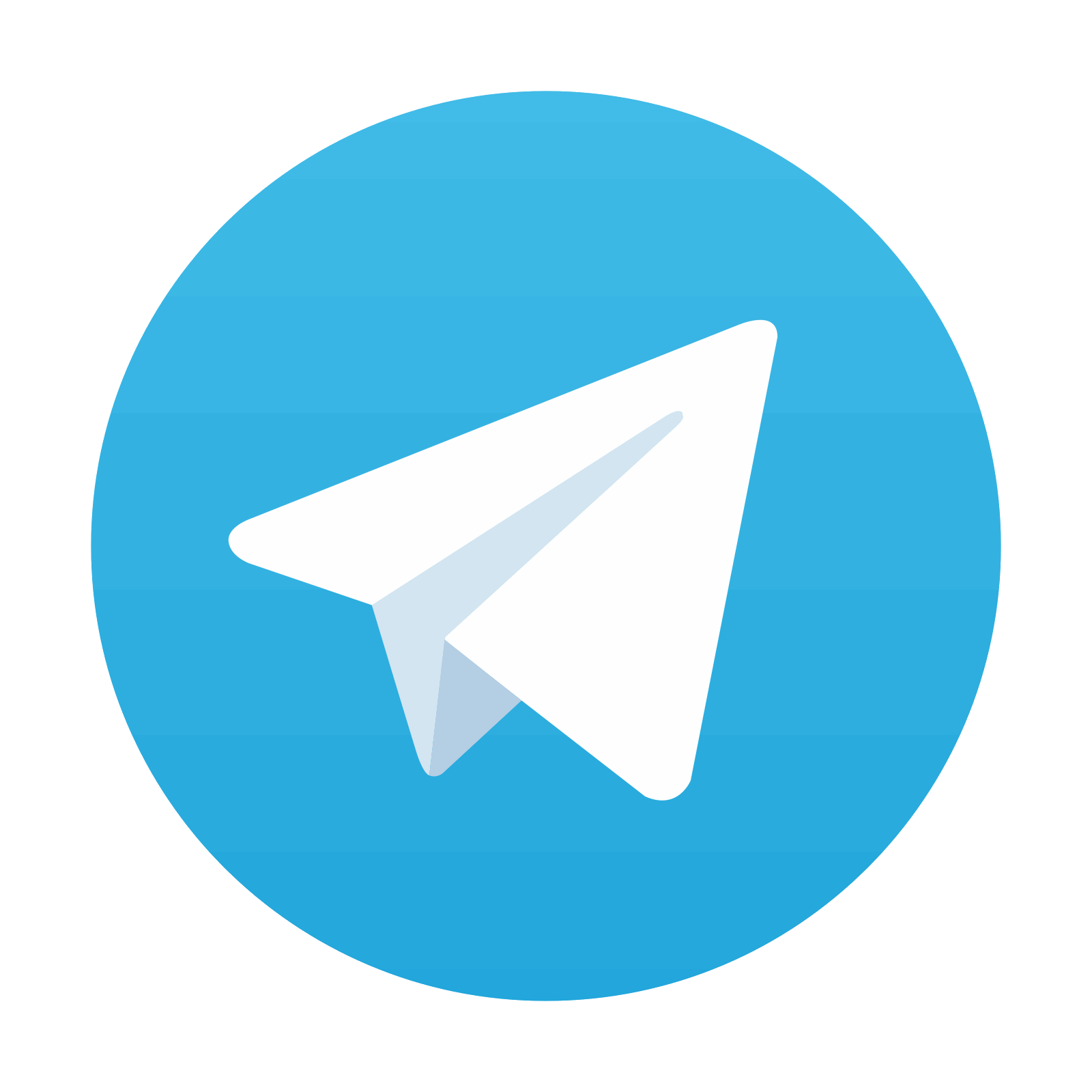
Stay updated, free articles. Join our Telegram channel

Full access? Get Clinical Tree
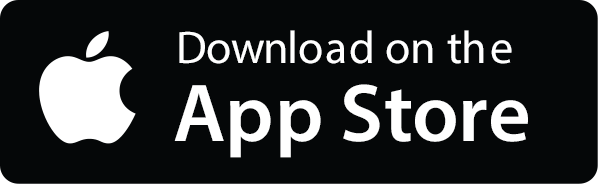
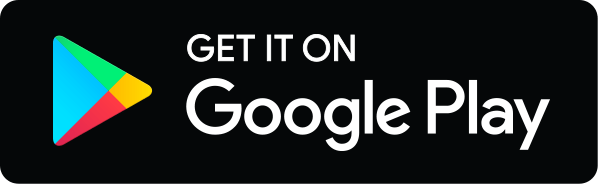