This chapter analyzes the capacity of the transplanted kidney to transport solute and water. The intrinsic capacity of the transplanted kidney to regulate glomerular filtration rate (GFR), to exhibit T-G feedback are described along with alterations that flow from retrieval ischemia and surgical engraftment. Water homeostasis with respect to osmotic diuresis, tubular transport and tubular responses to hormone are described. Pathologic alterations of tubular transport are described including renal tubular acidosis potassium handling mineral metabolite handling, handling of magnesium and urate. Renal transport altered by standard immunosuppressive drug utilization is then described with particular reference to cyclosporin A, tacrolimus, and sirolimus.
Keywords
T-G feedback, ischemia, RTA, cyclosporin A, tacrolimus, electrolyte handling
Introduction
The transplanted kidney is a healthy organ responding to a multiplicity of complex and variant clinical experiences and insults. There is no single model to adequately explain the manner in which solutes are handled by the transplanted kidney or the physiologic derangements that might be encountered. There is an ordered sequence of transplant events that can be isolated and provide the impetus for unique alterations in function that can be delineated. The hemodynamic state of the donor prior to harvesting can adversely affect early function of the graft. Perioperative circumstances, including the effects of warm and cold ischemia on the donor kidney, produce functional abnormalities similar to those intentionally produced by investigators examining ischemic acute renal failure. Intraoperative factors including hypotension, blood loss, and technical damage establish conditions capable of deranging the function of the transplant. Additionally, the transplanted kidney, both early and several months after engraftment, is a denervated organ with all the consequences to tubular function attendant upon that state. The use of newer immunosuppressive agents, which directly alter physiologic function of the kidney, also affects the performance of the new graft.
The placement of a functioning allograft in a setting in which ischemic effects have been minimized and engraftment has been uncomplicated may result in the passage of salt and water during the early post-transplant period. The nature and amount of salt and water are a function of volume and osmolar loads imposed by the azotemic state, which presents excess solute loads and stimuli for the elaboration of saluretic hormones. The effect of other comorbid disorders associated with uremia, such as secondary hyperparathyroidism, which may persist post-transplant, can alter the handling of such solutes as calcium, phosphorus, hydrogen ion, and bicarbonate even when excretory function is excellent.
The maintenance of a functioning allograft depends on forestalling immunologically mediated assault. For all but identical twins, transplant recipients are maintained on immunosuppressive medications, some of which may have an impact on salt and water regulation. For example, many recipients receive corticosteroids early in the transplant period regardless of the additional immunosuppressive medicines during the maintenance regimen. These drugs have profound effects on sodium, chloride, potassium, and water regulation. Other immunosuppressive agents including calcineurin inhibitors and rapamycin may alter renal transplant tubular function. Since the early 1980s, calcineurin inhibitors have been the mainstay of maintenance immunosuppression in the allograft recipient. Both cyclosporine and tacrolimus have the ability to profoundly alter renal blood flow and thus renal function through reduced glomerular filtration with resultant effects on the handling of sodium, potassium hydrogen and uric acid. Rapamycin, one of the newer agents to be added to the armamentarium may also lead to tubular dysfunction and altered solute transport. On this background, immunologic assault in the form of acute and chronic rejection can profoundly alter the handling of salt and water by the transplanted kidney, possibly related to effector cells or their released cytokines.
This review, updated from its previous version, first explores the intrinsic capacity of the transplanted kidney to modulate solute and water, and to maintain the internal milieu in homeostatic balance. By using the classic observations of Bricker et al. and more recent studies of tubular function that minimize warm ischemia and avoid the confounding variables of immunosuppressive drugs and rejection, the handling of solutes and water by the denervated transplanted organ will be characterized.
The remainder of this review deals with the various derangements of solute and water handling observed clinically after successful engraftment. These separate syndromes will be explained employing clinical data and observations in the human. When experimental data from transplant or related fields of research derived from animal models are applicable, they will be discussed and placed in proper context in an attempt to explain the etiology of or the physiologic basis that underlies the clinical syndromes.
The effect of immunosuppressive therapies on renal function and metabolic derangements has been extensively studied. This revised chapter makes a special effort to explicate the impact of these potent agents, particularly that of calcineurin inhibitors and rapamycin. Those experiments that reveal the multiplicity of effects of these agents in general are reviewed in an attempt to suggest a unifying theory as to their physiologic consequences.
Intrinsic Capacity of the Denervated Transplanted Isograft
To observe the intrinsic capacity of the transplanted kidney to maintain solute and water homeostasis, an experiment must be designed to minimize the effects of ischemia related to harvest, the solute load that the azotemic patient presents to the new kidney, the use of immunosuppressive medications, and the effects of rejection. These criteria were best met by the classic renal autotransplantation studies reported by Bricker et al. in the dog. These researchers left one kidney intact, excised the contralateral kidney, and retransplanted it into a discreet hemibladder. In this two-kidney autotransplantation model in nonuremic animals, renal hemodynamics, maximal concentration and dilution capacity, response to vasopressin, sodium, and potassium excretion, response to modulation of plasma volume by hypertonic and isotonic infusion, and the response to mercurial diuretics were studied. When renal hemodynamics had returned to normal after surgery in the transplanted kidney, the measured tubular functions were virtually equal in the just transplanted kidney to those observed in the contralateral, undisturbed kidney. Sodium excretion rates factored for respective solute loads were also equivalent to the contralateral, undisturbed kidney as was the ability to maximally concentrate or dilute the urine after several days. This study illustrates the ability of the denervated autotransplanted kidney to maintain normal solute and water balance.
In healthy individuals, head-out water immersion increases sodium excretion. Interestingly, in renal denervated dogs, the natriuretic response to head-out water immersion is completely abolished, suggesting a major role for the renal nervous system for this response. Yet, despite renal denervation, it has been shown that patients with well-functioning renal transplants of less than two months duration are capable of a normal natriuretic response to head-out water immersion. This observation suggests that renal nerve activity is not essential for a normal natriuretic response to head-out water immersion in renal transplant recipients. When six renal transplant recipients were compared to, six normal individuals subjected to head-out water immersion, it was demonstrated that the denervated, transplanted kidneys of the six recipients were able to maintain a sodium excretory response to head-out water immersion identical to that obtained in the normal controls. These studies, taken together with the earlier reports, illustrate that the denervated autotransplanted kidney is able to maintain normal solute and water balance.
More precise experimental observations about renal tubular function in the transplanted kidney were made in the studies of Muller-Suur et al. and Norlen et al. These investigators used a rat model in which recipient animals underwent bilateral nephrectomy just prior to isotransplantation. Using standard micropuncture techniques to assess tubular function, Muller-Suur et al. were able to show that five minutes after reestablishment of circulation to the donor kidney that had suffered the least amount of cold ischemia, there was no statistical difference in the single-nephron glomerular filtration rate (GFR) of the transplant compared to the untransplanted normal kidney (13.4 nl/min per 100 g of body weight vs 14.1). After an initial polyuric phase related to solute load, a phenomenon discussed later, the passage of sodium and potassium into the urine was equivalent in the transplant to that of the normal, untransplanted kidney. Norlen et al. measured proximal tubule reabsorptive capacity using micropuncture techniques in a similar experimental rat model. They demonstrated that there was normal proximal tubular fluid reabsorption (TF/P inulin ratio of 2.1 [± 0.4]) as well as normal whole-kidney function and normal single-nephron GFR. These studies show that bulk solute handling by the denervated, isotransplanted kidney is normal on a whole-kidney clearance basis. These nonrejecting grafts can maintain a relatively undisturbed total GFR, single-nephron GFR, and proximal tubular absorptive capacity as determined by micropuncture.
The functional integrity of the more distal nephron segments as well as the intrinsic capacity of the transplanted kidney to exhibit tubuloglomerular (T-G) feedback was investigated further by Muller-Suur et al. in the rat isotransplant model. Two experimental paradigms were employed. In the first, the isograft was punctured in the presence of the normal contralateral kidney; in the second, the puncture occurred in the solitary isograft after contralateral nephrectomy. In contrast to the observations by Norlen et al. the investigators found that the whole-kidney GFR of the transplanted kidney fell approximately 40% when the contralateral kidney was left in place. Yet when the remnant kidney was removed, the GFR in the isotransplant rose toward but not entirely to the two-kidney control value. These animals were then studied 15 hours after isotransplantation at a time when single-nephron GFR had risen essentially to normal in order to measure the effects of T-G feedback. There was normal urine flow rate, normal sodium and potassium excretion, a normal TF/P inulin, and a slightly reduced GFR. When unilateral nephrectomy was performed, urine flow and sodium/potassium excretion rates increased in both the transplanted kidney and in the control. Interestingly, T-G feedback was found to be intact, permitting appropriate modulation of GFR in response to solute load by the transplanted kidney when the contralateral, normal kidney was in place. An increase in osmotic and solute load that would occur after the contralateral kidney was removed would be expected to have produced a substantial reduction in GFR if no other compensatory mechanisms were at play. In the actual experimental situation, the single remaining transplanted kidney demonstrated a decreased sensitivity of the T-G feedback mechanism, measured as a shift in the T-G set-point ( Fig. 94.1 ). This attenuation of the feedback sensitivity set-point allowed the GFR to rise toward normal rather than fall in the transplanted single kidney to maintain normal clearance despite the increased solute load reaching the macula densa. Muller-Surr et al. advanced the hypothesis that increased interstitial pressure and volumes to which the transplanted kidney is exposed after contralateral nephrectomy explains resetting of T-G feedback. In summary, the isotransplanted kidney, when studied with a functioning renal remnant, retains its capacity to modulate GFR to solute load, but resets the T-G feedback set-point to maintain GFR in the face of increased bulk blood flow when placed in an environment devoid of additional functioning nephron mass represented by a normal remnant kidney.

Kidney transplantation between identical twins provides an interesting clinical counterpart to the rat and dog isograft studies. Bricker et al. analyzed the intrinsic capacity of the human kidney allograft to maintain solute and water homeostasis in the original set of identical twins transplanted at the then Peter Bent Brigham Hospital (now Brigham & Women’s Hospital) in Boston in 1954, an advance in clinical medicine recognized for the Nobel Prize of 1990. Recipients of an identical twin kidney allograft require no immunosuppressive therapy and rarely exhibit rejection. When compared to the remaining kidney function of the identical donor sibling, the transplanted organ was able to maintain an equivalent filtration fraction and showed a normal ability to concentrate and dilute urine as well as to acidify and to alkalinize the urine when challenged. Further, the allograft could increase and decrease the rate of sodium excretion in the face of load, to respond to various volume- and drug-related stimuli, and to retain the capacity to alter filtration fraction and renal blood flow acutely while maintaining extracellular fluid volume and composition. One can conclude from isograft studies in animals and from the observations in identical twin human allografts that the transplanted denervated kidney retains the capacity to modulate solute and maintain volume homeostasis.
Ischemia Attendant to Harvest, Preservation, and Engraftment
Experimental models and clinical observation demonstrate that the transplanted kidney has the intrinsic capacity to maintain solute and water homeostasis. Unfortunately, the allograft in clinical settings is exposed to injurious environmental pressures to which it must respond by modifications of physiologic function. This section of the chapter will attempt to isolate several of the insults to which the functioning allograft is exposed and reveal the means by which the transplant has successfully responded to these challenges or delineate the derangements that flow from unsuccessful responses.
Although every attempt is made to minimize the ischemic effect of kidney transplantation by paying scrupulous attention to donor management, to conditions of harvest, organ preservation, and to conditions of engraftment, some degree of ischemic injury is a uniform phenomenon. Even in the early studies of Bricker et al. of autotransplantation in the dog, an initial drop in GFR and renal plasma flow that normalized several days after surgery was observed. This initial drop in GFR was felt to be due to tissue ischemia induced by the operative procedure and did not have any lasting effects on kidney function as revealed by their measurements.
Muller-Suur et al. also noted important consequences of early ischemia in their Munich-Wistar rat isograft model. They observed that even in the group of animals that received the least amount of protocol-determined cold ischemia prior to implantation, a group able to maintain normal single-nephron GFR, there still were increases in urine flow rate with diminished urinary concentration (approximately 100 mOsm/kg) during the first three hours postoperatively, thought a consequence of surgical ischemic injury. During this initial polyuric phase, there was an additional increase in sodium and potassium excretion paralleling the enhanced urine flow rate. An initial polyuria and the formation of hypotonic urine, even when GFR is normal, were felt to be a universal consequence of the unavoidable ischemia of the transplantation event.
These findings have been confirmed in reperfusion studies in renal allografts in humans. Alejandro et al., noted that in the ensuing hours of post-transplant, fractional excretion of rates of sodium were elevated to >20% with an impairment of urinary concentrating ability even in those with normofiltration. Although significant tubular dysfunction occurred, by morphometric analysis, only ~2% of proximal tubular cells exhibited necrosis and obstruction of the tubular lumen. Unlike studies in the native kidney and supporting the work of others, this points away from an important contribution of tubular obstruction to postischemic injury in the transplanted allograft.
In turn, it has been suggested that afferent arteriolar constriction with an attendant fall in the glomerular perfusion pressure is the primary mechanism by which glomerular filtration is affected in the freshly transplanted kidney. One contributing mechanism may be that the high fraction of filtered sodium reaching the macula densa mediates afferent vasoconstriction via tubulo-glomerular feedback. In patients with persistent hypofiltration post-transplantation, loss of polarity of proximal tubular cells with redistribution of cytoskeletal proteins including Na + /K + -ATPase, fodrin and ankyrin from the basolateral membrane to the cytoplasm has been noted with subsequent impairment of proximal tubular sodium reabsorption.
In addition to tubuloglomerular feedback, vascular mediators are felt to contribute to a state of afferent arteriolar vasoconstriction. With sustained injury and delayed function of the transplant, both elevation in plasma renin activity and endothelin-1 levels have been observed. In addition, there appears to be resistance of afferent arterioles to the vasodilator actions of endogenous atrial natruiretic peptide, which is present in excess. Thus, postischemic allograft injury appears to be accompanied by an imbalance that favors constrictor hormones over those that dilate the afferent arteriole with a resultant depression in GFR by lowering of glomerular perfusion pressure.
Urinary exosomes released into the urine by fusion of the outer membrane of multivesicular bodies with the apical plasma membrane of renal epithelial cells are shown to include membrane and cytosolic proteins, which have the characteristics of all renal tubule epithelial cells, podocytes, and transitional epithelial from the urinary collecting system. Importantly, several of these proteins found in urinary exosomes have been implicated in various kidney diseases and suggest that the examination of urinary endosomes may allow for a non-invasive measurement of site-specific biomarkers.
Several studies have demonstrated changes in excretion of urinary exosomal Na + /H + exchanger isoform 3 (NHE3) and fetuin-A after renal ischemia/reperfusion. NHE3, a sodium transporter protein in the proximal tubule, was present in the urine within 24 hours after renal allograft transplantation with levels returning to undetectable 48 hours after transplantation relating to a marked improvement in renal function. Conversely, NHE3 levels were not increased in the urine in the setting of acute allograft rejection. This data suggests that NHE3 may be a temporal marker of renal ischemia/reperfusion injury. Urinary exosomal fetuin-A levels have been shown to be increased after ischemia/reperfusion in allograft recipients but also in donor patients suggesting this may be a response to a change in renal hemodynamics and not specific to renal ischemia/perfusion injury.
The excretion of urinary exosomal aquaporin-1 has been studied both in animal models and in human allograft recipients. Aquaporin-1 is a water channel protein abundantly expressed in renal epithelial cells of the proximal tubules and the descending limb. It is known that renal ischemia/reperfusion in the native kidney leading to acute kidney injury is associated with decreased renal aquaporin-1 expression with subsequent data showing a decreased abundance of urinary exosomal aquaporin-1 in Sprague-Dawley rats subjected to unilateral renal ischemia/reperfusion as well as a renal transplant recipient at two and six days after renal transplantation despite a dramatic decrease in the plasma creatinine concentration following the operation. There was no decrease in urinary exosomal aquaporin-1 excretion in both animal models of nephrotic syndrome and patients with proteinuria. These data suggest that urinary exosomal aquaporin-1 may be a useful urinary biomarker to predict posttransplant acute kidney injury related to ischemia/reperfusion injury. Follow-up studies in larger sets of patients will be needed to confirm these findings and validate the utility of the role of urinary exosomal proteins in the detection of renal/ischemia reperfusion injury.
Norlen et al. used microsphere injection techniques to evaluate alterations in intrarenal blood flow during varying lengths of warm and cold ischemia of the renal transplant. Analysis of cross sections of rat kidney showed that microspheres injected during the reperfusion phase after two hours of cold ischemia showed no areas of preferential blood flow. Longer degrees of ischemia (12–16 hours) resulted in poor filling of the medulla. Except for the polyuric phase, which always seems to accompany such ischemia, there was no functional or structural abnormality after two hours of cold ischemia. On the other hand, after 12–16 hours of cold ischemia, there were dilated and collapsed tubules interspersed with morphologically “normal” tubules. The dilated tubules exhibited no glomerular filtration and were thought a consequence of obstruction by swollen epithelial cells in the corticomedullary region. This study provides anatomic correlates to the ischemic engraftment and preservation procedures.
The different studies in animals taken together show that short-term ischemia may derange volume homeostasis and concentration ability. Longer periods of ischemia may result in delayed reperfusion of the corticomedullary region, possibly due to cellular swelling in that area. The effect of the more prolonged ischemia to the transplant is a fall in GFR and an early loss of concentrating ability of the tubule. These alterations are potentially reversible spontaneously and may lead to no long-term sequelae. Clinically, the absence of urine formation after surgery suggests longer ischemic injury, whereas some element of the universally encountered polyuria is a consequence of shorter periods of ischemia.
Most data, however, would suggest that early ischemia/reperfusion injury of short duration does not lead to untoward long-term consequence to the allograft. Prolonged injury on the other hand, may contribute to late renal allograft deterioration and failure. To evaluate the association between initial ischemia/reperfusion injury occurring secondary to organ retrieval, storage, and transplantation and late renal allograft deterioration and failure, Azuma et al. studied the patterns of proteinuria, cellular infiltration, cytokine expression, and glomerular sclerosis over time in 344 Lewis and Fischer rats after 45 minutes of warm ischemia of a singe kidney. Intracellular adhesion molecule I, endothelin, and major histocompatibility complex (MHC) class II expression were found to be upregulated within two to five days after the injury, which enhances the antigen load presented to the recipient and the appropriate signal pathways to enhance antigen recognition, culminating in a drive for additional acute and later chronic rejection. In the animals, proteinuria developed after 8 weeks, and glomerulosclerosis, arterial obliteration, and interstitial fibrosis occurred after 16 weeks. These data suggest that early ischemia and reperfusion, if severe enough, may not only contribute to early dysfunction but also to late renal deterioration and chronic rejection. Further studies are needed for better understanding of the factors mediating these abnormalities.
Allograft in the Azotemic Solute-Loaded Recipient
Clinical observations teach that the placement of a functioning allograft into an azotemic patient leads to copious urine formation. The typical diuretic phase postoperatively has been attributed to several potential etiologies. As has been previously discussed, ischemia per se affects the concentrating ability of the nephron. Solutes that accumulate in azotemic patients, even in the face of adequate dialysis, can induce an osmotic diuresis when GFR is adequate. Additionally, the administration of loop diuretics such as furosemide and osmotic diuretics such as mannitol during the transplant operation will contribute to this polyuric phase. Lastly, the etiologic role of atrial natriuretic peptide (ANP) as the basis for some of this polyuric phase has been suggested by observations in humans and studies on animals using a volume-overloaded ischemic kidney model and measurements of that peptide in early clinical transplant experience.
During the immediate postoperative period, the diuresis that frequently occurs can be profound and, if not followed assiduously with appropriate replacement therapy, can be life threatening. In their study of six patients after native nephrectomy and transplantation, Ogden et al. observed diuresis of 5–14 liters over the first 24 hours after transplantation. The diuresis was osmotic, with the osmolar clearance of electrolytes accounting for more than 50% of the total solute clearance. Although these patients were relatively hyperosmolar due to retained blood urea nitrogen (BUN), their osmotic diuresis post-transplant was not primarily driven by urea but by electrolytes, principally sodium. Swenson et al. studied sodium balance in four patients who had undergone kidney isotransplantation and found negative sodium balance of 150–1000 mEq during the immediate postoperative period. They found that the rate of sodium loss correlated with pretransplant overexpansion of extracellular space (ECS) and that water excretion was more a function of sodium than urea excretion. Additionally, they observed hypovolemia related to this osmotic diuresis in several patients with postural hypotension and tachycardia when urine output was inadequately replaced. These data suggest that the osmotic diuresis may be a result of an inability to retain filtered sodium when required and not merely of the presence of increased filtered loads.
As was discussed in the previous section, the ischemic insult of transplantation alters the ability of the tubule to concentrate urine, in particular the proximal tubule. This occurs even in the optimal setting of live donor transplantation whereby ischemia is minimized. In a study of eight renal allograft recipients of live donor kidneys, Bugge et al. noted a high fractional excretion of sodium during the first hour after surgery correlating with a high urinary excretion of N-acetyl-ß-glucosaminidase. NAG is a lysosomal enzyme found in high concentrations in renal tubules, especially in the S3 segment of the proximal tubule. The data taken together suggest that it is the defect in proximal tubular transport contributing to the post-transplant osmotic diuresis that hitherto was thought to be a consequence of solute loads presented to disordered cortical collecting tubules.
Diuretics, including both osmotic and loop, given during the perioperative period also contribute to the post-transplant polyuria. Mannitol has been shown to reduce the frequency of postsurgical acute renal failure after renal transplantation. It has been suggested that the combination of mannitol and volume expansion gives better protection than volume expansion alone, but the mechanism for this protective effect is not known. By its osmotic activity, mannitol increases plasma volume expansion, decreases systemic and renal vascular resistance, and counteracts swelling of tubular cells. All of these properties of mannitol may contribute to its protective effect against ischemic renal injury. Loop diuretics reduce sodium reabsorption and oxygen consumption in the thick ascending limb of Henle, and simultaneously, increase the oxygen availability for the S3 segment of the proximal tubule that may protect against ischemic injury.
One might infer that the correct clinical response to the expansion component of the diuresis would be to restrict perioperative volume loads. In fact, such inference is absolutely false, as it has been shown that challenges of hypervolemia during renal transplantation decrease the post-transplant period of dysfunction due to postsurgical acute renal failure. It has long been known that salt loading prior to ischemia or nephrotoxic insults delimits the physiologic display of injury; the transplant condition is not different in this regard. Some have hypothesized that the protective effect of volume expansion may be due to released atrial natriuretic factor (ANF). One argument is that the intrinsic hypervolemia of the transplant recipient at the time of surgery and the additional volume loads imposed by the transplant team generate high levels of ANF, which protect the nephron from ischemic injury, an unavoidable consequence of the surgical event. Measurements of pulmonary artery pressure (PAP), pulmonary wedge pressure (PWP), and central venous pressure (CVP) as an estimate of systemic volemia in seventeen patients during allograft placement were compared with levels of ANF. Although it was difficult to statistically relate measures of these parameters of volume status to markedly elevated levels of ANF, there was a clear positive correlation between the changes in ANF and acute changes in central volemia ( Fig. 94.2 ). This study suggests that during transplantation ANF is elevated at the time of surgery and its release is facilitated by therapeutic volume challenges. Additional data by Nyberg et al. and Plum et al. lend further support to this view. In a study of 14 patients undergoing live donor kidney transplantation, Nyberg et al. showed that ANF levels rose significantly after transplantation and volume expansion and by stepwise multiple linear regression analysis was the strongest determinant of post-transplant diuresis. Similar findings were also reported by Plum et al. in the early post-transplant period.

The protective role of ANF on the ischemic injury of transplantation has been suggested by animal models. By using an ischemic renal injury rat model, Gianello et al. investigated whether the atrial natruiretic peptide by itself was able to improve early renal function after an ischemic injury. In a group of Wistar rats subjected to right nephrectomy and left renal artery occlusion for 30 minutes followed by a two hour infusion of rat atrial natruiretic peptides, there was a significant improvement in early recovery of renal function as compared to those rats who received isotonic saline alone. There was a notable increase in urinary flow rate, sodium excretion and improvement In GFR recovery. A similar experiment confirmed and extended these observations in a dog model showing protective ANF effects with low doses of human exogenous ANF. It is worth noting in both animal models, a striking increase in GFR after ANF infusion. In the intact kidney, the enhanced GFR induced by ANF results from a hemodynamic effect as noted by vasodilation of the preglomerular afferent arteriole and constriction of the efferent artery. It has been hypothesized that a similar mechanism of action of ANF is involved in the ischemically injured kidney model.
In addition to the glomerular effects, ANF also considerably improves natriureisis and fractional sodium reabsorption rates suggesting that ANF may also have direct tubular effects and may be direct or mediated by an inhibition of the renin-angiotensin-aldosterone system.
These experiments in animals show that volume expansion and the resultant diuresis is protective of recovery after ischemic renal injury. This conclusion is corroborated by studies in renal transplant recipients who have improved graft function after perioperative volume expansion. The onset of a rapid osmotic diuresis after transplantation heralds a rapid recovery and all attempts should be made to augment the early and copious formation of urine through volume expansion and diuretics. There is evidence to suggest that the protective nature of volume expansion leading to early renal recovery from surgical injury may be partially related to ANF release.
In addition to hypervolemia-induced release of ANF, there is evidence that glucocorticoids, a standard concomitant of clinical transplantation, directly stimulate ANF release and by doing so provide further protection to the renal transplant against ischemic injury. Eight healthy men were studied by Weidmann et al. in a cross-over, “blinded” study of the effect of prednisone (50 mg/day for nine days) on ANF while on a diet containing 130 mmol of sodium and 75 mmol of potassium. During the prednisone therapy period, there was no change in sodium balance, blood pressure, or weight compared with the placebo period. Over the nine-day study period, the mean supine ANF was nearly threefold greater during prednisone intake than during placebo intake in controls. Confirmatory studies in Sprague-Dawley rats by Gardner et al. showed that rats injected with dexamethasone, 1 mg/day, for one to two days while water deprived, exhibited a twofold increase in ANF. These animals were then killed and slot-blot analysis of atrial cells by using a cDNA probe for ANF showed 1.5-fold to two-fold increase in mRNA for ANF in those animals treated with steroids. These findings suggest that glucocorticoids directly stimulate ANF synthesis by atrial cells. Although not directly studied in transplantation, the early use of glucocorticoids in that setting may enhance the release of ANF in the early post-transplant period, play some part in the observed initial polyuria, and serve as a protective therapeutic maneuver against perioperative ischemia.
Although it has been shown in animal models of acute ischemic renal failure that ANF ameliorates renal damage, administration of synthetic ANF in humans following renal transplantation in attempts to promote initial graft function have not shown any beneficial effects. In a prospective, double-blind study by Sands et al. 10 pairs of cadaveric kidneys were transplanted into 20 recipients. Upon revascularization of the allograft, paired recipients received either humanized ANF or placebo administered intravenously as a 50- µg bolus, followed by a 4-hour infusion at 0.1 µg/kg/min. Renal allograft function was determined both by serum creatinine and measurements of GFR by (125I)iothalamate. No significant differences were noted in renal allograft function between the two groups. Similar results have been obtained by others. These results do not mean that ANF plays no role in preserving renal function after transplantation as extracellular volume expansion is a major stimulus for endogenous ANF secretion. It suggests that hANF alone is not superior to conventional extracellular volume expansion.
The early diuretic phase after transplantation is important for the long-term outcome of the graft. Although there is suggestion that the early diuretic phase is related to impairment of function in the concentrating segment and proximal tubule, the impairment is transient, with no long-term sequelae. The osmotic load provided by the azotemic state and the perioperative volume expansion with resultant additional solute loads presented to the kidney, all contribute to diuresis directly and further stimulate ANF, which is responsible for an additional component of urine formation. Every attempt should be made to facilitate post-transplant diuresis, which improves immediate graft function, enhances the rapid recovery of the transplant recipient, helps to make the early management period less difficult for the transplant team, and culminates in a substantial increase in graft survival.
Solute Regulation During Rejection
The most unique syndrome that a transplanted kidney must deal with is that of acute rejection. Derangements of renal function, which characterize this immune assault on the allograft, include not only a decrease in the GFR but also altered solute excretion. The diminution of sodium concentration in the urine has even been used by some as a useful clue to the diagnosis of acute rejection. Renal retention of sodium reflects intrarenal ischemia, the final result of the immunologic assault on the transplanted allograft. In general terms, renal inflammation with organ swelling and edema can extrinsically reduce renal blood flow. More precise mechanisms responsible for physiologic dysfunction during rejection are doubtless at play and are worthy of a great deal of study. Acute cellular rejection of the kidney is associated with the release of monokines from activated T lymphocytes and from monocytes, which are in great measure responsible for the changes one calls inflammation. One important cytokine released is interleukin-2 (IL-2). Although not formally studied in kidney transplantation, patients who are receiving supraphysiologic doses of IL-2 for immunotherapy of metastatic melanoma or renal cell carcinoma exhibit functional changes in solute handling similar to those encountered during acute rejection. IL-2 regimens result in systemic hypotension due to systemic vasodilatation associated with azotemia and low urinary excretion rates of sodium. Although the amount of systemic IL-2 released during rejection is insufficient to cause systemic hypotension, the local release of the cytokine into the renal circulation may be sufficient to vasodilate renal beds directly or indirectly, open endothelial tight junctions, and contribute to the edema and high intrarenal pressures thought to be responsible for extrinsic reduction in total renal blood flow and sodium avidity.
Another cytokine, tumor necrosis factor (TNF), is found to be elevated during acute rejection episodes. In a group of prospectively monitored transplant recipients, TNF elevation was diagnostic of rejection in half of the cases. Even when not diagnostic of acute allograft rejection alone, elevated TNF measured during rejection amplifies the importance of cytokines during the immunologic assault. The effects of human TNF on glomerular histology in rabbits given doses of 0.08, 0.8, and 80 g/kg/liter for five hours were studied by Bertani et al. At the 0.8g/kg/liter dose administered, there was leukocyte infiltration of glomerular capillaries as well as inflammatory cells within the glomerular capillaries. At the dose of 8 g/kg/hr, there was renal failure accompanied by the leukocyte infiltration with fibrin accumulation. The effects of TNF on renal vascular resistance released during acute rejection may mediate the observed endothelial damage.
Inflammatory prostanoids of the vasoconstrictive variety released from activated monocytes infiltrating the allograft have been implicated in the reduction of renal blood flow and impaired excretory function of rejection. The role of arachidonic acid metabolites in the transplant physiologic derangement during acute rejection has been studied by Coffman et al. using a rat allograft model between inbred rat strains (Lewis rat donors to Brown-Norway recipients). Associated with the histologic evidence of cellular rejection based on interstitial and perivascular infiltrates of mononuclear cells and the reduction of inulin clearance rates, there was an increase in the production of thromboxane B2 (TXB2) without a correlative increase in prostaglandin E2 (PGE2) or 6-ketoPGF1α (the stable metabolite of prostacyclin [PGI2]) ( Fig. 94.3 ). Attempts at reversal of the cellular rejection by using cyclophosphamide resulted in improvements in the clearance of inulin as well as in a reduction in the urinary levels of TXB2. Additionally, the use of a thromboxane synthetase inhibitor resulted in an improvement in GFR and renal blood flow during acute cellular rejection episodes when administered before an immune assault on the organ. The fact that a thromboxane synthetase inhibitor did not completely ameliorate the detrimental effects of the acute cellular rejection suggests that other factors also may be at play during rejection. In another study by Munger et al. kidneys were transplanted from Munich Wistar (MW) rats to syngeneic controls and MHC-incompatible recipients to evaluate the effects of surgery and acute rejection on glomerular hemodynamics by clearance and micropuncture techniques. A significant decrease in both single-nephron GFR and glomerular capillary pressure was found in animals with acute rejection. Micropuncture studies showed that the principal derangement in renal microvascular function during acute rejection is intense preglomerular vasoconstriction. Interestingly, the glomerular histology was normal, suggesting that locally acting vasoconstrictor influences such as TXA2 and leukotrienes were most likely responsible for the depressed renal function.

A recent study by Velic et al. examined the effect of transplant rejection on the functional activity and regulation of transporters involved in sodium reabsorption in the proximal tubule using a rat allograft model of acute rejection without immunosuppression. In those kidneys with histologic evidence of acute rejection, downregulation of mRNA and protein levels for Na+/H+ exchanger type-3 (NHE-3) were noted, independent of ischemia.
In follow-up, the same group extended their experiments to the distal nephron to further characterize the role of rejection on expression and function of transporters and receptors. In the cortical collecting duct, mRNA expression and function of the epithelial Na + -channel (ENaC) and mRNA and protein expression of the water-channel AQP2 were downregulated. To differentiate between possible effects of acute rejection and those directly related with the transplant process such as denervation or ischemia/reperfusion, syngeneic transplantations were performed. Whole kidney mRNA and protein expression of ENaC and AQP2 remain unchanged. Addition of cyclosporine abolished the downregulation of both channels, again supporting the notion that these changes are the result of acute rejection. Both studies show that in models of acute rejection, transporters important in Na + reabsorption and consecutively solute and volume reabsorption may lead to a significant decrease in energy consumption and represent a period of adaptation by the kidney to support recovery of the stressed organ and protect it from further non-immunologic and immunologic damages.
These studies taken together suggest that changes in expression of proteins involved in tubular transport may be subjected to rejection-associated mechanisms. This is a fertile area for future research and may provide therapeutic targets for the accelerated recovery of acute rejection of the allograft, and therefore, the prognosis of the renal transplant.
In summary, true rejection is a state of low GFR, intrarenal vasoconstriction, and sodium avidity related to the immune activity within the graft that often persists beyond the abrogation of the cellular infiltration or humoral assault on the tissue. This may reflect the consequences of inflammatory mediators released by various infiltrating cells and adaptive tubular regulation of solute transport. This is a fertile area for future research and may provide therapeutic targets for the accelerated recovery of acute rejection of the allograft, and therefore, the prognosis of the renal transplant.
Renal Tubular Acidosis Associated With Ischemia and Rejection
Renal tubular acidosis (RTA) is the most commonly encountered group of tubular abnormalities found after successful renal transplantation and may cause profound changes in acid base balance. One can describe various types of renal tubular acidosis including proximal RTA, Fanconi syndrome, distal RTA and Type IV RTA with hyporeninemic hyperaldosteronism, some of which may overlap and vary as a function of time during the lifespan of the allograft. For example, proximal RTA and Fanconi syndrome have been noted to occur early in the post-transplant period and resolve spontaneously suggesting that this may be in part attributable to acute ischemic renal failure related to the harvest procedure. Other types of renal tubular acidosis, particularly distal RTA may appear months to years after the transplant and are due to chronic rejection or drug-induced nephrotoxicity, including calcineurin inhibitors. Secondary hyperparathyroidism, obstructive uropathy and urinary tract infections may also contribute to the pathogenesis of RTA. Chronic RTA following transplantation is important as it may interfere with bone metabolism and at times lead to nephrocalcinosis and nephrolithiasis.
The presence of hyperchloremic, nonanion gap acidosis in the transplant patient was first described by Massry et al. in 1967. In their case, hyperchloremic acidosis appeared on day 33 after successful transplantation when GFR was improving and the serum potassium was 3.3 mEq/liter, chloride was 1215 mEq/liter, and plasma bicarbonate was 15 mEq/liter. Additionally, this patient had glycosuria, aminoaciduria, and hyperphosphaturia along with the metabolic acidosis, suggesting a complete Fanconi syndrome due to proximal tubular dysfunction. The clinical picture was best defined as a dual effect located in the distal and the proximal tubule, in part because of the failure to acidify the urine, even with administration of exogenous acid coupled to the Fanconi syndrome. Better additionally showed that when excess buffer base was provided to the distal nephron, this patient was unable to generate a steep hydrogen ion gradient, indicating that there was a distal tubular defect in transporting hydrogen ions either into the lumen from the tubular cell or from the blood into the lumen. In the report of Rubini et al. from 1967, an early post-transplant tubular dysfunction similar to the Fanconi syndrome with spontaneous resolution was observed. Rubini et al. also suggested that distal tubular dysfunction was found along with the Fanconi syndrome, arguing that the form of RTA observed in the early post-transplant period is a mixed disorder of proximal and distal tubular dysfunction perhaps the result of ischemic injury encountered during the harvest and engraftment procedure.
The impact of high circulating levels of parathyroid hormone (PTH) of the recipient on proximal tubular acidification may contribute to the early post-transplant RTA as shown by studies of Arruda et al. in dogs in which PTH was infused acutely. Animals receiving a human PTH infusion of 1 U/min or a bolus of 100 U followed by a continuous infusion of 5 U/min exhibited an increase in bicarbonate excretion and an increase in urinary pH. The increase in urine pH was associated with an increase in total titratable acid due to an increase in proximal tubular rejection of phosphate. The next acid excretion remained unchanged during the bicarbonaturia due to a decrease in ammonia excretion. The urine–blood (U-B) PCO2 ratio remained stable during the study period, suggesting the distal tubular ability to secrete H + was not altered by the PTH infusion.
Purer forms of distal tubular acidosis are the types of acid–base disorders most likely to persist after transplant and can become a clinical problem requiring intervention. In a study by Better et al. six patients not receiving cyclosporine were shown to have urinary acidification defects after successful cadaveric transplant. All these patients showed an inability to create a steep gradient for hydrogen ion exchange in the distal tubule. Follow-up studies done over the next 15 months showed that each patient was able to acidify the urine and excrete titratable acid, in spite of the development of chronic transplant rejection in two patients. Indeed, Better et al. concluded that neither rejection nor the immunosuppressive medications used at the time of their study played an important role in the urinary acidification defect that they were observing in transplant recipients. They argued that the defect observed was truly the result of ischemic injury attendant on the transplantation event and organ retrieval, which slowly resolves over time.
The natural history of RTA after renal transplantation has been best described in studies by Wilson and Siddiqui in 32 patients prospectively studied for one to three years with repetitive urinary acidification testing. The authors argued that there are two clinical pictures of RTA after renal transplantation. Fourteen of the 32 patients initially had a distal RTA with an inability to reduce the urine pH below 5.3 in the face of systemic acidosis. Ten other patients initially had a systemic, hyperchloremic acidosis with depressed serum bicarbonate levels but with a normal ability to acidify the urine in the face of the acidosis. In this latter group, two patients had clearly demonstrable proximal tubular bicarbonate wasting. The presumed proximal bicarbonate wastage and the hyperchloremic acidosis disappeared or improved with time in seven of the ten patients. Partial distal defects of hydrogen ion transport with a persistent acidification defect remained in nine of the 14 patients with distal lesions. They concluded that initially after surgery, a wide variety of tubular transport defects may be found from pure forms of proximal RTA, sometimes with a complete Fanconi syndrome, to distal RTA, although a mixed defect was most commonly encountered. With a resumption of normal organ function, there is a fairly rapid recovery of the proximal tubular acidification capacity. In most patients, a distal acidification defect remains for a greater length of time with ultimate resolution in most. Those with persistent distal lesions become a management problem with respect to potassium homeostasis and healing of the metabolic bone disease of renal failure, which requires the careful attention of the astute clinician.
A second group of patients will develop new defects in renal tubular acidification because of the immunologic complications of rejection. Wilson and Siddiqui also described the new appearance of a distal tubular acidification defect in patients who had no previous abnormalities of acid–base tubular homeostasis, which they suspected was due to superimposed renal rejection. Batlle et al. directly studied the role of chronic rejection on hyperchloremic metabolic acidosis. In five of six carefully studied patients with chronic rejection, urine pH could not be acidified below 5.5, and the U-B PCO2 difference was not increased after a sodium sulfate buffer base infusion. A tubular defect of distal hydrogen ion secretion was found to account for this inability to acidify the urine. These investigators emphasized the development of interstitial infiltrates by round cells characteristic of chronic rejection in five of these six patients, who ultimately lost renal function. A similar pathologic picture, with the infiltration of round cells into the parenchyma associated with the development of RTA, is seen in other states of immune activation and renal infiltration by immune cells such as in Sjögren syndrome. The distal RTA of Sjögren syndrome may result from an absence of the H + -ATPase pump, a key enzyme in distal urinary acidification by the intercalated cells of collecting ducts, and it has been hypothesized that this deficiency may also account for the RTA of kidney transplant rejection. Jordan et al. studied six kidney transplant patients with biopsy proven rejection and two control patients physiologically and by immunohistochemistry. Impaired ammonium excretion as well as impaired proton secretion with reduction in urinary PCO 2 was noted in those patients with rejection. In none of the patients was there a total absence of intercalated cell H + -ATPase staining as seen in Sjögren syndrome, but there was an inverse relationship between the abundance of H + -ATPase staining and degree of scarring. A direct relationship was noted in the abundance of H + -ATPase-stained intercalated cells and 100/plasma creatinine. It may be that the tubulitis of rejection not only affects H + -ATPase-mediated acidification by individual intercalated cells but also, via disruption of tubular anatomy, reduces the transepithelial voltage leading to defective ammonium excretion and a distal RTA. The development of secondary distal RTA may be an early sign of the immunologic process that leads to rejection.
In summary, renal tubular acidoses that affect all portions of the nephron can be observed after successful renal transplantation. Proximal tubular defects including the complete Fanconi syndrome are found most often in the perioperative period. The proximal tubular abnormality resolves as the transplant function improves. Distal tubular defects of acidification are encountered both early and late after successful renal transplantation and are the most common lesions encountered. These lesions can persist throughout the functional life of the kidney, although they usually recede at a slower rate than the early proximal RTA. These defects have been described in patients before the introduction of calcineurin inhibitors to the immunosuppressive protocol. The nature of RTA in the transplant patient will be revisited in our discussion of the impact of immunosuppressive medications on renal function. Ischemic injury related to organ retrieval and placement has been described as the cause for the abnormalities seen early after transplantation. A second, spontaneously appearing distal RTA is seen in patients whose graft is undergoing rejection. The development of distal RTA may be an early sign that a transplant is having an immunologic insult.
Syndromes of Potassium Handling
After successful renal transplantation, potassium transport was generally normal in the precyclosporine era. Postoperatively, if one did encounter a problem with potassium homeostasis, hypokalemia was frequently observed. The most common cause for this hypokalemia is polyuria and inadequate replacement during the diuretic phase, as was discussed earlier in this chapter. A large osmotic diuresis drives potassium into the urine and limits the tubular capacity to modulate potassium. In addition, a distal RTA and the use of relatively high doses of steroids early in the postsurgical period may contribute to the hypokalemia. These circumstances aside, persistent hypokalemia has been found to be uncommon after the immediate postoperative period.
Hyperkalemia also has been described after renal transplantation in the absence of impaired filtration, significant acidosis, potassium supplementation, or calcineurin inhibitor use. DeFronzo et al. observed clinical hyperkalemia in 23 of 75 patients in the first three months following successful transplantation. Four patients of this group were studied in detail to ascertain the mechanism for their hyperkalemia. As would be expected after a successful renal transplant, these patients responded appropriately to volume and sodium challenges with respect to the renin-angiotensin-aldosterone axis. Increased distal delivery of solute achieved by a loop diuretic, by a carbonic anhydrase inhibitor, or by bicarbonate infusion did not enhance potassium excretion, suggesting that a distal sodium-potassium exchanger was defective. Therapeutic challenge with 9-fluorohydrocortisone also failed to reduce serum potassium. The failure to enhance potassium excretion by increasing the solute delivery and the impaired mineralocorticoid response pointed to impaired distal tubular potassium transport as the etiology for the observed hyperkalemia. Thiazide diuretics were able to diminish the serum potassium level and enhance potassium excretion, suggesting that this defect was not structural. Indeed, studies of these patients showed that hyperkalemia disappeared with time, further suggesting that the distal tubular defects of potassium transport may resolve as transplant function improves.
The presence of the syndrome of pseudohypoaldosteronism originally described by DeFronzo et al. remains controversial, with confirmation by some and rejection by others. The quoted rate of occurrence of 30% has been most vigorously challenged. In one supporting study of a 56-year-old woman with hyperkalemia, Uribarri et al. found diminished kaliuresis and a marked kaliuretic response to 9-fluorohydrocortisone after successful renal transplantation. Other clinicians pointed to hypoaldosteronism in patients related to adrenal failure and renal transplantation. In two such patients, low plasma renin and aldosterone levels in the salt-restricted, volume-contracted, upright posture were absent. When challenged with 9-fluorohydrocortisone, these patients had a normal kaliuretic response. Several other reports of transient hypoaldosteronism supported this initial observation by Perez and Oster and Rosenbaum et al. These studies suggested that after transplantation, impaired secretion of potassium from the distal tubule may appear to be due either to direct tubular dysfunction or to mineralocorticoid deficiency. This pseudohypoaldosteronism is observed early in the post-transplant period and resolves spontaneously. The functional defect can be corrected with thiazide diuretics. The hyperkalemia of true hypoaldosteronism also can appear after transplantation and responds to replacement therapy.
It is important to emphasize that although potassium-handling defects were interesting before the use of calcineurin inhibitors, their clinical significance was trivial. With the introduction of cyclosporine and tacrolimus into the immunosuppressive armamentarium, the problem of hyperkalemia has become more prominent. A complete discussion of the effect of calcineurin-inhibitor immunosuppressants on tubular handling of potassium is the subject of a later section of this chapter.
Mineral Metabolism States After Transplant
Renal transplant handling of elements related to mineral metabolism is perhaps the most complex of the matters discussed in this chapter. The alterations in hormonal status and in the synthesis of regulators of these elements provided by the azotemic milieu coupled to the circumstances that surround surgery and early patient management added to the intrinsic transplant properties contribute to several different distinct syndromes found in a temporal sequence ( Table 94.1 ). Patients may be hypercalcemic, eucalcemic, or hypocalcemic. They may be hypophosphatemic and/or hypomagnesemic. Parathyroid gland function may be normal or exhibit persistent secondary hyperparathyroidism or rarely autonomous function (tertiary hyperparathyroidism). Renal hydroxylation of vitamin D may be subnormal early or normal late. The tubule may intrinsically leak phosphate, calcium or magnesium. This section attempts to isolate these factors and provide a context for understanding the impact of renal transplantation on renal metabolic bone disease.
Hypercalemia |
Persistent secondary hyperparathyroidism |
Tertiary hyperparathyroidism |
Tubular phosphate leak |
Calcium-containing antacids |
Low or normal calcemia |
Early |
High-dose steroids |
1,25-dihydroxy vitamin D |
Late |
Normal renal function |
Tubular calcium leak |
Hypophosphatemia |
Early |
Persistent secondary hyperparathyroidism |
High-dose steroids |
Antacids |
Late |
Persistent secondary hyperparathyroidism |
Tubular phosphate leak |
Hypomagnesemia |
Early |
High-dose steroids |
Diuretics |
Late |
Cyclosporine A |
Almost all allograft recipients have some degree of secondary hyperparathyroidism, which may be unmasked, when the transplant is placed. Serum PTH concentrations decrease progressively during the first three to six months after grafting; however, 1 year after transplantation, resolution of hyperparathyroidism is incomplete in 50% of recipients. Pretransplant factors associated with persistent secondary hyperparathyroidism after kidney transplantation include duration of dialysis, parathyroid gland size, and the development of nodular hyperplasia of parathyroid glands. Due to the long lifespan of parathyroid cells of approximately 20 years and the cell renewal rate of approximately 5% per year, the process of involution of the parathyroid glands may take from a few months to several years. Incomplete normalization of renal function, suboptimal levels of calcitriol and decreased intestinal calcium absorption secondary to corticosteroid therapy also may contribute to the high PTH concentrations after transplant. In great measure, the impact of persistent hyperfunction of the gland characterizes renal handling of ions important for mineral metabolism in transplant recipients.
During the initial period of graft function, hypercalcemia is commonly observed due to the effect of hyperparathyroidism. High PTH concentrations stimulate the renal production of calcitriol leading to increased intestinal calcium absorption and improved skeletal mobilization of calcium. Additional factors contributing to the resolution of the skeletal resistance to PTH include correction of uremia and normalization of serum phosphorus levels, thus facilitating the release of calcium due to osteoclastic bone resorption. Finally, resorption of soft tissue calcifications can contribute to the post-transplant hypercalcemia.
Transient hypercalcemia post-transplant is common, and spontaneous resolution occurs in most cases within one year after grafting. In approximately 5–10% of recipients, hypercalcemia persists beyond the first year but resolves gradually within two to five additional years. Such persistent mild hypercalcemia is usually well tolerated and not associated with untoward effects on the allograft. If severe hypercalcemia (>12 mg/dl) is encountered post-transplant, complications including renal dysfunction, nephrocalcinosis, pancreatitis and vascular calcifications become significant risks and may require elective parathyroidectomy.
During the initial period of graft function, hypercalcemia is commonly observed due to the effect of hyperparathyroidism. Despite elevated PTH levels, the gland retains its capacity to respond to short-term administration of calcium by downregulation of PTH formation and release. The higher levels of ionized calcium required to suppress PTH secretion show that the parathyroid gland has an altered “calcium stat” ( Fig. 94.4 ). In spite of successful renal transplantation, the parathyroid gland continued to require a higher threshold of ionized calcium to suppress function completely. In this circumstance, the higher serum calcium sometimes found in these patients is a consequence of an appropriate response to the fixedly elevated PTH concentration and reset threshold. Other potential causes for hypercalcemia include calcium-containing antacids used to prevent the ulcerogenic potential of high-dose steroids used during the early post-transplant period. Additionally, if serum calcium levels are unable to reach the new set-point for gland suppression parathyroid hyperfunction will continue. There is also the potential for the loss of suppressive ability that calcium has on parathyroid gland function. This conversion from what is called “secondary” to what has been labeled “tertiary” hyperparathyroidism can occur before the transplant surgery or thereafter, but is a rare event. If allowed to continue without surgical reduction in gland mass, tertiary hyperparathyroidism can cause persistent hypercalcemia and hypophosphatemia at a cost primarily to bone mineralization.

Normal or even low levels of serum calcium after successful transplantation in the face of elevated PTH have also been encountered. Confounding variables that characterize the early post-transplant period, which can impair gut absorption of calcium, include high doses of steroids used to prevent rejection and inadequate hydroxylation of vitamin D by the transplant. That the “normal” or low levels of calcium often persist in the setting of persistent elevation of PTH levels, even when gut absorption of calcium returns toward normal as prednisone is reduced and the graft hydroxylation of vitamin D normalizes, suggests the presence of additional alterations in calcium metabolism. By using isotopic calcium excretion studies, Sakhaee et al. were able to show that a primary tubular calcium leak occurred in such patients in spite of normal or even elevated PTH levels, normal 1,25-dihydroxy vitamin D levels, and normal intestinal absorption of calcium. This primary calcium leak provides a persistent stimulus for PTH generation, explaining the measured abnormalities, in part, years after successful transplantation in some patients.
Of all the reported electrolyte abnormalities that follow renal transplantation, hypophosphatemia is the most commonly encountered, occurring in up to 93% of patients during the first few months after transplantation. Although this phenomenon is usually limited to the early post-transplant period, hypophosphatemia may persist for more than 10 years. Traditionally, several pathogenic factors have been implicated in the development of post-transplant hypophosphatemia including persistent hyperparathyroidism, relative deficiency of calcitriol, use of glococorticoids. Pabico and McKenna reconfirmed the findings of McCarron et al. with respect to responsiveness of the parathyroid glands to calcium infusions and extended those findings to examine tubular handling of phosphate. Despite an elevated parathyroid hormone level in the six study patients, an infusion of 15 mg/kg of ionized calcium over 3 hours raised serum calcium into the normal range, lowered serum PTH toward normal levels, and diminished urinary cyclic AMP appropriately. Serum phosphorus rose commensurate with an increase in tubular reabsorption of phosphorous (TRP) reflecting the relationship between TRP and PTH levels. Parfitt et al. confirmed the possibility that a primary leak of phosphorus existed in their study of a group of transplant patients with normal serum calcium, slightly elevated parathyroid hormone level, and normal urinary cyclic AMP, but high tubular rejection rates of phosphorus as a function of glomerular filtration rate. These two studies make several points in general and about phosphorus as a function of glomerular filtration rate. These two studies make several points in general and about phosphate handling in particular. Transplant impact on mineral metabolism is complex. In most, there is persistent hyperparathyroidism even after successful renal transplantation, whichh is responsive to calcium infusion albeit with a higher calcium stat or setpoint. Second, phosphorous handling by the tubule in some reflects PTH levels and in others reflects a primary tubular leak as clearly shown by Graf et al.
These effects may be compounded by the use of immunosuppressive, antacid and diuretic drugs. Micropuncture studies performed on Sprague-Dawley rats by Frick et al. suggest that glucocorticoids may cause an increased tubular rejection of phosphorus in the proximal tublule. Rats receiving a diet stable in calcium and phosphorus infused with a modified Ringer’s lactate solution, which contained an increased dose of inorganic phosphorus, while receiving 0.03 mg/kg/min of hydrocortisone 90 minutes before micropuncture reabsorbed only 29% of the filtered phosphorus load in the proximal convoluted tubule, as opposed to 41% proximal tubule reabsorption in a control group. Another confounding variable in the equation of post-transplant hypophosphatemia is the frequent, often indiscriminate use of antacids. These agents bind phosphate in the gut lumen and prevent absorption. The decreased intestinal absorption magnifies the hypophosphatemia owing to the PTH effect at the tubule and to the intrinsic tubular phosphorus leak. It has become clear in the face of recent data, that none of these factors can explain hypophosphatemia in its entirety. Recently, a new family of phosphorus-regulating hormones termed “phosphatonins” have been described that may play an important role in the pathophysiology of phosphorus wasting in the post-transplant period.
Although elevated PTH levels are noted in the majority of patients after kidney transplantation, a growing body of evidence suggests that it is not the primary cause of defective renal phosphorus reabsoprtion. In one study, identical serium PTH levels were observed in post-transplant recipients with normal and low serum phosphorus concentrations. In addition, suppression of PTH levels with intravenous calcium infusion failed to normalize serum phosphorus concentration and fractional excretion of phosphorus in those sujbects with hypophosphatemia. Further support suggesting a non-PTH humoral mechanism in the development of hypophosphatemia came from an in-vitro study using opossum kidney cells incubated with sera from end-stage renal disease, chronic kidney disease and recipents both “early” (two weeks to one month) and “late” (9 to 12 months) post-kidney transplant. Sodium-dependent phosphorus transport was measured by radioactive phosphorus flux. There was significant inhibition of Na + -dependent phosphorus transport in those with ESRD, CKD and early post-transplant which persisted following the addition of a bovine PTH inhibitor. It was hypothesized that a humorally mediated adaptation mechanism maintains phosphorus homeostasis in renal failure by inhibiting phosphorus transport and sodium/phosphorus activity along the proximal tubule. Persistence of this putative circulating factor for some time following successful transplantation could therefore lead to hypophosphatemia accompanied by increased phosphaturia. Exciting work by several groups have suggested that fibroblast growth factor-23 (FGF-23) may be one such factor.
FGF-23 induces phosphaturia and inhibits renal 1-α hydroxylase leading to decreased calcitriol synthesis. Recent studies have shown that FGF-23 levels increase as CKD progresses and contribute to the declining calcitriol levels observed. In a prospective longitudinal study of 27 patients undergoing living donor kidney transplantation, 85% developed hypophosphatemia, including one who had previously undergone parathyroidectomy. Although FGF-23 levels decreased after transplantation, the levels remained significantly above mean levels reported in healthy individuals. FGF-23 was independently associated with serum phosphate, urinary excretion of phosphate and calcitriol levels. PTH was not independently associated with any of these parameters. This data has been corroborated by others and the term “tertiary hyperphosphatonsim” coined. In longitudinal follow-up of renal transplant recipients, FGF-23 declined over time with regression of renal phosphorus wasting. Taken together, it is reasonable to conclude that high FGF-23 levels that developed as a compensatory mechanism with worsening kidney function, persist post-transplant and contribute to the hypophosphatemia and phosphaturia noted in the early-transplant period. Other phosphatoninins have recently been described including secreted frizzled related protein-4 (sFRP-4), matrix extracellular phosphoglycoprotein (MEPE) and FGF-7. Their physiologic role in kidney transplant recipients remains to be determined.
Hypophosphatemia may be compounded by the use of immunosuppressive, antacid and diuretic drugs. Micropuncture studies performed on Sprague-Dawley rats by Frick et al. suggest that glucocorticoids may cause an increased tubular rejection of phosphorus in the proximal tubule. Rats receiving a diet stable in calcium and phosphorus infused with a modified Ringer’s lactate solution, which contained an increased dose of inorganic phosphorus, while receiving 0.03 mg/kg/min of hydrocortisone 90 minutes before micropuncture reabsorbed only 29% of the filtered phosphorus load in the proximal convoluted tubule, as opposed to 41% proximal tubule reabsorption in a control group. Another confounding variable in the equation of post-transplant hypophosphatemia is the frequent, often indiscriminate use of antacids. These agents bind phosphate in the gut lumen and prevent absorption. The decreased intestinal absorption magnifies the hypophosphatemia owing to the PTH effect at the tubule and to the intrinsic tubular phosphorus leak.
Clinically, patients may present with muscle weakness and osteomalacia when the plasma phosphate concentration is below 1.4 mg/dl. Although severe phosphate depletion with phosphate concentrations below 0.9 mg/dl is rare, it may lead to hemolytic anemia, rhabdomyolysis, decreased myocardial contractility, and respiratory failure. Oral phosphate supplements are capable of normalizing the serum phosphate concentrations after transplantation. Furthermore, the administration of neutral phosphate increases net acid excretion and may lead to a more rapid recovery of the latent metabolic acidosis observed during the early post-transplant period.
Both hypercalcemia and hypophosphatemia are metabolic conditions that may have profound effects on urinary acidification and systemic acid–base status. Studies in animals and humans show that acute infusion of synthetic PTH results in increased proximal tubular bicarbonate wasting and decreased net acid excretion. In a study of artificially induced hyperparathyroidism in normal humans, which is analogous to placing a healthy allograft in a patient with hyperparathyroidism, Hulter and Peterson showed that normal healthy male patients who were fed a stable diet and infused with synthetic PTH at a dose of 6800 U/mg for a period of 12–13 days exhibited a transient, early, mild hyperchloremic metabolic acidosis due to inhibition of proximal tubule bicarbonate regeneration and to a decrease in net titratable acid excretion. When a new steady state was achieved in which net acid returned to normal and plasma bicarbonate levels were elevated, the acidosis was terminated, and subjects were left in a mild metabolic alkalosis. These results oppose the clinical observation that hyperparathyroidism is associated with persistent metabolic acidosis. Although high serum levels of PTH can transiently produce metabolic acidosis because of bicarbonate rejection and a decrease in net acid excretion, the renal or extrarenal mechanism that propagates this metabolic acidosis related to persistently elevated PTH remains uncertain.
Alterations in magnesium homeostasis after transplantation can also be directly related to hyperfunction of the parathyroid gland. This having been said, multiplicities of factors that make up the transplant experience alter the direct relation between excess PTH and magnesium level. Variations in serum levels of magnesium are importantly related to magnesium intake. The protein and caloric malnutrition frequently encountered before and after transplantation is a contributor to magnesium homeostasis. During the early post-transplant period, steroid therapy and diuresis contribute to the hypomagnesaemia. Additionally, hypercalcemia can reduce tubular reabsorption of magnesium, leading to magnesium wasting. Although hypomagnesemia is commonly seen after transplant, it has rarely been of clinical consequence until the use of calcineurin inhibitors. The effects of the calcineurin inhibitors on the handling of magnesium will be discussed later in the chapter.
In summary, the disorders of divalent ion metabolism after transplantation are a function of three factors. The first factor relates to the background into which a working renal transplant is placed, with nutritional status and even more important, persistence of secondary hyperparathyroidism. The second factor is that drugs used after transplant affect the modulation of divalent ions. Finally, the third factor is that intrinsic abnormalities of tubular handling of calcium and phosphorus by recently identified phosphatonins can impair the maintenance of normal mineral metabolite levels and worsen any single abnormality. Taken together, all can synergistically contribute to the development of osteodystrophy and increased cumulative incidence of fractures post-kidney transplant.
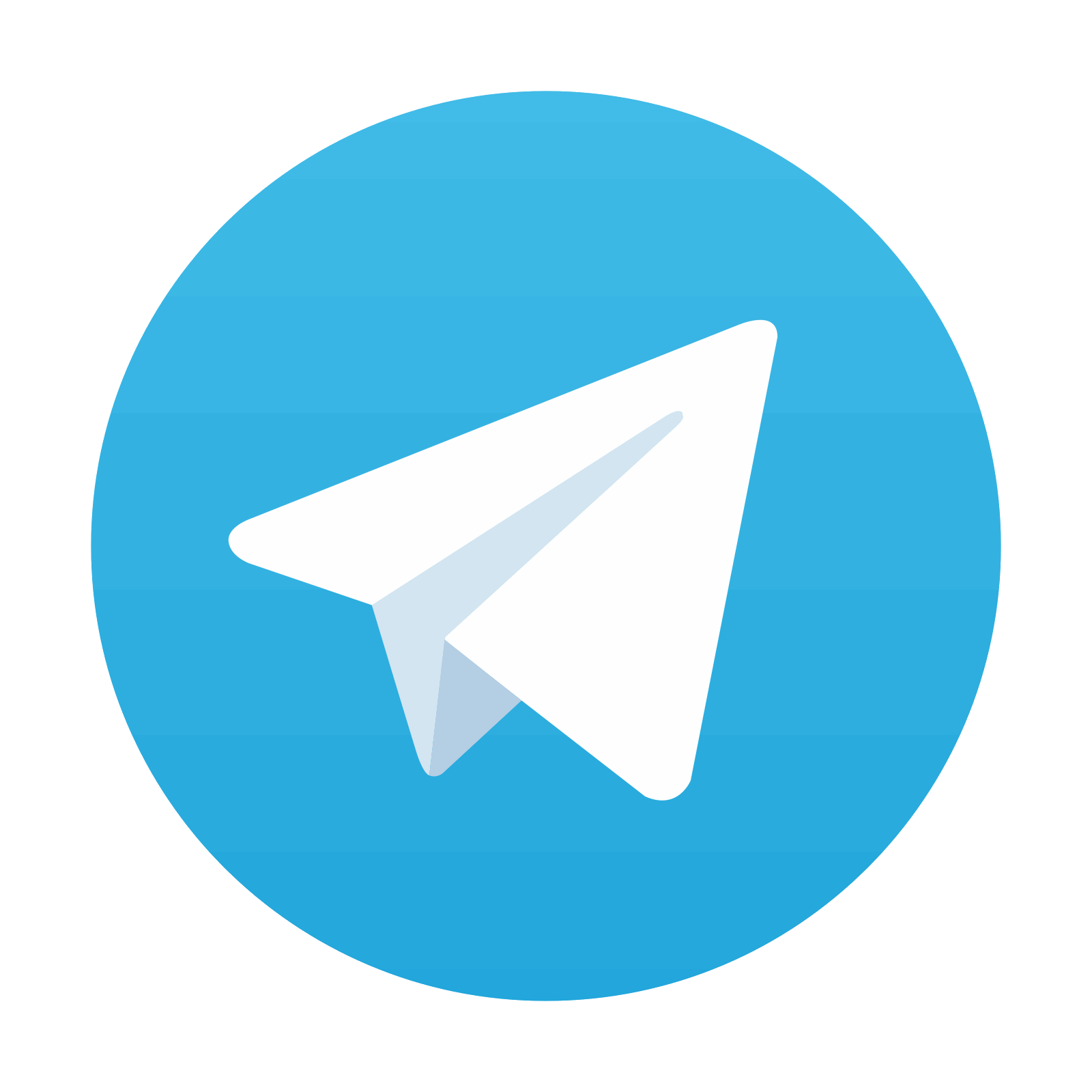
Stay updated, free articles. Join our Telegram channel

Full access? Get Clinical Tree
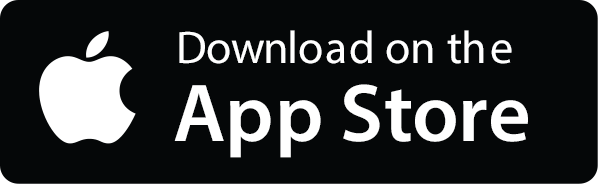
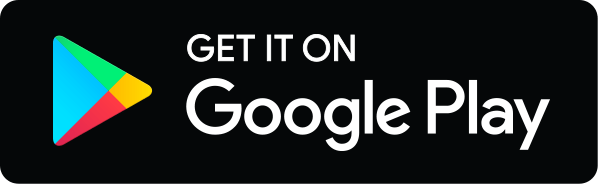