Sodium and its accompanying anions are the principle osmotically active solutes in extracellular fluid. When extracellular osmolality is low, intracellular osmolality is equally low. Therefore, although there are exceptions (Table 44.1), hyponatremia is usually associated with hypoosmolality and dilution of all body fluids.
The Plasma Sodium Concentration and Body Fluid Tonicity
Sodium and its accompanying anions are the principle osmotically active solutes in extracellular fluid. When extracellular osmolality is low, intracellular osmolality is equally low. Therefore, although there are exceptions ( Table 44.1 ), hyponatremia is usually associated with hypoosmolality and dilution of all body fluids.
Plasma Osmolality | Disorder | Pathogenesis |
---|---|---|
Normal | Pseudohyponatremia | Excess non-aqueous material decreases plasma water content; no change in ECF or ICF volume |
Hyperlipidemia | ||
Multiple myeloma | ||
Exogenous solutes | Expansion of ECF volume with non-sodium solutes and water; no change in ICF volume | |
Isotonic IV mannitol | ||
Irrigant absorption | ||
Increased | Hyperglycemia, hypertonic IV mannitol, and maltose containing IgG solutions | Initial expansion of ECF volume and shift of water out of cells; decrease in ICF volume |
When (as is usually the case) the concentration of non-permeant extracellular solutes other than sodium is very low, the plasma sodium concentration is a function of three variables, as indicated by the following equation:
Plasma [ Na + ] ≅ Exchangeable Na + + Exchangeable K + Total body water
Only the exchangeable fractions of sodium and potassium are included in the equation, because one-third of body sodium is bound to bone and osmotically inactive. This relationship, which has been validated empirically, indicates that the plasma (or serum) sodium concentration can be reduced by depletion of body cations, by an increase in body water or by a combination of these processes. Recently, it has been emphasized that the original equation describing the relationship between the plasma sodium concentration, exchangeable sodium, exchangeable potassium, and total body water has an intercept that can be explained theoretically. The simplified form of the relationship Eq. (44.1) , which omits the intercept, is useful conceptually, but should not be considered a completely accurate basis for predicting the effect of therapy on the plasma sodium concentration.
It is intuitively obvious that the extracellular sodium concentration should be proportional to the body’s content of water and soluble sodium. The sodium concentration falls when the body retains water (without solute) or when there are net external losses of sodium (without water). The importance of intracellular potassium stores to the plasma sodium concentration is less obvious. In potassium depletion, sodium ions move intracellularly as intracellular potassium is lost, balancing negative charges on intracellular macromolecules. Thus, external loss of exchangeable potassium causes an internal loss of extracellular sodium. Similarly, when intracellular potassium is replaced by hydrogen ions, rather than sodium or when it is lost with phosphate (an intracellular anion), the loss of osmotically active intracellular solute causes a redistribution of water from the intracellular to the extracellular fluid compartments, diluting extracellular sodium ions.
Physiologic Control of Water Excretion
Osmotic Regulation
Controlled by changes in water intake, vasopressin secretion, and water excretion, the plasma sodium concentration is normally prevented from rising above 142 mEq/L or falling below 135 mEq/L. When the plasma sodium concentration changes by as little as 1% (with a corresponding change in plasma osmolality), cell volume receptors (“osmoreceptors”) in the hypothalamus respond, relaying signals to vasopressin-secreting neurons located in the supraoptic and paraventricular nuclei whose axons terminate in secretory bulbs in the neurohypophysis. The antidiuretic hormone, arginine vasopressin, which is released into the systemic circulation by the neurohypophysis, controls water excretion by the kidneys. The hormone activates V 2 receptors on the basolateral membrane of principal cells in the renal collecting duct, initiating a cyclic AMP-dependent process that culminates in increased production of water channels (aquaporin 2), and their insertion into the cells’ luminal membranes. The effect of vasopressin on water flow is inhibited by locally produced prostaglandin E 2 , which is stimulated by vasopressin action on V 1 receptors. Vasopressin’s short half-life in the circulation and continuous shuttling of aquaporins between the collecting duct’s cell membrane and cytosol allow rapid changes in urinary water excretion in response to changes in body fluid tonicity.
Vasopressin levels are normally unmeasurable when the plasma sodium concentration falls to approximately 135 mEq/L. Low levels of the hormone allow the excretion of large volumes of a maximally dilute urine (≈50 mOsm/kg) which reduces body water content and restores the plasma sodium concentration to normal. At higher plasma sodium levels, plasma vasopressin is directly related to the plasma sodium concentration, reaching levels that are high enough to promote the excretion of maximally concentrated urine (≈1200 mOsm/kg) at a plasma sodium concentration of approximately 142 mEq/L. A rising plasma sodium concentration also stimulates thirst. Ingested water is retained, returning the plasma sodium concentration back towards normal.
Hemodynamic Regulation
Under day-to-day conditions, vasopressin secretion, urinary free water excretion, and thirst respond primarily to changes in body fluid tonicity. Under pathologic conditions, osmotic control of vasopressin secretion and thirst can be overridden by hemodynamic stimuli. In addition to input from osmoreceptors, the hypothalamic neurons that secrete vasopressin also receive neural input from baroreceptors in the great vessels, and volume receptors in the atria. When these receptors are stimulated by hypotension or by a major reduction in plasma volume, impulses are carried via cranial nerves IX and X to the hypothalamus. The thirst center in the hypothalamus responds to similar non-osmotic stimuli. Vasopressin and thirst responses to hypovolemia and hypotension lead to water retention, despite hypotonicity of body fluids. These hemodynamic responses can be regarded as back-up systems that serve to maintain arterial blood volume under emergency conditions, sacrificing tonicity to tissue perfusion. Although high levels of vasopressin occur in response to hypovolemia, under experimental conditions, a rather large stimulus is required; while plasma vasopressin is measurably increased by a 1% change in plasma osmolality, a 10% change in extracellular fluid volume is required to elicit the same response. However, these experimental findings are difficult to reconcile with clinical observations, suggesting that non-osmotic vasopressin secretion occurs with more subtle volume depletion.
Hypotonic Hyponatremia: Classification and Pathogenesis
Traditionally, patients with hyponatremia are divided into categories according to their body sodium content and/or intravascular volume: low body sodium content (volume depletion); high body sodium content (edematous conditions) or normal body sodium content (euvolemic hyponatremia or SIADH). Although this time-honored approach is often helpful to clinicians, intravascular volume and body sodium content do not always change in parallel (e.g., self-induced water intoxication), and some causes of hyponatremia (e.g., diuretic-induced and cerebral salt-wasting) may be difficult to classify by intravascular volume. Moreover, physiologic responses to extracellular volume expansion and contraction often create ambiguities in volume status. Thus, secondary water retention in response to volume depletion and secondary natriuresis in response to water overload may ultimately yield similar values for total body sodium and water ( Figure 44.1 ).

Table 44.2 classifies hyponatremia by the physiologic mechanism underlying the electrolyte disturbance. As the plasma sodium concentration is proportional to the ratio of exchangeable cations and total body water, it follows that changes in sodium concentration are related to external balances of sodium, potassium, and water. However, because the plasma sodium concentration is normally maintained within a narrow physiologic range by control systems which regulate water balance, hypotonic hyponatremia can only occur if water excretion is impaired or overwhelmed. The various causes of hyponatremia are therefore divided according to the status of urinary water excretion. Disordered water balance is often accompanied by changes in cation balance, which also play a pivotal role in the pathogenesis of hyponatremia.
Urine Diluting Ability | Cause of Hyponatremia |
---|---|
Unimpaired | Psychotic polydipsia |
Beer potomania | |
Infantile water intoxication | |
Impaired: vasopressin-independent | Oliguric renal failure |
Tubular interstitial renal disease | |
Diuretics | |
Nephrogenic syndrome of antidiuresis a | |
Impaired: vasopressin-dependent | Hemodynamically-mediated |
Volume-depletion | |
Spinal cord disease | |
Congestive heart failure | |
Cirrhosis | |
Addison’s disease b | |
Cerebral salt-wasting b | |
SIADH (see Table 44.3 ) |
a Hereditary disorder of the V2 receptor with clinical features of SIADH, but with undetectable plasma vasopressin levels.
b Disorders with both hemodynamic and non-hemodynamic bases for vasopressin release.
Water Intoxication with Maximally Dilute Urine
Pathophysiology
Rarely, fluid intake can overwhelm normal mechanisms for water excretion. In the absence of vasopressin, urine osmolality falls to approximately 50 mOsm/kg. A typical American diet provides a daily load of 600 to 900 milliosmoles of solute (electrolytes and urea) that must be excreted. At this rate of solute excretion, the volume of maximally dilute urine equals 12 to 18 liters per day or 500 to 750 ml/hour. Water intake can occasionally exceed this large excretory capacity. Patients with severe acute water intoxication are truly “water-logged,” and susceptible to pulmonary edema due to retained water.
Self-Induced Water Intoxication in Psychotic Polydipsia
Polydipsia and polyuria are extremely common among institutionalized patients with mental illness. Many patients with polydipsia have frequent episodes of hyponatremia, which may present with seizures. About half the reported cases have had maximally dilute urine (urine osmolalities below 100 mOsm/kg) at presentation. In others, inappropriately concentrated urine was present immediately following seizures or in association with nausea, but the rate of correction of hyponatremia indicates that the urine became dilute soon afterwards.
In most psychotic water drinkers, hyponatremia can be ascribed to a generalized dilution of body solutes by retained water; thus, body weight increases in proportion to the severity of hyponatremia. Patients gain weight and become hyponatremic during the course of the day, and then spontaneously diurese, normalizing their plasma sodium concentration and body weight during the night. Caregivers in psychiatric hospitals routinely monitor weight changes in patients who are habitual water drinkers to determine when access to water must be rigidly restricted to avoid severe symptomatic hyponatremia.
Agents that interfere with the ability to maximally dilute the urine (e.g., diuretics or carbamazepine) should be avoided in polydipsic patients, as they can precipitate a rapid onset of life-threatening hyponatremia.
Water Intoxication in Infants
Acute water intoxication is common among infants who are given excessively dilute formula. The hungry infant ingests large volumes of fluid leading to water retention, despite the excretion of maximally dilute urine. Once water is restricted, the plasma sodium concentration self-corrects as large volumes of urine are excreted.
Beer Drinkers Potomania
Alcoholics who eat little and subsist on large volumes of beer may also become hyponatremic while excreting maximally dilute urine. Beer’s low protein content and the protein-sparing effect of its carbohydrate result in profoundly reduced blood urea nitrogen concentrations and urinary urea excretion. The total daily excretion of urinary solute may be only 200 to 300 milliosmoles. Thus, even at a urine osmolality of 50 mOsm/kg, urine output is limited to 4 to 6 liters per day, an amount that fails to match the enthusiastic beer drinker’s intake of electrolyte free water. A similar phenomenon has been reported in non-beer drinkers with a high fluid and low protein intake. Volume-depletion from gastrointestinal losses, and transient vasopressin release caused by nausea or alcohol withdrawal, may further limit the beer drinker’s ability to excrete free water, contributing to the development and persistence of hyponatremia.
Vasopressin-Independent Defects in Water Excretion
Pathophysiology
Maximal free water excretion depends on adequate delivery of glomerular filtrate to the renal diluting segments (the ascending limb of the loop of Henle and the distal tubule), reabsorption of salt without water by the diluting segments to create hypotonic fluid within the tubular lumen, and a collecting duct that is relatively impermeable to water, so that the dilute tubular fluid formed “upstream” can be eliminated in the final urine. Hyponatremia occurs when water is taken in at a time when these mechanisms are not functioning normally.
Renal Failure
The most obvious cause of impaired water excretion is oliguric renal failure. Even when nonoliguric, patients with advanced renal failure have fixed isosthenuria, and are unable to excrete dilute urine despite normally suppressed vasopressin secretion. In the absence of renal failure, urinary dilution can still be impaired, despite low levels of vasopressin, by two mechanisms: (1) enhanced proximal reabsorption of the glomerular filtrate, limiting fluid delivery to the renal diluting segments (as in volume depletion, congestive heart failure, and cirrhosis) ; and (2) impaired sodium reabsorption in the renal diluting segments (by diuretics or tubular interstitial disease).
Diuretic-Induced Hyponatremia
Both thiazides and loop diuretics interfere with the ability to maximally dilute the urine. Thus, both classes of diuretic can lead to water intoxication in patients who habitually ingest extremely large volumes of water. Diuretics are one of the most important causes of hyponatremia. Most cases are caused by thiazide or thiazide-like agents; loop diuretics are implicated much less commonly. Thiazides may be the sole factor responsible for causing hyponatremia, and they may also exacerbate hyponatremia in patients with disorders associated with SIADH. The mechanism of thiazide-induced hyponatremia remains somewhat unclear; however, as for all causes of hyponatremia, water retention and/or cation depletion must be responsible. There is evidence that thiazides have a direct antidiuretic effect mediated by upregulation of aquporin 2 (AQP2).
Most cases of thiazide-induced hyponatremia have occurred in elderly small women who have been prescribed diuretics for the treatment of hypertension. The impairment of renal diluting ability caused by thiazides is more pronounced in elderly people, especially those who have previously experienced thiazide-induced hyponatremia. The predisposition of elderly women to severe hyponatremia may be explained by body size, in that small changes in body water and electrolyte content can lead to marked changes in serum sodium.
In susceptible individuals, the serum sodium may fall within hours of diuretic ingestion, and severe hyponatremia can develop in less than two days. While in many cases the diuretic had been recently prescribed, in others thiazides had been used chronically without incident until, for some reason, water intake increased, dietary salt and protein intake decreased or an intercurrent illness led to “inappropriate” antidiuretic hormone secretion. Mild hyponatremia often persists for a few weeks when diuretic therapy is withdrawn from patients with diuretic-induced hyponatremia, apparently reflecting temporary “resetting” of the osmostat or alternatively, slow restoration of depleted cation stores.
Although thiazide diuretics do not inhibit the ability to concentrate the urine, they do impair diluting ability in several ways : inhibition of electrolyte transport at the cortical diluting sites; direct stimulation of vasopressin release; direct upregulation of AQP2; reduction of glomerular filtration; and enhancement of fractional proximal water reabsorption, reducing delivery to diluting sites.
Positive water balance during the onset of thiazide-induced hyponatremia and negative balance during its correction have been documented. Some patients with thiazide-induced hyponatremia have low serum uric acid levels and high uric acid clearances (markers of volume expansion) which return to normal as the serum sodium normalizes. Most affected patients drink large amounts of water, and the superimposed diuretic prevents urine output from keeping pace with water intake.
Although increased total body water often contributes to the pathogenesis of thiazide-induced hyponatremia, there are many cases in which body weight decreased or remained the same during the fall in serum sodium. In others, direct measurements of total body water in affected patients have been normal. In these cases, other explanations for hyponatremia must be sought.
Negative cation balance plays a major role in the pathogenesis of diuretic-induced hyponatremia. Rejected cations may be excreted at a total concentration which exceeds that of plasma, directly “desalinating” the plasma even in the absence of water intake. Potassium depletion is an important factor in many cases; treatment of hypokalemia has been shown to increase the plasma sodium concentration with no change in body weight. Magnesium repletion may act similarly, presumably through an effect on skeletal muscle Na-K-ATPase. Surprisingly, despite negative cation balance, many patients appear to be euvolemic. Apparently, enough water is retained to offset the initial tendency toward hypovolemia. Once diuretics are withdrawn, urinary sodium excretion falls to very low levels.
Vasopressin-Dependent Defects in water Excretion
Pathophysiology
Normally, in response to hypotonicity, vasopressin secretion is suppressed, the collecting duct is impermeable to water, and a maximally dilute urine is formed. In two large surveys of hospitalized patients with hyponatremia, over 90% of cases were associated with elevated vasopressin levels. Vasopressin levels are rarely elevated into pathologic ranges, even in cases associated with ectopic secretion by tumors. Rather, vasopressin levels are inappropriately high relative to the plasma osmolality. Non-osmotic vasopressin secretion may be an adaptive response driven by hemodynamic stimuli or it may be “inappropriate” and independent of any of the usual physiologic mechanisms which regulate water excretion. Persistent vasopressin secretion despite hypoosmolality allows ingested or infused free water to be retained, causing hypotonic hyponatremia. Vasopressin-mediated hyponatremia is characterized by urine which is more concentrated (usually much more) than 100 mOsm/kg and which becomes more dilute after administration of a V2 receptor antagonist (see section “V 2 -Receptor Antagonists”).
Patients with inappropriate vasopressin secretion must take in water to become hyponatremic. In some cases, hyponatremia develops when electrolyte-free water is administered parenterally. More commonly, patients become hyponatremic while ingesting water. Theoretically, osmotic inhibition of thirst should prevent water ingestion when the ability to excrete water is impaired. However, patients with SIADH continue to drink despite plasma osmolalities below the normal osmotic threshold for thirst. Formal testing has shown that there is downward resetting of the osmotic threshold for thirst in SIADH, but that thirst responds to osmotic stimulation and is suppressed by drinking around the lowered set-point.
Escape from Vasopressin-Induced Water Retention
Experimentally, after several days of constant vasopressin infusion and constant water intake, there is an escape from the antidiuretic effect of vasopressin. With the onset of vasopressin escape, the urine becomes less concentrated, allowing water balance to be re-established at a new steady-state in which the plasma sodium concentration stabilizes at a level lower than normal. In experimental models, escape is temporally associated with a marked decrease in renal aquaporin-2 protein, accompanied by suppression of aquaporin-2 mRNA levels ( Figure 44.2 ). V2-receptor mRNA expression and binding are decreased, as is c-AMP production in response to vasopressin, Plasma and urine aldosterone and mean arterial pressure are increased as are thiazide-sensitive Na-Cl co-transporter and ENaC proteins in the distal nephron that are known to be upregulated by aldosterone. Inhibition of nitric oxide synthase or prostaglandin synthesis synergistically inhibit the escape phenomenon, supporting a role for nitric oxide and prostaglandins in mediating vasopressin escape.

In conditions characterized by vasopressin-mediated water retention, (e.g., SIADH, congestive heart failure), renal escape from vasopressin-induced antidiuresis (along with decreased water intake in some cases) permits patients with vasopressin-mediated hyponatremic states to manifest a relatively stable level of hyponatremia, despite continued water intake and continued presence of vasopressin.
Hemodynamic Causes of Vasopressin-Mediated Hyponatremia
Pathophysiology
Hypovolemia, heart failure, and cirrhosis are the most common non-osmotic stimuli for antidiuretic hormone secretion. In a series of 100 consecutive hospitalized patients with hypotonic hyponatremia, volume contraction (29%), advanced heart failure (25%), and liver cirrhosis (16%) were identified as the cause of hyponatremia in a high percentage of cases. The hemodynamic abnormalities that stimulate vasopressin release in these conditions also promote sodium reabsorption by the renal tubules (mediated by aldosterone, increased sympathetic nervous system activity, peritubular Starling forces, etc.), causing both sodium and water retention. In volume-depletion, sodium retention serves to replace a sodium deficit; in heart failure and cirrhosis, sodium retention serves to compensate for the circulatory abnormality, but it also causes edema.
Volume-Depletion
Sodium and potassium losses associated with gastrointestinal fluids (or with urinary losses caused by osmotic or loop diuretics) do not directly lower the plasma sodium concentration, because these fluids are either hypotonic or isotonic. However, the intravascular volume-depletion caused by such losses is a hemodynamic stimulus for thirst and vasopressin secretion; as a result, ingested water is retained, lowering the plasma sodium concentration. Thus, hyponatremia in these conditions is associated with a reduced content of both total body cations and water. However, in many patients, compensatory water retention makes it difficult to detect the underlying volume depletion. Laboratory clues, including a low urine sodium concentration and elevated serum uric acid levels, can be helpful diagnostically.
Spinal Cord Disease
Hyponatremia is very common after spinal cord injury, particularly among patients with complete quadriplegia. Contributing factors include a large water intake (reflecting physician recommendations, angiotensin II-mediated thirst, and loss of pharyngeal and gastric satiety signals), and baroreceptor-mediated vasopressin release. One study showed normal osmoregulation of vasopressin secretion and excretion of a water-load when subjects were supine, but with the subjects in a sitting position, there was a reduced osmotic threshold and increased sensitivity for vasopressin release, and urine diluting ability and free water clearance were markedly impaired.
Edematous Conditions
Severe hyponatremia can occur despite increased body sodium content if retained sodium is offset by a disproportionate increase in body water.
Congestive Heart Failure
Hyponatremia in heart failure stems from reduced cardiac output and blood pressure which stimulate vasopressin, catecholamines, and the renin–angiotensin–aldosterone axis. Increased vasopressin levels have even been documented in patients with impaired left ventricular function before the onset of symptomatic heart failure. Hyponatremic patients with congestive heart failure have higher levels of plasma renin activity, norepinephrine, epinephrine, and lower renal and hepatic plasma flows than normonatremic patients with an apparently similar degree of heart disease. Hyponatremia in heart failure is associated with a poor prognosis.
Hepatic Cirrhosis
Cirrhosis is characterized by a hyperdynamic circulation with low blood pressure, low systemic vascular resistance, and high cardiac output. Systemic vasodilatation causes relative underfilling of the arterial vascular compartment and neurohumoral responses similar to those that occur in response to a low cardiac output. Activation of the renin–angiotensin–aldosterone axis and the sympathetic system, combined with non-osmotic release of vasopressin, results in renal water and sodium retention. Escape from the sodium-retaining effect of aldosterone does not occur, and there is renal resistance to atrial natriuretic peptide. Although the pathogenesis of the peripheral arterial vasodilation is incompletely understood, increased vascular nitric oxide by the endothelium may play a role. In a rat model of cirrhosis, normalization of vascular nitric oxide production with a nitric oxide synthetase inhibitor corrects the hyperdynamic circulation, improves sodium and water excretion, and decreases neurohumoral activation.
Peritoneovenous shunting of hyponatremic cirrhotic patients with refractory ascites improves cardiac output, renal plasma flow, and creatinine clearance, and results in an immediate diuresis and natriuresis with a decrease in urine osmolality and an increase in plasma sodium concentration associated with a small but significant decrease in plasma vasopressin levels.
Inappropriate Vasopressin Secretion (SIADH)
Pathophysiology
Definitions
Non-osmotic release of vasopressin without a hemodynamic stimulus to account for it is considered “inappropriate”. When Bartter and Schwarz first described the syndrome of inappropriate antidiuretic hormone secretion (SIADH), they defined clinical criteria for the disorder which are still generally accepted: hypoosmolality and clinical euvolemia with a sodium-containing urine (>30 mmol/L) that is less than maximally dilute (>100 mOsm/kg) without recent diuretic use or impaired renal function. Schwarz and Bartter also excluded endocrine disorders–primary and secondary adrenal insufficiency and hypothyroidism–from this designation. We have not made this exclusion, because patients with undiagnosed endocrine disturbances may present with all the clinical features of SIADH. Indeed, the discovery of SIADH is often the presenting feature of a clinically important systemic disease. Abnormal vasopressin secretion may be caused by ectopic production of the hormone by tumors, from disordered secretion by the neurohypophysis or from increased sensitivity to the hormone ( Table 44.3 ).
Major Classification | Common Examples |
---|---|
Tumors | Small-cell lung cancer |
Head and neck tumors | |
Lung diseases | Pulmonary infection |
Hypoxia and hypercarbia | |
Severe asthma | |
Neurologic disorders | Subarachnoid hemorrhage a |
Guillain-Barre syndrome a | |
CNS infections | |
Cerebral hemorrhage and infarction | |
Brain tumors | |
Endocrine diseases | Hypothyroidism a |
Hypopituitarism | |
Isolated ACTH deficiency | |
Medications | Arginine vasopressin and desmopressin acetate |
Amiodarone | |
Chlorpropamide | |
Carbamazepine and oxcarbazepine | |
Cyclophosphamide | |
Nonsteroidal anti-inflammatory agents; serotonin reuptake inhibitors | |
Tricyclic antidepressants | |
Vincristine | |
3,4-methylenedioxymethamphetamine (ecstasy) | |
Hereditary | Nephrogenic syndrome of antidiuresis b |
Miscellaneous, transitory causes | Surgery |
Pain and stress | |
Nausea | |
Alcohol withdrawal |
a Disorders with both hemodynamic and non-hemodynamic bases for vasopressin release.
b Hereditary disorder of the V2 receptor with clinical features of SIADH, but with undetectable plasma vasopressin levels.
Patients with the syndrome of inappropriate antidiuretic hormone secretion (SIADH) retain ingested water, but they have no evidence of volume depletion and no tendency to form edema. Because of water retention, SIADH causes mild, subclinical volume-expansion, which is reflected by high uric acid clearance, a low plasma uric acid concentration, and urine sodium excretion which matches or exceeds sodium intake. Clinicians make use of these characteristics to distinguish SIADH from hyponatremia caused by volume-depletion. The recently described syndrome of nephrogenic inappropriate antidiuresis (see section “Common Causes of SIADH”) is discussed in this section because it exhibits clinical features of SIADH; in physiological terms, however, it is a cause of hyponatremia that is independent of vasopressin, as plasma vasopressin levels are undetectable ( Table 44.2 ).
Patterns of Vasopressin Secretion
In most patients with SIADH, vasopressin secretion has followed one of two basic patterns: “reset osmostat” or “vasopressin leak”. In the reset osmostat variant of SIADH, seen in patients with chronic, debilitating illness and in normal pregnancy, the urine can be diluted maximally, but at a lower set-point than normal. Such patients are thus mildly hyponatremic, but unlike other patients with SIADH, their plasma sodium concentration is very stable and they do not require dietary water restriction or other measures used to treat chronic hyponatremia. In the vasopressin leak variant, the basal level of vasopressin is elevated and unresponsive to osmotic stimuli when the plasma osmolality is low, but the levels increase appropriately when the plasma osmolality increases above a threshold level. Less commonly, patients exhibit erratic vasopressin secretion which is unrelated to osmotic stimuli. In about 10% of patients who present with typical clinical manifestations of SIADH, plasma vasopressin levels are at a low basal level that fails to increase as plasma osmolality increases. Such a pattern would be expected if an antidiuretic factor other than vasopressin were produced or if the collecting tubules were hypersensitive to normal hormone levels.
Interplay of Water Retention and Cation Depletion in SIADH
In SIADH, increased intravascular volume decreases renin secretion and increases release of atrial natriuretic peptide. These volume and hormonal changes promote sodium excretion, despite a low serum sodium concentration. Natriuresis in SIADH blunts the increase in extracellular volume caused by water retention ( Figure 44.1 ), but it also exacerbates hyponatremia ( Eq. (44.1) ). Balance studies in a group of patients with SIADH showed that during a period of high water and low sodium intake, the plasma sodium concentration decreased by 8 mEq/L with no gain in weight and no increase in chloride space (a measure of extracellular fluid volume). Negative sodium and potassium balances accounted for the stability of extracellular volume, and for over 80% of the calculated solute loss. During a period of high sodium intake, over 600 mEq of sodium was retained (with only a small increase in weight and chloride space), fully accounting for the 11 mEq/L increase in serum sodium concentration. In this study, there was a strong negative correlation between water intake and sodium balance, and between water intake and aldosterone secretory rate. Similar findings have been reported in studies in which pituitary extract was administered chronically to normal subjects.
In an experimental model of SIADH produced by DDAVP and half-isotonic saline in the rat, hyponatremia was caused exclusively by negative balances of sodium and potassium; water balance, which was slightly negative, did not contribute to the decrease in sodium concentration. Despite negative balances for sodium, chloride, and water, the extracellular volume (measured by inulin space) was not contracted, suggesting that water shifted from the intracellular to the extracellular space in response to the loss of intracellular solute (potassium and phosphate).
Similarly, direct measurements of body composition in a rat model of chronic SIADH showed that after 14 days of severe hyponatremia, body water content had returned to control levels ( Figure 44.3 ). Body sodium and chloride levels were reduced after one day of hyponatremia and were sustained for 14 days, and body potassium was significantly decreased after 7 days. Acutely, water retention was the major cause of hyponatremia, but solute depletion was primarily responsible when the electrolyte disturbance was sustained.

Urinary losses can directly lower the plasma sodium concentration when the concentration of sodium plus potassium in the urine is higher than the plasma sodium concentration. This can occur when high vasopressin levels (which concentrate the urine) and high rates of sodium and potassium excretion occur together. The excretion of hypertonic urine generates free water, in essence “desalinating” the plasma.
Serum Bicarbonate Concentration in SIADH
In SIADH, the serum sodium and chloride concentrations are lowered by dilution, but the serum bicarbonate concentration is typically normal. This finding has been explained by a direct effect of hyponatremia on the adrenal gland to increase aldosterone secretion, which then augments renal net acid excretion. Patients with hyponatremia due to hypopituitarism have many features in common with patients with non-endocrine SIADH, but their serum bicarbonate concentrations are about 5 mmol/l lower. Consistent with the hypothesis that hyponatremia-induced hyperaldosteronism is responsible for the normal serum bicarbonate in classic SIADH, aldosterone levels are much lower in patients with ACTH deficiency than in patients with non-endocrine SIADH.
Common Causes of SIADH
Tumors
The first cases of SIADH were described in patients with lung cancer. Small-cell carcinoma of the lung remains a common cause of the syndrome; approximately 10 to 15% of these patients present with SIADH, whereas fewer than 1% of patients with non-small-cell lung cancer become hyponatremic. Ectopic production of vasopressin appears to be responsible for most cases hyponatremia associated with small-cell carcinoma. Arginine vasopressin, oxytocin, and neurophysins have been found by radioimmunoassay in tumors, and are produced by the vast majority of small-cell lung cancers; the quantity of vasopressin peptide is closely correlated with the presence of hyponatremia. Atrial natriuretic peptide mRNA has also been detected in a high percentage of cell lines from patients with small-cell cancer. Hyponatremia develops in approximately 3 to 7% of patients with head and neck cancer ; the mechanism for SIADH associated with these tumors is unknown. Although a number of other tumors can produce vasopressin, there are very few reports of hyponatremia associated with them. SIADH may also emerge in patients with acute tumor lysis syndrome.
Pulmonary Disease
Mild hyponatremia has long been recognized in patients with tuberculosis. The mechanism for vasopressin release has not been determined, but hormone levels fall in response to water-loading, reflecting the reset osmostat variant of SIADH. Ectopic secretion of antidiuretic hormone was the presumptive cause of SIADH in a patient with central diabetes insipidus who developed pulmonary tuberculosis. Tuberculosis-associated hyponatremia resolves within days to weeks of antituberculous therapy. Plasma vasopressin levels are typically elevated on admission in patients with bacterial pneumonia, and fall rapidly during treatment. Hyponatremia is common, and usually self-corrects relatively rapidly after a few days. Abnormal vasopressin secretion in simple pneumonia is not attributable to hypovolemia, hypotension or abnormal PO 2 or PCO 2 . An antidiuretic decapeptide (“pneumadin”), which rapidly increases arginine vasopressin (AVP) levels, has been isolated from rat and human lung. Further study is needed to determine if the decapeptide mediates SIADH associated with pneumonia and other lung disorders. Acute respiratory failure (hypoxia and hypercarbia) and severe asthma are also associated with SIADH.
Endocrine Disease
Hyponatremia is present in up to 88% of patients with Addison’s disease, and in 28% of patients with isolated ACTH deficiency. In Addison’s disease, vasopressin is released in response to volume-depletion caused by mineralocorticoid deficiency, and altered hemodynamics caused by glucocorticoid deficiency. Glucocorticoids may also directly inhibit vasopressin release. Unlike Addison’s disease, impaired water excretion in hypopituitarism is not associated with hyperkalemia, and it does not respond to volume replacement with isotonic saline so that hyponatremia in this condition has all the features of SIADH. However, hyponatremia in patients with hypopituitarism is associated with a lower serum bicarbonate concentration than in patients with hyponatremia from other causes of SIADH (see section “Serum Bicarbonate Concentration in SIADH”). Impaired water excretion in hypopituitarism and in adrenalectomized mineralocorticoid replaced subjects is associated with elevated vasopressin levels; administration of a vasopressin V2 receptor-antagonist normalizes urinary water excretion in adrenalectomized mineralocorticoid replaced rats. Inhibitory glucocorticoid receptors have been identified in the magnocellular neurons which secrete vasopressin, and these receptors are markedly increased under hypoosmolar conditions. Hyponatremia with clinical features of SIADH has been described frequently in hypothyroidism; impaired water excretion can be corrected rapidly by the administration of thyroid hormone. However, it is unclear whether hemodynamic or intrarenal factors, rather than vasopressin, is responsible for impaired water excretion in this condition.
Non-Diuretic Drugs
Arginine vasopressin used therapeutically in the treatment of gastrointestinal bleeding or in the management of septic shock, and the vasopressin analog desmopressin acetate (DDAVP), a pure V2 receptor-agonist used to treat diabetes insipidus, enuresis, and von Willebrand disease, may cause hyponatremia. In addition, a growing number of drugs that are unrelated to vasopressin have been reported to cause hyponatremia. Most published reports involve thiazide and thiazide-like diuretics, chlorpropamide, carbamazepine, oxcarbantipsychotics, antidepressants, and nonsteroidal anti-inflammatory drugs. Vincristine and vinblastine increase vasopressin release by unknown mechanisms, and hyponatremia associated with these agents is dose related.
Several drugs associated with hyponatremia appear to increase the response of the collecting duct to circulating vasopressin. Chlorpropamide has been the most thoroughly studied. In addition to augmenting release of vasopressin from the neurohypophysis, chlorpropamide increases the number of vasopressin receptors on collecting tubule cells and inhibits renal medullary synthesis of prostaglandin E 2 , an agent which blunts the hydroosmotic effect of vasopressin by diminishing adenylate cyclase activity. Inhibition of PGE 2 permits increased cAMP formation, enhancing the effect of the hormone. Nonsteroidal anti-inflammatory agents increase the hydroosmotic effect of vasopressin by a similar mechanism. Hyponatremia attributable solely to nonsteroidals has been rarely reported, but these commonly used drugs may exacerbate other causes of hyponatremia.
Carbamazepine most commonly causes hyponatremia when it is given to subjects who habitually drink large volumes of water. Carbamazepine increases water permeability of the distal inner medulary collecting duct in the absence of vasopressin, an effect that is cAMP-dependent and negated by a vasopressin V2 receptor-antagonist, implying an action on the V2 receptor–protein G complex. Oxcarbazepine, which is enjoying increased use because of fewer drug interactions than carbamazepine, causes hyponatremia in 9% of patients with epiliepsy who are treated with the drug.
Hyponatremia caused by cyclophosphamide may also be due to enhanced vasopressin action, but the mechanism for this effect has not yet been elucidated. Cyclophosphamide’s antidiuretic effect is delayed, with a time-course which parallels excretion of active metabolites of the drug. Cyclophosphamide’s antidiuretic effect may be enhanced by indomethacin. There are many reports of SIADH associated with psychotropic drugs, including phenothiazines, monoamine oxidase inhibitors, and tricyclic antidepressants; causality has been most convincingly demonstrated with tricyclics. More recently, a large number of cases of SIADH have been reported in patients taking serotonin reuptake inhibitors (SSRIs). Prospective series in elderly patients have shown that 12 to 40% became hyponatremic within two weeks of starting therapy with paroxetine. All of these agents act centrally, and could conceivably affect vasopressin release directly. There is evidence that SSRIs may have a direct effect on water permeability of the inner medullary collecting duct, increasing AQP2 without changing vasopressin levels. The recreational drug, 3,4-methylenedioxymethamphetamine (“Ecstasy”) has been associated with severe, and sometimes fatal, acute hyponatremia. Ecstasy induces vasopressin secretion, and users of the drug who become hyponatremic typically manifest marked polydipsia. Many cases of SIADH associated with amiodarone have been reported.
Post-Operative SIADH
Vasopressin levels are elevated after operative procedures, and remain elevated for several days. Administration of hypotonic fluid during this period of antidiuresis causes acute hyponatremia, with potentially disastrous consequences.
Urinary cation loss has been shown to play an important role in the pathogenesis of hyponatremia in patients with post-operative SIADH. In the post-operative period, it is common for physicians to infuse several liters of isotonic or hypotonic saline solutions, exceeding the intended replacement of third-space and external losses, and actually causing extracellular fluid volume-expansion. A balance study in women undergoing uncomplicated gynecological surgery showed that sodium plus potassium concentrations in the urine peaked at 295±9 mEq/L, and remained hypertonic to plasma for the first 16 hours after induction of anesthesia ( Figure 44.4 ). Because of the action of vasopressin and the natriuretic response to saline-induced volume-expansion, electrolyte-free water was generated, lowering the plasma sodium concentration despite infusion of isotonic saline. The infused saline was, in effect, “desalinated.” A similar phenomenon occurs when patients with subarachnoid hemorrhage and “cerebral salt-wasting” are given large volumes of isotonic saline to protect cerebral perfusion.

Neurologic Disorders and Cerebral Salt-Wasting
An association between hyponatremia and intracranial disease has been recognized since the 1950s, and hyponatremia has been reported in a wide variety of systemic diseases involving the CNS, including systemic lupus erythematosus, Guillain-Barre syndrome, meningitis, encephalitis, brain tumors, and brain abscesses. Noting increased urinary sodium excretion in hyponatremic patients with neurologic disorders, Peters referred to the condition as “cerebral salt-wasting”. Once it was recognized that high rates of urinary sodium excretion could be caused by unregulated secretion of antidiuretic hormone, most investigators ascribed hyponatremia in brain disease to SIADH, a syndrome which has been associated a wide array of central nervous system disorders, consistent with the multiple anatomic pathways leading to vasopressin secretion by hypothalamic neurons ( Table 44.3 ).
Cerebral salt-wasting was generally a forgotten term until the early 1980s, when a more concerted effort was made to understand the pathogenesis of hyponatremia in patients with intracranial disease (especially subarachnoid hemorrhage). Reduced blood and plasma volume were found in most patients with intracranial disease who were presumed to have hyponatremia secondary to SIADH. Prospective studies of patients with subarachnoid hemorrhage given maintenance fluids documented negative sodium balance, decreasing plasma volume, and increasing BUN among patients who became hyponatremic within a week of presentation. A course consistent with cerebral salt-wasting was also observed in patients with head injury, brain metastases, and hydrocephalus.
Maintaining an adequate circulatory volume has important clinical implications, especially in subarachnoid hemorrhage, where volume depletion and fluid restriction have been reported to predispose to cerebral ischemia and infarction. This finding, and evidence that volume-expansion protects against cerebral ischemic events in subarachnoid hemorrhage, has led to a general acceptance of “hypertensive, hypervolemic, hemodilutional” therapy for the disorder. When such treatment is given, a high urine sodium concentration and hyponatremia are not reliable indicators of salt-wasting, because hyponatremia may be due to SIADH and the natriuresis may be a response to iatrogenic volume-expansion. In one study, a positive balance for sodium could be documented in most patients believed to have cerebral salt-wasting when calculations included all infusions from the time of first contact with medical or paramedical personnel. High levels of catecholamines which are often associated with brain injury decrease venous capacitance and raise blood pressure, potentially increasing “effective arterial blood volume,” and promoting a physiological natriuresis.
A valid diagnosis of cerebral salt-wasting requires proof of urinary sodium losses, despite reduced effective arterial blood volume. Attempts to establish a diagnosis of hypovolemia have included clinical impressions, central venous pressure, and measurements of plasma and blood volume; none of these can define hypovolemia definitively.
As in patients with SIADH, plasma vasopressin levels are increased and urine sodium concentrations are elevated, but the increased vasopressin secretion in cerebral salt-wasting has been attributed to volume-depletion caused by the primary salt-wasting. Investigation into the pathogenesis of cerebral salt-wasting has focused primarily on the relative roles of natriuretic hormones and vasopressin after subarachnoid hemorrhage. Atrial natriuretic peptide (ANP) and brain natriuretic peptide (BNP) are both derived from cardiac tissue, and have natriuretic and aldosterone-inhibiting properties. Although BNP has been localized to the hypothalamus, it is primarily of cardiac origin. Cardiac release of ANP and BNP is regulated in part by the CNS, and brain injury may induce their release from cardiac tissue. Plasma ANP levels generally correlate with the presence and severity of blood in the ventricles and increased intracranial pressure. In one study, however, plasma levels of BNP, but not ANP or vasopressin, were correlated with urinary sodium excretion, and in a rat model of cerebral salt-wasting, ANP levels decreased and BNP levels did not change. Jugular venous sampling in suspected cerebral salt-wasting did not support cerebral release of BNP. It is thus unclear whether elevated natriuretic peptide levels are responsible for natriuresis in subarachnoid hemorrhage, and other factors, such altered sympathetic tone, depressed aldosterone levels, ouabain-like compound, and endothelin may contribute. In fact, several studies have indicated that fludrocortisone may be effective in the treatment of cerebral salt-wasting.
Regardless of the pathogenesis of renal salt-wasting in patients with subarachnoid hemorrhage, hyponatremia appears to be caused by vasopressin release that is independent of volume-depletion, and is thus “inappropriate.” In a large prospective study of acute aneurysmal subarachnoid hemorrhage treated aggressively with isotonic saline (between 3 and 8 liters daily), hyponatremia developed in one-third of the patients within 4 to 6 days, despite positive fluid balance, increased blood volume, and suppressed plasma renin and aldosterone levels. Plasma vasopressin was measured at concentrations of 1 to 4 pg/ml, despite plasma osmolalities at which the hormone should have been undetectable. Although plasma ANP levels were also increased in most patients, they did not correlate with serum sodium concentration. Thus, it is likely that both salt loss and SIADH contribute in varying degrees to the pathogenesis of hyponatremia after subarachnoid hemorrhage.
The true incidence of cerebral salt-wasting among patients with subarachnoid hemorrhage is probably low, and a clear distinction between this entity and SIADH in neurosurgical patients may not be important, because in either case the most effective treatment is intravenous hypertonic saline.
Nephrogenic Syndrome of Inappropriate Antidiuresis
A gain-of-function mutation of the V2 receptor gene, named nephrogenic syndrome of antidiuresis (NSIAD), was first described in 2005. Patients with this X-linked disease present with clinical and laboratory evaluations typical of SIADH, with undetectable plasma vasopressin levels. Missense mutations substituting cysteine or leucine for arginine on codon 137 cause constitutive activation of the vasopressin receptor, analogous to loss-of-function mutations found in patients with X-linked nephrogenic diabetes insipidus. Infants with NSIAD are at high risk for hyponatremia, because their diet consists primarily of liquid with little solute. However, the disease can escape detection until adulthood, and it should be suspected in young patients with a clinical diagnosis of idiopathic SIADH, especially if there is unresponsiveness to vasopressin antagonists. Female heterozygotes with skewed X-inactivation may develop spontaneous hyponatremia or an abnormal water-loading test.
Adaptations to Hypotonic Hyponatremia
Organic Osmolytes and Cell Volume Regulation
The osmotic challenges faced by hyponatremic patients are analogous to those faced by invertebrate organisms when they are exposed to a hypotonic environment. Throughout nature, cells respond to water stress in a similar manner. Water crosses cell membranes in response to osmotic forces, equalizing the activities of intracellular and extracellular solute. Cell volume is determined by the amount and concentration of intracellular solute. Since at equilibrium intracellular and extracellular osmolalities are equal, the relationship between cell volume and extracellular osmolality can be described by the following equation:
Cell water ≈ Cell solute content Extracellular osmolality
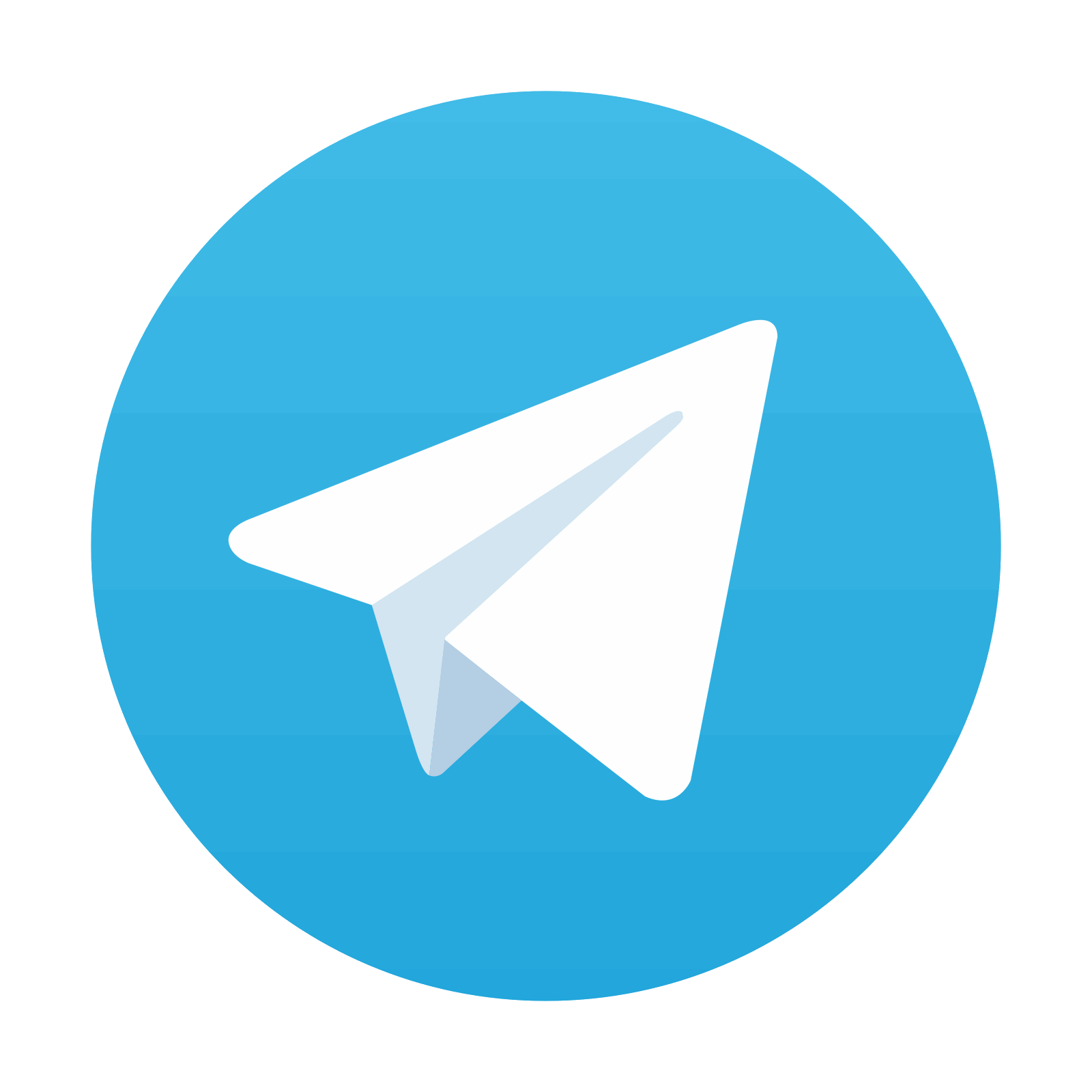
Stay updated, free articles. Join our Telegram channel

Full access? Get Clinical Tree
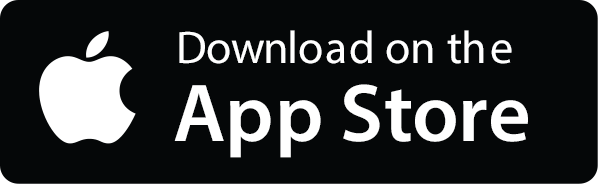
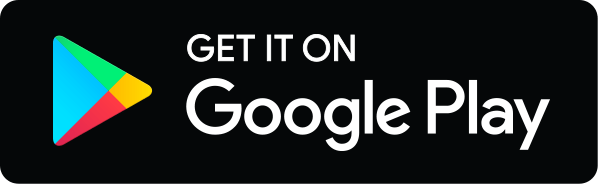