This chapter discusses recent advances in the understanding of genetic kidney diseases that primarily involve the glomerulus. The chapter is organized according to the site or mechanism of the primary abnormality: abnormalities of extracellular matrix, storage diseases affecting the glomerulus, primary defects in the slit diaphragm, cytoskeletal defects and defective transcriptional regulation.
Key words
Alport syndrome, Thin Basement Membrane Nephropathy, HANAC Syndrome, Collagen IV Nephropathies, Pierson Syndrome, Fabry disease, Congenital Nephritic Syndrome of the Finnish Type, Autosomal Recessive Steroid-Resistant Nephritic Syndrome, Nephritic Syndrome, Early Onset Type 3, Autosomal Dominant Focal Segmental Glomerulosclerosis, MYH9 -related diseases (Epstein and Fechtner Syndromes), Denys-Drash Syndrome, Diffuse Mesangial Sclerosis, Frasier Syndrome, Nail-Patella Syndrome
Introduction
The study of inherited diseases arising from single gene defects can provide a wealth of data about the roles that particular proteins normally play in tissue structure and function. The identification of the specific genetic abnormalities underlying several inherited renal disorders has allowed investigators to draw inferences regarding the specific activities of certain glomerular proteins, and the consequences of mutations of these proteins for glomerular function.
In this chapter we will discuss some recent advances in the understanding of genetic kidney diseases that primarily involve the glomerulus. We have organized the chapter according to the site or mechanism of the primary abnormality ( Table 84.1 ): abnormalities of extracellular matrix, storage diseases affecting the glomerulus, primary defects in the slit diaphragm, cytoskeletal defects and defective transcriptional regulation.
Disorders of Extracellular Matrix |
---|
Alport syndrome |
Thin Basement Membrane Nephropathy |
HANAC syndrome |
Pierson Syndrome |
Storage Disorders |
---|
Fabry Disease |
Other Storage Disorders Associated with Glomerular Dysfunction |
Disorders of the Podocyte Slit Diaphragm |
---|
Congenital Nephrotic Syndrome of the Finnish Type |
Autosomal Recessive Steroid-Resistant Nephrotic Syndrome |
Nephrotic Syndrome, Early Onset, Type 3 |
Disorders of the Podocyte Cytoskeleton |
---|
Autosomal Dominant Focal Segmental Glomerulosclerosis |
MYH9 -related disease (Epstein and Fechtner Syndromes) |
Disorders of Podocyte Gene Regulation |
---|
Denys-Drash Syndrome and Diffuse Mesangial Sclerosis |
Frasier Syndrome |
Nail-Patella Syndrome |
Disorders of Extracellular Matrix
Alport Syndrome
The investigation of the molecular biology of Alport syndrome (AS) has provided important insights into the role played by type IV collagen in maintaining the structure and function of the glomerular basement membrane (GBM).The clinical features of AS represent the phenotypic consequences of mutations that alter the expression of type IV collagen in basement membranes.X-linked, autosomal recessive and autosomal dominant forms of AS have been characterized at the gene and protein levels. Absence of three specific isomers of type IV collagen, the α3(IV), α4(IV) and α5(IV) chains, from Alport GBM results early in life in GBM attenuation, manifesting clinically as hematuria. Over time, GBM deficient in these chains undergoes progressive thickening and disorganization, with the development of proteinuria and, ultimately, renal failure.
Clinical Features
Renal Findings : The cardinal finding of AS is hematuria effected males have persistent microscopic hematuria, often first detected during infancy. Many also have episodic gross hematuria during the first two decades of life. Boys who are free of hematuria during the first 10 years of life are unlikely to be affected.
Females who are heterozygous for X-linked AS (XLAS) may have intermittent hematuria, and about 7% of obligate female heterozygotes never manifest hematuria. Hematuria appears to be persistent in both males and females with autosomal recessive AS (ARAS). Proteinuria is usually absent in the first few years of life, but develops eventually in all XLAS males, many XLAS females and in both males and females with recessive disease. Proteinuria increases progressively with age, and may eventuate in the nephrotic syndrome.
Hypertension also increases in incidence and severity with age. Like proteinuria, hypertension is more likely to occur in affected males than in affected females with XLAS, but there are no gender differences in the autosomal recessive form.
End-stage renal disease (ESRD) develops in virtually all affected males with XLAS, but the rate of progression shows significant interkindred variability. Renal half-life in men with XLAS is about 25 years. Several authors have observed that the rate of progression to renal failure is fairly constant among affected males within a particular family although significant intrakindred variability in the rate of progression to renal failure has occasionally been reported.
Progression to ESRD in females with XLAS was, until recently, considered an unusual event.However, in a landmark study of several hundred XLAS females published in 2003, Jais and colleagues found that about 12% developed ESRD before age 40 (compared with 90% of XLAS males), increasing to 30% by age 60 and 40% by age 80 as previously described by Grunfeld et al. Both males and females with ARAS appear likely to progress to ESRD during the second or third decade of life.
Hearing Deficit : About 50% of XLAS males display sensorineural deafness by age 25 and by age 40 approximately 90% of XLAS males are deaf. Although it has been clear for some time that the hearing defect in Alport syndrome localizes to the cochlea, histological correlates of deafness in Alport patients have only recently been recognized. Merchant and colleagues found unique and consistent abnormalities in the cochleae of Alport patients, consisting of a zone of separation between the basilar membrane and the cells of the organ of Corti, associated with a cellular infiltrate in the tunnel of Corti and extracellular spaces of Nuel. Electron microscopy showed that the zone of separation occurred between the basement membrane of the organ of Corti and the basilar membrane.These authors speculated that abnormal adhesion of the organ of Corti to the basilar membrane might disrupt the mechanical relationships between outer hair cells and the basilar membrane, resulting in hearing impairment.
Hearing loss in AS is never congenital and usually becomes apparent by late childhood to early adolescence in boys with XLAS. Hearing impairment in members of families with AS is always accompanied by evidence of renal involvement. There is no convincing evidence that deaf males lacking renal disease can transmit AS to their offspring.In females with XLAS hearing loss is less frequent, tends to be less severe and occurs later in life. There do not appear to be gender differences in the incidence or course of deafness in autosomal disease.
In its early stages the hearing deficit is detectable only by audiometry, with bilateral reduction in sensitivity to tones in the 2000 to 8000 Hz range. In affected males the deficit is progressive and eventually extends to other frequencies, including those of conversational speech. Although these males frequently require hearing aids, their deafness tends to stabilize once they develop ESRD.
Ocular Defects : Ocular defects are not unusual in patients with AS, occurring in at least 15 to 30% of patients. Anterior lenticonus, the conical protrusion of the central portion of the lens into the anterior chamber of the eye, is virtually pathognomonic of AS.Nielsen found that all reported patients with anterior lenticonus who had been adequately examined exhibited evidence of chronic nephritis and sensorineural deafness. Anterior lenticonus is almost entirely restricted to AS families with rapid progression to ESRD (i.e., before age 30) and deafness. Anterior lenticonus is absent at birth, usually appearing during the second to third decade of life. Marked attenuation and fractures of the central portion of the anterior lens capsule have been observed histologically.
A variety of other ocular lesions has been reported in patients with AS. Perhaps the most commonly occurring abnormalities are pigmentary changes in the perimacular region, consisting of whitish or yellowish granulations surrounding the foveal area. Retinal flecks are frequently accompanied by anterior lenticonus but may also occur in the absence of lenticonus. These lesions may arise as a result of abnormalities of Bruch’s membrane, the basement membrane that supports the retinal pigment epithelium.
Corneal endothelial vesicles (posterior polymorphous dystrophy) have been observed in AS patients by several investigators, and may be indicative of abnormalities in Descemet’s membrane, the basement membrane underlying the corneal endothelium. Rhys et al. recently described recurrent corneal erosion in AS patients, which they ascribed to abnormalities of the corneal epithelial basement membrane.
Diffuse Leiomyomatosis : The association of AS with leiomyomas of the esophagus and tracheobronchial tree has been reported in approximately 20 families. Affected females in these kindreds typically exhibit genital leiomyomas as well, with clitoral hypertrophy and variable involvement of the labia majora and uterus. Symptoms usually appear in late childhood and include dysphagia, post-prandial vomiting, retrosternal or epigastric pain, recurrent bronchitis, dyspnea, cough and stridor. Leiomyomatosis may be suspected by chest X-ray or barium swallow, and confirmed by computed tomography or magnetic resonance imaging.
Arterial Disease : There have been several reports of arterial disease in males with Alport syndrome, including aneurysms of the thoracic and abdominal aorta and the cerebral arterial system.
Pathology
There are no pathognomonic lesions by light microscopy or direct immunofluorescence in AS. Electron microscopy frequently reveals diagnostic abnormalities. The cardinal ultrastructural feature of the kidney in AS is the variable thickening, thinning, basket-weaving, and lamellation of the GBM ( Figures 84.1 and 84.2 ). Identical morphologic features are apparent in some patients without a family history of nephritis. Such patients may represent new COL4A5 mutations, they may be offspring of asymptomatic female carriers, or may have autosomal recessive disease.


Ultrastructural alterations of GBM were noted in several reports of hereditary nephritis in the 1960s. The most prominent abnormality is the irregular appearance of the lamina densa, which demonstrates zones of thickening, thinning, and splitting that may be interposed with segments of GBM that appear entirely normal. The abnormalities can affect a portion of a capillary loop, an entire loop, some or all loops, and can even spare individual glomeruli.
The thick segments measure up to 1200 nm, usually have irregular outer and inner contours, and are found more commonly in males than in females. The lamina densa is transformed into a heterogeneous network of membranous strands, which enclose clear electron-lucent areas. The electron-lucent zones may be entirely clear or contain round granules of variable density that measure between 20 and 90 nm in diameter. The origin of these granules is unknown, but Rumpelt has hypothesized that they represent degenerating islands of visceral epithelial cell cytoplasm. Young children and females, and adult males on occasion, may have diffusely attenuated segments of GBM measuring as little as 100 nm or less;in some instances, discontinuities of the GBM have been observed.
The specificity of the GBM changes has been questioned. Focal lamina densa splitting and lamellation may be observed in renal biopsies from patients with a variety of glomerular disorders. Clinical correlation and immunofluorescence microscopic examination of all biopsies are necessary when AS is the suspected diagnosis. Diffuse thickening and splitting of the GBM is strongly suggestive of AS.In the absence of immune deposits, these changes are pathognomonic.
Not all Alport kindreds demonstrate these characteristic ultrastructural features. Thick, thin, normal and nonspecifically altered GBM have all been described. Although diffuse attenuation of GBM has been considered the hallmark of “benign familial hematuria” or thin basement membrane nephropathy, some patients with this abnormality are members of kindreds with a history of progression to renal failure. Thus the signficance of an ultrastructural finding of thin GBMs must be considered in the context of the family history, basement membrane expression of type IV collagen α chains (see below), and genetic information.
Rumpelt described a correlation between the percent of GBM showing splitting of the lamina densa and the degree of proteinuria in AS patients, suggesting that impaired permselectivity may be a functional consequence of the GBM alteration. Kim and colleagues observed that morphometric parameters such as mesangial volume fraction, cortical interstitial volume fraction, and percent global glomerular sclerosis were inversely correlated with creatinine clearance in AS, while creatinine clearance and surface density of peripheral GBM were directly correlated. Similar relationships have been observed in other diseases, such as diabetic nephropathy and membranoproliferative glomerulonephritis (MPGN). However, patients with AS showed significantly greater impairment of filtration for any degree of structural change in comparison with patients with diabetic nephropathy or MPGN. This observation suggests that these morphologic abnormalities only partially account for reductions in creatinine clearance in AS. Decreased conductivity of water across the altered glomerular capillary wall could contribute to the decrement in filtration.
Genetics and Pathophysiology
Genetics and Biochemistry of Type IV Collagen
Glomerular basement membranes are composed of several major constituents, including type IV collagen, laminin, nidogen and heparan sulfate proteoglycan.The type IV collagen family of proteins comprises six isomeric chains, designated α1–α6(IV), ( Table 84.2 ). These chains show extensive sequence homology and share basic structural features, including: (1) a major collagenous domain of about 1400 residues containing the repetitive triplet sequence glycine(Gly)-X-Y, in which X and Y represent a variety of other amino acids. (2) A carboxy-terminal noncollagenous (NC1) domain of about 230 residues. (3) A noncollagenous amino-terminal sequence of 15–20 residues. The major collagenous domain of each chain contains about 20 interruptions of the collagenous triplet sequence, while each noncollagenous domain contains 12 completely conserved cysteine residues which participate in critical disulfide bonds.
Chain | Gene | Chromosome | Collagenous Domain # Residues (Interruptions) | Noncollagenous Domain # Residues |
---|---|---|---|---|
α1(IV) | COL4A1 | 13 | 1413 (21) | 229 |
α2(IV) | COL4A2 | 13 | 1449 (23) | 227 |
α3(IV) | COL4A3 | 2 | 1410 (23) | 233 |
α4(IV) | COL4A4 | 2 | 1421 (26) | 231 |
α5(IV) | COL4A5 | X | 1430 (22) | 229 |
α6(IV) | COL4A6 | X | 1417 (25) | 228 |
Each type IV collagen molecule is a trimer composed of three α chains.The classical type IV collagen trimer isolated from the extracellular matrix of the murine EHS sarcoma has the composition α1(IV) 2 -(α2(IV) 1 . Type IV collagen α chains form trimers through associations between their carboxy-terminal NC1 domains, accompanied by folding of the collagenous domains into triple helices. Variable residues within the NC1 domains confer specificity upon chain-chain associations. Type IV collagen triple helices form networks through several types of intermolecular interaction:end-to-end linkages between the carboxy-terminal domains of two type IV collagen triple helices, covalent interactions between four triple helices at their aminoterminal ends, and lateral associations mediated by binding of the carboxy-terminal domains of one trimer to the collagenous region of another trimer. Disulfide bonds involving conserved cysteine residues are critical to the interactions between NC1 domains. These linkages between type IV collagen molecules produce a nonfibrillar, open-network assembly that associates with laminin through interactions mediated by nidogen.
Various integrins mediate cell attachment to type IV collagen.Attachment of glomerular epithelial cells to the α3(IV) chain appears to involve the α3β1 integrin.
The six type IV collagen genes are arranged in pairs on three different chromosomes ( Figure 84.2 , Table 84.2 ). The human α1(IV) and α2(IV) chains are encoded by the genes COL4A1 and COL4A2 , respectively, on chromosome 13. COL4A3 and COL4A4 are located on chromosome 2 and encode the α3(IV) and α4(IV) chains of type IV collagen, respectively, while the α5(IV) and α6(IV) chains are encoded by the COL4A5 and COL4A6 genes on the X chromosome. The 5′ ends of each gene pair are adjacent to each other, separated by sequences of varying length containing motifs involved in the regulation of transcriptional activity.
Evidence from a variety of sources indicates the existence of at least three type IV collagen networks in mammalian basement membranes. Studies of the distribution of these networks in basement membranes using monospecific antibodies have revealed a high degree of tissue specificity ( Table 84.3 ). A network composed of heterotrimers with the composition α1(IV) 2 -α2(IV) is found in all basement membranes, although it is a relatively minor component of mature GBM.A network comprising α3(IV)-α4(IV)-α5(IV) heterotrimers is the predominant collagen in mature GBM, and also occurs in Bowman’s capsules and the basement membranes of distal and collecting tubules. The network consisting of α5(IV) 2 -α6(IV) heterotrimers is present in Bowman’s capsule and distal and collecting tubule basement membranes, but is not present in GBM. The epidermal basement membraneand basement membranes surrounding aortic smooth muscles cells (EBM) contains α1(IV) 2 -α2(IV) and α5(IV) 2 -α6(IV) networks, but not the α3(IV)-α4(IV)-α5(IV) network.
Chain | Kidney | Eye | Cochlea | Skin |
---|---|---|---|---|
α1(IV) | All BMs mesangium | All BMs | All BMs | All BMs |
α2(IV) | All BMs mesangium | All BMs | All BMs | All BMs |
α3(IV) | GBM, | Lens capsule | Inner/outer sulci | None |
BC | Descemet’s membrane | Spiral limbus | ||
dTBM | Bruch’s membrane | Spiral prominence | ||
Internal limiting membrane | Basilar memb | |||
α4(IV) | GBM | Lens capsule | Inner/outer sulci | None |
BC | Descemet’s membrane | Spiral limbus | ||
dTBM | Bruch’s membrane | Spiral prominence | ||
Internal limiting membrane | Organ of Corti BM | |||
α5(IV) | GBM | Lens capsule | Inner/outer sulci | DEJ |
BC | Descemet’s membrane | Spiral limbus | ||
dTBM | Bruch’s membrane | Spiral prominence | ||
Internal limiting membrane | Organ of Corti BM | |||
α6(IV) | BC | Unknown | Unknown | DEJ |
dTBM |
The α3(IV) chain has been identified as the target of anti-GBM autoantibodies in Goodpasture syndrome. The Goodpasture epitope resides in the carboxy-terminal NC1 domain of α3(IV).
Abnormalities of Type IV Collagen Genes and Proteins in Alport Syndrome
There are three genetic forms of Alport syndrome ( Table 84.4 ).The X-linked form (XLAS), resulting from mutations in COL4A5 , accounts for about 80% of patients with the disease. About 15% of patients have autosomal recessive Alport syndrome (ARAS), which arises from mutations affecting both alleles of COL4A3 or COL4A4 . The heterozygous parents of children with ARAS often have asymptomatic hematuria, although some have normal urinalyses.Finally, approximately 5% of patients have autosomal dominant Alport syndrome (ADAS), due to heterozygous mutations in COL4A3 or COL4A4. Heterozygous mutations in COL4A3 or COL4A4 have also been found in families with thin basement membrane nephropathy (see below). It is not yet clear why many, perhaps most, individuals with heterozygous mutations in these genes have asymptomatic hematuria, while others have progressive disease.
Affected Inheritance | Locus | Gene Product |
---|---|---|
X-linked | COL4A5 | α5(IV) |
X-linked+leiomyomatosis | COL4A5 + COL4A6 | α5(IV)+α6(IV) |
Autosomal recessive | COL4A3 | α3(IV) |
COL4A4 | α4(IV) | |
Autosomal dominant | COL4A3 | α3(IV) |
COL4A4 | α4(IV) |
XLAS : Several hundred COL4A5 mutations have been reported in XLAS families.These mutations are distributed throughout the gene, and with few exceptions each mutation is unique. About 20% of reported COL4A5 mutations are large rearrangements, predominantly deletions. Missense mutations account for about 35-40%, about 15% are splice-site mutations, and 25–30% are nonsense mutations or small frame-shifting deletions or insertions that result in premature stop codons. About 10 to 15% of COL4A5 mutations occur as spontaneous events in the proband, explaining why some patients with XLAS lack a family history of the disease.
The association of XLAS with leiomyomatosis of the esophagus and tracheobronchial tree has been reported in several dozen families. Affected members of these families exhibit large deletions that span the adjacent 5’ ends of the COL4A5 and COL4A6 genes. These deletions involve varying lengths of COL4A5 , but the COL4A6 breakpoint is always located in the second intron of the gene. Leiomyomatosis does not occur in patients with deletions of COL4A5 and COL4A6 that extend beyond intron 2 of COL4A6 .Mutations of COL4A6 alone do not appear to cause Alport syndrome, consistent with the absence of the α6(IV) chain from normal GBM.
The great majority of missense COL4A5 mutations are guanine substitutions in the first or second position of glycine codons that result in the replacement of a glycine residue in the collagenous domain of α5(IV) by another amino acid. Such mutations are thought to interfere with the normal folding of the mutant α5(IV) chain into triple helices with other type IV collagen α chains. Glycine lacks a side chain, making it the least bulky of amino acids, and small enough to allow three glycine residues to fit into the interior of a tightly wound triple helix. The presence of a bulkier amino acid in a glycine position presumably creates a kink or an unfolding in the triple helix. Glycine substitutions in the α1 chain of type I collagen account for the majority of mutations causing osteogenesis imperfecta, and are common in other genetic disorders of collagen. Abnormally folded collagen triple helices exhibit increased susceptibility to proteolytic degradation. The position of the substituted glycine, or the substituting amino acid itself, may influence the effect of the mutation on triple-helical folding, and ultimately the impact of the mutation on the severity of the clinical phenotype.
ARAS : To date, mutations causing ARAS have been found in the COL4A3 or COL4A4 gene in several dozen patients. Some of these patients are homozygous for their mutations and others are compound heterozygotes. As with COL4A5 , there appear to be no mutation hot spots in COL4A3 or COL4A4 . Although it is possible that ARAS could result from the combination of a mutation in one allele of COL4A3 and a mutation in one allele of COL4A4 , no such example has been described. It is worth noting that the mating of two individuals with asymptomatic hematuria due to heterozygous COL4A3 or COL4A4 mutations can result in a child who has mutations in both alleles of COL4A3 or COL4A4 and, as a result, ARAS.
The reported COL4A3 and COLA 4 mutations in ARAS include nonsense, frame-shift, splicing and missense mutations. As with XLAS and other heritable collagen disorders, a common type of mutation in ARAS is a glycine substitution in the collagenous domain of α3(IV) or α4(IV).
ADAS : Heterozygous COL4A3 and COL4A4 mutations have been described in several families transmitting Alport syndrome as an autosomal dominant disease. It is not clear why some individuals with heterozygous COL4A3 or COL4A4 mutations are asymptomatic or exhibit only isolated microhematuria, while others have a progressive nephropathy. Several possibilities can be proposed: the type and/or site of the mutation may be critical, the presence of certain polymorphisms in these genes may influence the effect of a pathogenic mutation, or a polymorphism or mutation in another gene may modify the effect of the mutation. In some cases, a heterozygous missense mutation in COL4A3 or COL4A4 might be more detrimental than a deletion or nonsense mutation, because the mutant chain can then induce the degradation of normal chains with which it forms abnormal trimers ( Figure 84.1C ).
Genotype-Phenotype Correlations in Alport Syndrome : Male patients with COL4A5 deletions consistently exhibit sensorineural deafness and progression to ESRD during the second or third decade of life. Most of the missense, nonsense and splicing COL4A5 mutations described thus far have also been associated with early progression to ESRD in the second or third decade of life and sensorineural deafness. Single amino acid substitutions that alter an mRNA splice site, leading to exon skipping, or small deletions or insertions that shift the transcriptional reading frame, can clearly produce such aberrant protein products that a severe phenotype results. Several missense mutations in COL4A5 have been associated with late-onset (after the third decade) ESRD and deafness. Mutations near the 5’ end of the COL4A5 gene are associated with earlier onset of ESRD.
The severity of disease in a female heterozygous for a COL4A5 mutation is probably influenced to some extent by the nature of the mutation, but the extent of inactivation of the X chromosome carrying the normal COL4A5 allele may be a more important factor. Jais et al. were unable to demonstrate a genotype effect on outcome in XLAS heterozygotes, presumably due to the stronger influence of X-inactivation. Guo et al. described a woman with a severe Alport phenotype (ESRD at age 30), one of whose COL4A5 alleles carried two missense mutations. Analysis of DNA isolated from the patient’s kidney and leukocytes showed inactivation of greater than 90% of the X chromosomes carrying the normal COL4A5 allele. Other investigators, however, have not been able to confirm that X-inactivation pattern of leukocytes predicts phenotype in XLAS heterozygotes. In a mouse model of X-linked Alport syndrome the relative activity of the X chromosome carrying the mutant COL4A5 allele determines the outcome of heterozygous females.
Phenotypic information on patients with ARAS is as yet somewhat sparse. Available data indicate that patients with ARAS progress to ESRD before age 30 and have sensorineural deafness, regardless of gender.
Type IV Collagen in Alport Basement Membranes : Several studies in the 1980s established that the native kidneys of male AS patients failed to bind anti-GBM antibodies from patients with Goodpasture syndrome or from AS patients with post-transplant anti-GBM nephritis. These early studies, combined with the observation by Hudson and colleagues that type IV collagen was the target of Goodpasture antibodies, provided the first indication that AS might represent a primary disorder of type IV collagen. The availability of chain-specific antibodies has more recently allowed detailed investigation of the expression of the six type IV collagen α chains in AS basement membranes.
GBM, distal and collecting tubular basement membrane (TBM) and Bowman’s capsules of males with XLAS usually lack expression of the α3(IV), α4(IV) and α5(IV) chains, but do express the α1(IV) and α2(IV) chains ( Table 84.5 , Figure 84.3 ). The α6(IV) chain is not expressed in Bowman’s capsule or distal TBM of XLAS males whose basement membranes lack α5(IV) expression. Women who are heterozygous for XLAS mutations frequently exhibit mosaicism of GBM expression of the α3(IV), α4(IV) and α5(IV) chains, while expression of the α1(IV) and α2(IV) chains is not affected. The epidermal basement membrane (EBM) normally expresses the α1(IV), α2(IV), α5(IV) and α6(IV) chains, but not the α3(IV) or α4(IV) chains ( Table 84.5 ). Most males with XLAS show no EBM expression of α5(IV) or α6(IV), while female heterozygotes frequently display mosaicism ( Table 84.5 , Figure 84.1 ). Lens capsules of some males with XLAS do not express the α3(IV), α4(IV) or α5(IV) chains, while expression of these chains appears normal in other patients.
GBM | BC | dTBM | EBM | |
---|---|---|---|---|
X-linked (males) | ||||
α3(IV) | − | − | − | normally absent |
α4(IV) | − | − | − | normally absent |
α5(IV) | − | − | − | − |
X-linked (females) ** | ||||
α3(IV) | mosaic | normally absent | ||
α4(IV) | mosaic | normally absent | ||
α5(IV) | mosaic | mosaic | ||
Autosomal recessive (males and females) | ||||
α3(IV) | − | − | − | normally absent |
α4(IV) | − | − | − | normally absent |
α5(IV) | − | + | + | + |
* In some AS kindreds, staining of basement membranes for type IV collagen chains is entirely normal.Therefore, a normal result does not exclude a diagnosis of X-linked or autosomal recessive AS.
** Some heterozygous females have normal basement membrane immunoreactivity for type IV collagen chains. Thus, a normal result does not exclude the carrier state.

In patients with ARAS, GBMs show no expression of the α3(IV), α4(IV) or α5(IV) chains, but α5(IV) and α6(IV) are expressed in Bowman’s capsule, distal TBM and EBM ( Table 84.5 , Figure 84.3 ). Therefore, XLAS and ARAS may be distinguishable by immunohistochemical analysis.
It is important to note that immunostaining for type IV collagen is normal in some patients with XLAS and ARAS. Consequently, normal expression of α3(IV), α4(IV) and α5(IV) chains in basement membranes does not exclude a diagnosis of AS.
The abnormalities of type IV collagen expression observed in AS indicate that a mutation affecting one of the chains involved in the α3(IV)-α4(IV)-α5(IV) network can prevent GBM deposition of the entire network. Similarly, a mutation involving the α5(IV) chain can interfere with basement membrane expression of α5(IV)-α6(IV). Several lines of evidence suggest that the effects of a type IV collagen α chain mutation on the basement membrane expression of its “partner” chains are mediated by post-translational events. Substitution of glycine residues in the α1 chain of type I collagen comprise the majority of mutations causing osteogenesis imperfecta. These mutations impair the folding of α1(IV) chains with α2(IV) chains into trimers, and the resulting trimers exhibit enhanced susceptibility to extracellular proteolytic degradation. By similar means, an α5(IV) chain carrying a glycine substitution could bring about the destruction of normal α3(IV) and α4(IV) chains. Studies of mutations in the α1 chain of type IV collagen in C. elegans suggest another possible mechanism. Whether the α1(IV) mutation results in a null allele (deletion or nonsense mutation) or a glycine substitution, the α2(IV) chain accumulates intracellularly and never reaches the basement membrane. Thus, mutations producing null COL4A5 alleles, or α5(IV) proteins that cannot form trimers (due to alterations of the carboxy-terminal domain), or some glycine substitutions, may simply prevent secretion of α3(IV) and α4(IV) chains, which are eventually degraded. Results of in vitro studies by Kobayashi and colleagues support the hypothesis that α5(IV) mutations impair the assembly of α3(IV)-α4(IV)-α5(IV) heterotrimers.
Pathogenesis of Basement Membrane Lesions in Alport Syndrome : The tissue pathology of AS arises from abnormalities of basement membrane expression of the α3(IV)-α4(IV)-α5(IV) collagen network. This network is usually absent from or under-expressed in the basement membranes of AS patients, although in the basement membranes of some patients the networks are present but presumably defective in structure and function. The most straightforward demonstration of the consequences of the absence of this network may be anterior lenticonus. Absence or defective expression of these chains results in thinning and mechanical weakness of the anterior lens capsule, which fails to maintain the normal conformation of the lens. Microhematuria, the first and invariable renal manifestation of AS, may likewise reflect GBM thinning and a tendency to develop focal ruptures early in life, due to the absent or defective expression of the α3-α4-α5(IV) network. Episodic gross hematuria precipitated by infections, which is not uncommon during the first two decades of life, may reflect increased susceptibility of the GBM to proteolysis. On the other hand, the resolution of gross hematuria, which typically occurs by adolescence, may be a function of progressive GBM thickening.
The relentless thickening and lamellation of the Alport GBM features the accumulation of extracellular matrix proteins that are normally absent from GBM, or present in only small amounts. The α1(IV)–α2(IV) network is highly expressed in the basement membranes of primordial glomeruli, but is normally replaced by the α3(IV)–α5(IV) network at the capillary loop stage of glomerular maturation. In mature glomeruli the α1(IV)–α2(IV) network is confined to the mesangium and the subendothelial region of GBM. This developmental switch fails to occur in Alport glomeruli. The α1(IV)–α2(IV) network persists in mature Alport glomeruli and accumulates, spreading from its normal subendothelial location to occupy the full width of GBM. Similarly, type V collagen and type VI collagen are normally found in the mesangium and in small quantities in the subendothelial GBM, but are markedly overexpressed in Alport GBM. In addition, laminin chains that are normally confined to the mesangium are expressed in Alport GBM. The molecular events that underlie these anomalies in protein expression remain to be elucidated. Whatever the mechanism, glomerulosclerosis in AS may result from the ectopic deposition of certain collagens in GBM.
AS resembles other chronic glomerulopathies in that deterioration of glomerular filtration rate is closely correlated with fibrosis of the renal interstitium. Measurable increases in cortical interstitial volume are unusual in males with XLAS under age 10 years, but progressive expansion of the interstitium frequently occurs during the second decade of life. It is not surprising that at least some of the factors driving interstitial fibrosis in AS are nonspecific, such as increased TGF-β1 activity, monocytic infiltration, epithelial-mesenchymal transformation and enhanced metalloproteinase expression. If the pathogenesis of interstitial fibrosis in AS is not specific to the disease, it is conceivable that therapies that interfere with interstitial fibrosis may be of benefit to AS patients, without correcting the primary abnormalities of type IV collagen expression.
Diagnosis
Alport syndrome should be considered in the differential diagnosis of patients with persistent microhematuria. Electron microscopic examination of renal tissue remains the most widely available and applied means for diagnosing AS. The presence of diffuse thickening and multilamellation of the GBM predicts a progressive nephropathy, regardless of family history. Unfortunately, ultrastructural information alone does not establish the mode of transmission in a particular family. In a patient with a negative family history, electron microscopy cannot distinguish de novo X-linked disease from autosomal recessive disease. In some patients the biopsy findings may be amibiguous, particularly females and young patients of either sex. Furthermore, rare families with progressive nephritis and COL4A5 mutations in association with thin GBMs have been described, indicating that the classic GBM lesion is not present in all AS kindreds.
In families with a firm diagnosis of AS, evaluation of individuals with newly-recognized hematuria can be limited to ultrasound of the kidneys and urinary tract in most instances. In the absence of tumor or structural anomalies of the urinary tract, a diagnosis other than AS is unlikely. A suspected diagnosis of AS may be confirmed by immunohistochemical methods. Monospecific antibodies directed against type IV collagen α chains are available, making it possible to reliably evaluate renal basement membranes for expression of α3(IV), α4(IV) and α5(IV) chains ( Table 84.5 ). Since the α5(IV) chain is normally expressed in the epidermal basement membrane (EBM), examination of skin biopsies by immunofluorescence for expression of α5(IV) is an additional tool for making a diagnosis of AS. Absence of the α5(IV) chain from EBM is diagnostic of XLAS. Given a male patient with a positive family history and clinical features characteristic of AS, examination of skin for α5(IV) expression may obviate the necessity for kidney biopsy. Unfortunately, a normal result does not exclude the diagnosis of AS, since in some XLAS kindreds (about 20%) affected males express α5(IV) in their renal and epidermal basement membranes.Heterozygous females frequently express α5(IV) mosaically in the skin. While clearly mosaic expression of α5(IV) is diagnostic of the carrier state, a normal result does not exclude heterozygosity.
Renal expression of type IV collagen α chains can serve to confirm a diagnosis of AS, and can in addition differentiate XLAS and ARAS ( Table 84.5 ).In most males with XLAS renal basement membranes are devoid of the α3(IV), α4(IV) and α5(IV) chains, while females frequently show mosaic expression of these chains. In most males and females with ARAS the GBM, Bowman’s capsule and distal TBM show no expression of the α3(IV) and α4(IV) chains, while α5(IV) is expressed in Bowman’s capsule and distal TBM but not GBM.
The ability to diagnose Alport syndrome by skin biopsy may eventually reduce reliance on kidney biopsy for diagnosis of this disease. Skin biopsy is the initial diagnostic maneuver of choice when kidney biopsy is excessively risky, such as in patients with advanced renal insufficiency.
Genetic analysis provides the only means for reliably diagnosing the carrier state in asymptomatic female members of XLAS kindreds and for making a prenatal diagnosis of AS. There are also clinical situations in which a firm diagnosis of AS cannot be established, or in which it is not possible to determine the mode of transmission, despite careful evaluation of the pedigree and application of the full range of histological methods. In these situations genetic analysis has the potential to provide information essential for determining prognosis and guiding genetic counseling. Genetic counseling based upon erroneous determination of the mode of inheritance may have unintended consequences.
At the present time, the routine application of molecular analysis is limited by both specific and general problems. First, the sheer size of the type IV collagen loci (>50 exons), combined with the great variety of mutations, many of which are missense alterations of a single base pair, presents a formidable obstacle. Exon-screening methods, such as analysis of single-strand conformational polymorphisms, identify only about 50% of COL4A5 mutations. Detection schemes based on direct sequencing identify have a better detection rate (about 80–90%), but are relatively expensive. Consequently, at the time this chapter was written, only a single commercial laboratory offered molecular diagnosis of Alport syndrome ( www.genereviews.org ).
In the future, automation and miniaturization of DNA analytic techniques may result in a relatively simple, rapid and inexpensive means of assaying a blood or tissue sample for mutations at genetic loci relevant to Alport syndrome, replacing other diagnostic methods. For now, genetic analysis is desirable, but not necessarily available, when there is ambiguity regarding the diagnosis of Alport syndrome or the mode of transmission, or when prenatal testing is desired.
Treatment
Replacement of renal function by transplantation is currently the only “treatment” of proven value for AS. Analysis of data from the North American Pediatric Renal Transplant Cooperative Study (NAPRTCS) documents equivalent allograft survival rates in patients with familial nephritis, in comparison to patients with other diagnoses. However, some AS patients develop anti-GBM nephritis in the renal allograft, usually resulting in graft loss.Data from several transplant centers indicates an incidence of 3–4% in transplanted AS patients. These patients are usually male, always deaf, and likely to have reached ESRD before the age of 30. This profile describes the majority of AS patients presenting for renal transplantation, so its predictive value is rather limited. However, AS patients with normal hearing or late progression to ESRD appear to be at very low risk for the development of post-transplant anti-GBM nephritis. Females with XLAS also appear to be in a low-risk category.
The onset of post-transplant anti-GBM nephritis was within the first year following transplantation in approximately 75% of cases. Three-quarters of the allografts failed irreversibly, usually within a few weeks to months after diagnosis.Treatment with plasmapharesis and cyclophosphamide has been of limited benefit. Anti-GBM nephritis has recurred in seven of eight patients who underwent retransplantation. Post-transplant anti-GBM nephritis may recur despite an interval of many years between transplants, and in the absence of detectable circulating anti-GBM antibodies prior to retransplantation.
The target(s) of anti-GBM antibodies in some of these patients has been determined, with variable results. Most patients with XLAS exhibit antibodies that target the carboxy-terminal noncollagenous domain of the α5(IV) chain, but anti-α3(IV) antibodies have also been described. Antibodies against α3(IV) have been observed in ARAS patients with post-transplant anti-GBM nephritis.
It has been proposed that mutations in the COL4A5 gene that prevent expression of an immunogenic gene product, thereby preventing the establishment of tolerance for α5(IV), might be associated with an increased risk for the development of post-transplant anti-GBM nephritis. Of 14 AS patients with post-transplant anti-GBM nephritis examined for a COL4A5 mutation, seven, or 50%, had complete or partial deletions, compared with a deletion frequency of about 10% in the general AS population (discussed in ). Although the sample size is small, the data gathered thus far support the hypothesis that AS patients who develop anti-GBM nephritis after renal transplantation are more likely to carry deletions in the COL4A5 gene than the AS population at large.
Females who are heterozygous for COL4A5 mutations would not be expected to be at risk for the development of post-transplant anti-GBM nephritis, since the product of the normal COL4A5 allele would allow establishment of immunologic tolerance for α5(IV). Nevertheless, post-transplant anti-GBM nephritis has been reported in two females with AS. Both of these women were found to have ARAS, due to COL4A3 mutations.
Even if it were to prove true that certain COL4A5 mutations are associated with a higher risk for the development of post-transplant anti-GBM nephritis, this information would be of limited value in planning transplantation. It is clear that AS males with COL4A5 deletions can undergo renal transplantation without developing anti-GBM nephritis, indicating that other factors, presently unknown, must influence the initiation and elaboration of the immune response to the allograft. At this time it appears that the only way to determine whether a previously untransplanted AS patient will develop post-transplant anti-GBM nephritis is to perform the transplant, although as noted previously certain patients are at very low risk.
Interventions aimed at preventing or slowing the inexorable decline in renal function typical of male AS are currently under investigation. The role of angiotensin II in the pathogenesis of renal failure in AS is still not well understood. Studies of angiotensin inhibition in AS have involved small numbers of patients with proteinuria serving as their own controls, with relatively short follow-up, and have produced mixed results. However, Gross and colleagues found that ramipril therapy initiated prior to the development of proteinuria delayed the onset of proteinuria and renal failure and lengthened survival in a murine model of ARAS. Early angiotensin converting enzyme inhibition in dogs with XLAS had no effect on the onset of proteinuria, but did delay ESRD. These observations suggest that angiotensin blockade, initiated early in the course of disease might be beneficial in human AS.
Callis and colleagues found, in an uncontrolled study of eight AS males, that cyclosporine appeared to suppress proteinuria and stabilize renal function, over several years of observation. There has yet to be a published report that contradicts these findings. However, the results of trials presented in abstract suggest that patient responses to cyclosporine are variable, and that cyclosporine may accelerate interstitial fibrosis. In dogs with XLAS, cyclosporine failed to suppress proteinuria, but treated animals did exhibit a delay in progression to ESRD. At this time, it is the authors’ view that the efficacy of cyclosporine as a treatment for AS has not been demonstrated with the degree of certainty needed to overcome concerns about its nephrotoxicity.
Gene transfer approaches aimed at delivering a normal copy of the COL4A5 gene to glomeruli are currently undergoing investigation in canine models of AS.
Thin Basement Membrane Nephropathy (TBMN)
TBMN, like AS, is an inherited disorder of glomerular basement membranes that is characterized clinically by persistent microscopic hematuria and episodic gross hematuria. TBMN differs clinically from AS in several important respects: (1) It is only rarely associated with extrarenal abnormalities. (2) Proteinuria, hypertension and progression to ESRD are unusual. (3) Gender differences in the severity of the signs and symptoms of TBMN are not apparent. (4) Transmission is autosomal dominant in nature.TBMN and early AS may be difficult to distinguish histologically, since diffuse GBM attenuation is characteristic of both. However, the GBM of TBMN patients remains attenuated over time, rather than undergoing the progressive thickening and multilamellation that is pathognomonic of AS.
Clinical Features
Individuals with TBMN typically exhibit persistent microhematuria that is first detected in childhood. In some patients microhematuria is intermittent, and may not be detected until adulthood. Episodic gross hematuria, often in association with upper respiratory infections, is not unusual. The hematuria of TBMN appears to be life-long.It has been estimated that 20–25% of patients referred to a nephrologist for evaluation of persistent hematuria will prove to have thin GBM on renal biopsy.
Overt proteinuria and hypertension are unusual in TBMN, but have been described. Some of these patients exhibit focal global glomerulosclerosis on renal biopsy. In occasional adult patients with Alport syndrome the predominant GBM abnormality of is attenuation, rather than thickening and multilamellation. Other glomerular disorders such as IgA nephropathy may occur in patients with TBMN, altering the expected natural history and histopathology of the condition.
Pathology
Light and immunofluorescence microscopy are unremarkable in typical cases of TBMN or thin GBM disease. Most patients with TBMN exhibit diffuse thinning of the GBM as a whole, and of the lamina densa ( Figure 84.3 ). Normal GBM thickness is age- and sex-dependent. Both the lamina densa and the GBM normally increase rapidly in thickness between birth and age two years, followed by gradual thickening throughout childhood, adolescence and into adulthood. GBM thickness of adult men (373±42 nm) exceeds that of adult women (326±45 nm). Thus it is important to consider the age and sex of the patient when evaluating GBM width.Each EM laboratory should establish a consistent technique for measuring GBM thickness, and determine its own means and standard deviations for GBM width, to make comparisons with published data meaningful.
The definition of “thin” GBM in the literature is imprecise, in part because of the use of different techniques to measure GBM width. When an EM laboratory’s normal values for GBM width are similar to those of Steffes et al. a cut-off value of 250 nm will accurately separate adults with normal GBM from those with thin GBM. Where the normal values are significantly higher, a cut-off value of 330 nm is appropriate. For children, the cut-off is in the range of 200–250 nm (250 nm is within 2SD of the mean at age 11). It is useful to note that the intraglomerular variability in GBM width is small in thin GBM disease. Marked variability in GBM width within a glomerulus, in a patient with persistent microhematuria, should raise suspicion of Alport syndrome, although focal lamina densa splitting has been described in TBMN.
Genetics and Pathophysiology
TBMN is usually transmitted as an autosomal dominant condition. A negative family history may not be reliable, since patients eventually diagnosed as having TBMN are frequently unaware that they have relatives with hematuria. The first clue to the genetic basis of TBMN was provided by Lemmink and coworkers, who described a large Dutch TBMN kindred in which the disease locus was first mapped to chromosome 2 in the region of the COL4A3 and COL4A4 genes, and then affected individuals were found to be heterozygous for a missense mutation in COL4A4 . Since this landmark work, heterozygous mutations in COL4A3 or COL4A4 have been described in numerous TBMN families. However, linkage to the COL4A3 and COL4A4 genes has been excluded in other TBMN families, indicating that TBMN is a genetically heterogeneous condition.
To date, immunohistologic studies of type IV collagen in GBM of patients with TBMN or thin GBM disease have failed to uncover any abnormalities in the distribution of any of the six chains. However, it is tempting to speculate that in those kindreds with TBMN due to a heterozygous COL4A3 or COL4A4 mutation, a 50% reduction in the GBM α3(IV)–α4(IV)–α5(IV) network results in lamina densa and GBM thinning and fragility. This reduction is presumably insufficient to trigger the pathophysiologic events that produce the lamina densa splitting and GBM thickening characteristic of Alport syndrome (AS). Immunohistologic evaluation of GBM type IV collagen may be useful in the differentiation of TBMN and AS (see below).
Diagnosis
Patients with persistent, isolated microhematuria are usually candidates for kidney biopsy, once structural urinary tract abnormalities, urinary tract stones and tumors are excluded. If the patient’s family history indicates autosomal dominant transmission of hematuria, and there is no history of chronic renal failure, a presumptive diagnosis of TBMN can often be made without kidney biopsy. When family history is negative or unknown, or there are atypical coexisting features such as proteinuria or deafness, renal biopsy may be extremely informative. A finding of thin GBM may be further characterized by examining the distribution of type IV collagen α chains in the kidney. Normal distribution of these chains provides supportive, although not conclusive, evidence for a diagnosis of TBMN (see also preceding section on Alport syndrome).
Treatment
Patients who are given a diagnosis of TBMN should be reassured, but not lost to follow-up examination. The risk of chronic renal insufficiency appears to be small but real. Reasonable follow-up would include urinalysis and measurement of blood pressure and renal function every one to two years.
Hereditary Angiopathy with Nephropathy, Aneurysms and Cramps (Hanac Syndrome)
Missense mutations in the COL4A1 gene encoding the α1 chain of type IV collagen have been associated with autosomal dominant transmission of an angiopathy characterized by nephropathy, aneurysms and muscle cramps in several families. Renal symptoms include hematuria, renal insufficiency and renal cysts. Renal biopsies showed no abnormalities of glomerular basement membrane structure or type IV collagen expression, although basement membranes of Bowman’s capsules, tubules and interstitial capillaries showed irregular thickening and lamellation.
Pierson Syndrome
Pierson and colleagues reported familial cases of infantile nephrotic syndrome and distinct ocular abnormalities. The ocular abnormalities were marked by enlargement of the globe (buphthalmos) and reduction in the size and reactivity of the pupils (microcoria). All affected infants died within the first year of life.
Zenker and colleagues reported on consanguineous families with 11 children demonstrating similar clinical features. Several of these children were identified by fetal ultrasound, variably demonstrating kidney enlargement and hyperechogenicity, oligohydramnios, placental enlargement and/or pulmonary hypoplasia. Infants surviving to term had congenital nephrotic syndrome, with kidney biopsy or autopsy specimens revealing diffuse mesangial sclerosis.These children also had ocular globe enlargement and microcoria, with sporadic findings of cataract, posterior rupture of the lens capsule (lenticonus) and retinal abnormalities. In additional case reports, affected children had muscular hypotonia, central nervous system hemangioma and genital abnormalities. Kidney biopsy specimens from these patients also demonstrated diffuse mesangial sclerosis, as well as marked GBM irregularities. None of these children survived beyond the first year of life.
Zenker and colleagues localized the Pierson syndrome gene to chromosome 3p14–p22 by homozygosity mapping. Identifying the LAMB2 gene encoding the β2 chain of basement membrane laminin as a candidate, these workers went on to sequence homozygous and compound heterozygous mutations in five families, including two nonsense mutations, three frameshift mutations and one missense mutation.In one patient, a homozygous nonsense mutation of the LAMB2 gene was associated with loss of β2 laminin from the GBM.
The β2 chain of laminin is one of four β chains of basement membrane laminin. Individual laminins are heterotrimeric glycoproteins made up of variable α, β and γ chains, combining in 1:1:1 stoichiometric proportions to form cruciform structural subunits. In turn, laminin subunits self-assemble and generate macromolecular networks within basement membranes, serving myriad roles in overall extracellular matrix organization, determination of basement membrane properties and interactions with adjacent cells. The laminin β2 chain participates in the formation of several distinct αβγ laminins. In particular, laminin-11 comprised of α5β2γ1 is upregulated during development of the kidney glomerulus, in a characteristic mid-gestational switch of laminin and type IV collagen isoforms marking GBM maturation, . Partially recapitulating Pierson syndrome, targeted deletion of the mouse Lamb2 ortholog causes congenital nephrosis, with mesangial hyperceullularity and podocyte foot process injury. Interestingly, laminin β2-deficient mice also demonstrate neuromuscular synapse abnormalities with resulting muscular weakness, a feature with correlates in Pierson syndrome.
The mechanisms by which LAMB2 mutations give rise to nephrotic syndrome and accelerated kidney disease are uncertain. Ultrastructural abnormalities in Pierson syndrome suggest disruption of GBM structural integrity. Defects in glomerular cell-GBM interactions are also likely, as laminin-11 is a ligand for integrin extracellular matrix receptors, most importantly α3β1 integrin mediating glomerular cell attachment to the GBM.
Further discussion on the laminins and their biological roles in kidney and other tissues can be found in several excellent reviews.
Storage Disorders
Fabry Disease
Fabry disease is an X-linked disorder caused by deficiency of the lysosomal hydrolase enzyme α-galactosidase A (EC 3.2.1.22).Deficient α-galactosidase A (α-Gal A) activity results in accumulation of glycosphingolipid (globotrioacylceramide, or Gb3) in blood vessels and various organs leading to the clinical manifestations of the disease, including chronic renal failure. Also known as Anderson-Fabry disease or angiokeratoma corporis diffusum universale, Fabry disease was first described in 1898 in separate reports by Anderson in England and Fabry in Germany. Although the incidence of Fabry disease is approximately 1:40,000, making it a rare cause of renal failure, much is known about the genetic pathophysiology of the disorder. Most importantly Fabry disease has the potential to be effectively treated by enzyme replacement.
Clinical Features
Since Fabry disease is an X-linked disorder, severe clinical manifestations occur in hemizygous males while heterozygous females exhibit a variable but typically less severe course. However, there is increasing recognition of disease-related morbidity in heterozygous females. Data from the Fabry Registry indicated that about 70% of heterozygous females have symptoms of Fabry disease. Although median onset of symptoms was 13 years, and over 80% had a positive family history of Fabry disease, median age of diagnosis was 31 years. Major cerebrovascular, cardiac or renal events occurred in 20% at a median age of 46 years, and proteinuria and reduced glomerular filtration rate were common. These findings illustrate the importance of regular monitoring in females as well as males with Fabry disease.
The clinical features of Fabry disease are summarized in Table 84.6 . In hemizygotes (affected males) the initial features of the disease are seen in childhood and early adolescence and consist of paresthesias and pain in the hands and feet with episodic pain crises. The course of the disease is variable but usually leads to end-stage renal disease in the third to sixth decade. Myocardial or cerebral infarctions are typically terminal events
Skin | Angiokeratomas |
---|---|
Nervous system | Painful neuropathy |
Acroparesthesias | |
Hypohidrosis | |
Stroke | |
Dementia | |
Cardiac | Hypertrophy |
Coronary artery disease | |
Cardiomyopathy | |
Renal | Proteinuria |
Renal failure | |
Eye | Corneal and lenticular opacities |
The classic skin lesion, angiokeratoma corporis diffusum universale, consists of macules or maculopapules, cherry red to black in color and 1–3 mm in diameter. These lesions first appear in adolescence particularly in the scrotal area, lower back and trunk. There may be up to several dozen lesions. Other skin manifestations include palmar erythema, splinter hemorrhages, and conjunctival or buccal mucosal telangiectasias. One of the most profound and debilitating aspects of the disease is peripheral nerve involvement leading to painful crises, acroparesthesias, and hypohidrosis. Central nervous system involvement includes cerebral infarctions and dementia.Corneal and lenticular opacities occur in all hemizygotes and most heterozygotes.
The most common cardiac manifestation in Fabry disease is coronary artery disease but hypertrophy, congestive heart failure, valvular lesions, and arrythmias are common. A form of the disease limited to the heart has been described and is characterized predominantly by cardiac hypertrophy. These patients often have mutations that result in detectable amounts of enzyme. In fact, among 230 consecutive male patients presenting with left ventricular hypertrophy 3% had low plasma α-Gal A activity and may represent cardiac limited variants of the disease.
The earliest renal manifestation is a concentrating defect.Proteinuria, typically in the range of 0.5 to 2 g daily, is first detected in young adulthood. Nephrotic-range proteinuria is unusual but may be seen. The urinary sediment is most often bland although some patients exhibit microscopic hematuria. There is increased urinary glycosphingolipid, and urinary myelin bodies, both free and within tubular cells, may be found. Classic Maltese crosses can be seen when the urine sediment is examined with a polarizing microscope. Fabry disease has been reported in association with IgA nephropathy and SLE.
End-stage renal disease typically occurs in the third to sixth decade but has been reported in patients less than 20 years old. The clinical course of the renal failure has been described by several investigators.Donati and colleagues followed 12 patients with end-stage renal disease. Eight patients were transplanted and all survived with the longest patient being 66 months post transplant. Three dialysis patients died 9, 11 and 12 months after starting dialysis prompting these investigators to conclude the prognosis was better after transplantation. Nissenson and colleagues described 17 patients (including one woman) with Fabry disease who underwent treatment for end-stage renal disease. Eighty three percent of the patients survived to 33 months and 41% were transplanted.
Pathology
Intracellular glycosphingolipid accumulation is found in most structures of the kidney including glomerular epithelial, endothelial and mesangial cells, tubular epithelial cells, interstitial cells, and blood vessels including endothelial, perithelial and smooth muscle cells. During routine processing of paraffin-embedded sections lipids are dissolved by the xylene so that the cells which contained them look like foam cells with empty vacuoles ( Figure 84.4 ). With fresh or fixed-frozen tissue lipids can be identified with fat stains such as Sudan III or oil red O. The glomerular epithelial cells are most prominently affected in the kidney.These cells are filled with small, uniformly sized vacuoles, giving them a foamy appearance ( Figure 84.4 ). Vacuoles are often present in parietal epithelial cells but are not commonly seen in endothelial or mesangial cells. In the later stages of the disease the glomeruli become sclerotic.

Vacuolization of tubular cells is most prominent in the loop of Henle and distal convoluted tubule and is rarely seen in the proximal tubule. Most blood vessels are involved with vacuoles present in endothelium, smooth muscle cells and perithelial cells.In later stages insudative arteriolar changes are observed.
Immunofluorescence is negative except in advanced glomerular sclerosis where non-specific uptake of immunoglobulin or complement can be seen. Glycosphingolipid can be detected with antibodies to trihexosylceramide.
The characteristic lesion of Fabry disease is seen on electron microscopy (Figure 84.8). Stacks or whorls of dense inclusions ranging in diameter from 0.3 to 10 µm (average 3 µm) are found in lysosomes of affected cells. Terms such as onion skin or myelin figures have been used to describe the concentric dense layers of glycosphingolipid deposits.The deposits can also appear as parallel arrays (“zebra bodies”). Cells that appear normal on light microscopy can have inclusions.In the glomerulus, deposits are present in every cell type but are less numerous and smaller in endothelial and mesangial cells. Heterozygous females have similar but fewer inclusions. Other intracellular organelles appear normal. Foot process fusion occurs with heavy proteinuria.In the later stages of the disease the glomerular basement membrane becomes wrinkled and thickened and mesangial interposition may occur. These changes are suggestive of glomerular ischemia. Typical inclusions may be present in Bowman’s space and tubular lumena, and cells containing glycosphingolipid may be found in the urine and can be used for making the diagnosis of Fabry disease.
Genetics and Pathophysiology
The α-Gal A protein has a molecular weight of approximately 50 kDa. It is a homodimeric glycoprotein containing 5 to 15% oligosaccharide chains.Processing includes cleavage of the signal peptide and carbohydrate modifications resulting in the mature 100 kDa enzyme.The mature protein then moves to its site of action in the lysosome. The protein has been crystallized and analyzed in more detail.
α-Gal A cDNA was first isolated in 1986. The coding sequence comprises 1290 bp and codes for a protein of 429 amino acids including a 31 amino acid signal peptide. There are four possible N-glycosylation sites, three of which are used. The α-Gal A gene is located on the long arm of the X chromosome at Xq22.1. The gene is 12 kb in size and contains 7 exons ranging in size from 92 to 291 base pairs. The sequence for the 5’ untranslated region, the signal peptide and the first 33 amino acids of the mature enzyme are contained in exon 1. The gene is Alu -rich, containing 12 Alu repetitive elements each containing 300 nucleotides that comprise 30% of the gene. Polymorphisms of the α-Gal A gene have been described but are rare. These polymorphisms along with other highly polymorphic markers near the α-Gal A gene can be used for diagnosis and carrier detection.
Almost all unrelated families with Fabry disease have different mutations. The majority of the mutations are single base substitutions in the coding region with two thirds occurring in exons 5 through 7. Other mutations include gene rearrangements such as duplications, deletions, insertions, and RNA processing defects.
Deficiency of α-Gal A leads to progressive intracellular accumulation of neutral glycosphingolipids, particularly those with α-galactosyl moieties, the most abundant of which is globotriaosylceramide (Gb3). Glycosphingolipids are normal constituents of the plasma membrane, the membranes of intracellular organelles, and circulate in association with apolipoproteins, particularly low density lipoprotein. The glycosphingolipids that accumulate in Fabry disease are identical to those found in normal tissue. All tissues except red blood cells accumulate Gb3 with the highest concentrations found in the diseased kidney.
Accumulation of glycosphingolipids, particularly in the CNS and cornea, results from retention of endogenously metabolized glycosphingolipid. The presence of glycosphingolipid in vascular endothelium and smooth muscle may be due to uptake of circulating Gb3. Vascular pathology results from the swollen endothelium narrowing or occluding the vascular lumen.In addition, the endothelium likely serves as an initiator of vascular thrombosis. Whether the renal pathology is entirely secondary to vascular disease or due to glomerular and/or tubular epithelial cell toxicity is unclear. The most prominent early lesion in the kidney is swelling and vacuolization of visceral epithelial cells which likely is the underlying basis for the proteinuria and may be an important factor in the development of glomerular sclerosis.
Classically affected hemizygotes have a large variety of mutations. Patients with mutations that maintain some residual α-Gal A activity tend to have milder phenotypes. Examples include patients with disease confined predominantly to the heart, who most often have missense mutations and express low levels of α-Gal A activity.
Mice in which the α-Gal A gene has been deleted by gene targeting techniques completely lack α-Gal A activity as expected. No clinical phenotype is detectable at 10 weeks of age. However, ultrastructural and histochemical studies, and lipid analysis demonstrate the classic lamellar inclusions in the kidney and accumulation of Gb3 in the liver, kidneys and in cultured fibroblasts. In the kidney the lipid inclusions are first seen in renal tubular cells. Enzyme activity can be restored in cultured embryonic fibroblasts of the deficient mice by transducing the cells with a retrovirus containing the α-Gal A gene, resulting in decreased accumulation of Gb3 in the fibroblasts. Infusion of transduced bone marrow cells corrects the enzyme deficiency and reduces glycosphingolipid stores in α-Gal A-deficient mice.
Diagnosis
Diagnosis of the hemizygote patient can usually be made on clinical grounds with the additional information from slit lamp examination of the eye. The diagnosis should be confirmed by demonstrating decreased to absent α-Gal A activity in serum, leukocytes, cultured skin fibroblasts or biopsied tissue. Atypical variants may have enzyme activity up to 35% of normal. A dissociation may also exist between serum and tissue enzyme activity.
Heterozygous females have intermediate levels of α-Gal A activity but values may be in the low normal range making measurement of enzyme activity an insensitive way of diagnosing carriers. Alternatives include careful slit lamp eye exam, measuring urinary ceramide digalactoside and trihexoside, or by molecular techniques using restriction fragment length polymorphisms of either the α-Gal A gene or closely linked markers on the X-chromosome. Identification of carriers is particularly relevant when family members are being considered as living kidney donors. Measurement of chorionic villus enzyme level or the enzyme level in cultured amniotic cells is possible.
Treatment
Until recently, clinicians could offer little beyond palliative, symptomatic care to patients with Fabry disease, including carbamazepine and gabapentin, or narcotics, for neuropathic pain, antihypertensive therapy and platelet antagonists. However, the landscape of Fabry therapy has been transformed over the past several years by the introduction of enzyme replacement therapy using recombinant human α-Gal A (agalsidase).
Studies conducted in the 1970s showed that injection of α-Gal A purified from human plasma resulted in transient reduction in plasma Gb3. Production of sufficient enzyme for clinical trials was not possible until the advent of recombinant DNA technology. In 2001, Ioannou and colleagues described pre-clinical studies in α-Gal A-deficient mice showing that recombinant human enzyme lowered tissue and plasma Gb3, providing the rationale for human treatment trials.
Phase I/II clinical trials demonstrated that agalsidase produced dose-dependent reductions in plasma, tissue and urine Gb3 in Fabry patients. Randomized clinical trials showed that agalsidase administration over 5–6 months resulted in reduced plasma and urine Gb3, amelioration of neuropathic pain, enhanced quality of life, clearing of Gb3 deposits from kidney, heart and skin, and improved cerebral blood flow. A multicenter longitudinal study showed that algasidase stabilized renal function in patients with mild to moderate renal impairment at baseline, and reduced left ventricular mass in those with left ventricular hypertrophy at baseline, over one to two years of treatment. Agalsidase therapy has been recommended for all affected males and symptomatic carrier females.
Long-term therapeutic outcomes of agalsidase therapy are also promising. Left ventricular mass index remained stable in patients without baseline ventricular hypertrophy and decreased significantly in those with ventricular hypertrophy at the onset of therapy. Pain severity decreased, and quality of life indicators increased, with therapy. Glomerular filtration rate was stabilized by therapy. Trials testing agalsidase therapy against placebo have shown reduced rate of loss of renal function in agalsidase-treated patients, although no effect on proteinuria was observed.
A problem that may affect the long-term efficacy of agalsidase treatment is development of neutralizing antibodies to the enzyme. Linthorst and colleagues found that 11/16 Fabry males developed high titers of neutralizing IgG antibodies to α-Gal A. After six months of treatment, antibody-negative patients had significantly lower urine Gb3 levels compared to antibody-positive patients, suggesting that the antibodies interfered with renal tubular Gb3 clearance. However, the impact of α-Gal A antibodies on the efficacy of agalsidase treatment remains to be determined. A recent analysis found no correlation between anti- α-Gal antibody titers and rate of change in glomerular filtration rate during treatment.
Options for managing end-stage renal failure in Fabry patients include dialysis and transplantation. Initial reports of kidney transplantation for Fabry disease suggested that the allograft might serve to correct the underlying metabolic defect. However, kidney transplantation does not improve the systemic manifestations of the disease. Graft and patient survival rates for patients with Fabry disease are similar to those in patients with other diagnoses. Glycosphingolipid can accumulate in the transplanted kidney but the deposits are detectable only by electron microscopy predominantly in capillary endothelial cells. Loss of the allograft from recurrent disease has not been described. Transplanting the kidney from a heterozygote can result in recurrent disease making donor screening important. Renal allograft recipients with Fabry disease are candidates for agalsidase treatment.
Fabry Disease in Childhood
The signs and symptoms of Fabry disease, particularly pain crises, acroparesthesias, angiokeratomas and corneal opacities, typically have their onset in childhood, although a specific diagnosis is frequently delayed until well into adulthood. Albuminuria and characteristic renal histological abnormalities have been described in children and adolescents with Fabry disease. Agalsidase therapy in a small number of pediatric patients was associated with clearing of GL-3 from dermal capillary endothelium, and mild or moderate infusion-associated reactions. In another study, affected children treated with agalsidase resulted in reduced plasma and urine GL-3 levels and decreased pain severity.
Other Storage Disorders Associated with Glomerular Dysfunction
Glomerular dysfunction may be detected in patients with various inherited storage diseases, associated with accumulation of the storage material in glomerular cells, mesangium or GBM (see Table 84.1 ). Deficiency of the enzyme lecithin-cholesterol acyltransferase (LCAT) prevents esterification of plasma cholesterol and thus interferes with reverse cholesterol transport from tissues to the liver. The accumulation of free cholesterol in tissues results in a variety of clinical abnormalities, including proteinuria that frequently progresses to ESRD. Light microscopic features of the kidney in LCAT deficiency include mesangial expansion and glomerular capillary wall thickening. The ultrastructural findings are distinctive, consisting of numerous, irregular lucencies in the mesangium and GBM that contain dense granular or membrane-like structures. These lesions regularly recur in renal transplants.
Sialidosis results from deficiency of sialidase, which removes terminal sialic acid residues from sialyl-oligosaccharides released during glycoprotein degradation. Consequently, sialic acid-rich material accumulates in various tissues.Renal involvement, manifested by proteinuria, occurs in type II, or dysmorphic-type, sialidosis. Light and electron microscopy of renal biopsy specimens shows massively swollen podocytes, due to the presence of innumerable thin-walled cytoplasmic vesicles. Vesicles may also be seen in mesangial, endothelial and tubular epithelial cells. Material within these vesicles binds the lectin wheat germ agglutinin, confirming the presence of terminal sialic acid moieties. Podocyte detachment has been observed in sialidosis and may be pathogenetically related to the proteinuria. Prominent accumulation of storage material in podocytes has also been described in I-cell disease, in which a mixture of mucopolysaccharides, lipids, and oligosaccharides accumulates, and in Hurler syndrome, in which the stored material is mucopolysaccharide.
Although renal disease is not unusual in older patients with glycogen storage disease type 1 (GSD1) due to glucose-6-phosphatase deficiency, it is not clear that the pathologic changes in the kidney arise from storage of glycogen. It has been proposed that proteinuria and glomerulosclerosis in patients with GSD1 result from hyperfiltration injury.
Disorders of the Podocyte Slit Diaphragm
Congenital Nephrotic Syndrome of the Finnish Type (CNF)
Clinical Features
This autosomal recessive condition is the predominant cause of massive proteinuria in the first month of life. While Finland appears to have the world’s highest incidence of CNF (about 1.2 cases/10,000 pregnancies), cases in children of non-Finnish ancestry have been reported from every part of the globe. Fetuses with CNF exhibit proteinuria in utero , manifested by elevated levels of α-fetoprotein in the amniotic fluid. Edema frequently appears during the first week of life. Cerebral palsy-like neurological disease has been described in a subset of patients, although the mechanisms of association with CNF are unclear. Infants with CNF eventually develop renal failure, however historically the effects of malnutrition, overwhelming infection and thrombosis frequently resulted in early death, prior to the development of ESRD. Today, with aggressive therapy these children can survive infancy and grow sufficiently to undergo renal transplantation, with excellent rates of patient and graft survival. In recent years, the clinical spectrum of glomerular disease due to NPHS1 mutations (see below) has expanded to include childhood- and adult-onset steroid-resistant nephrotic syndrome. Furthermore, the heterozygous state may, in some cases, contribute to nephrotic syndrome occurring sporadically.
Pathology
Nephrogenesis appears to proceed normally in kidneys of fetuses with CNF. However, histological abnormalities such as focal mesangial hypercellularity, dilatation of Bowman’s spaces and renal tubules, and visceral epithelial cell foot process fusion and microvillous transformation, are not unusual in these kidneys. Postnatally, early histological findings include mesangial hypercellularity and matrix expansion. Bowman’s spaces and proximal tubules may become cystically dilated. After six months, progressive glomerulosclerosis, tubular atrophy and interstitial fibrosis are evident. Immunofluorescence microscopy is typically unremarkable, except for albumin reabsorption droplets in tubular epithelial cells. Electron microscopy shows visceral epithelial foot process fusion and microvillous transformation, while the GBM typically appears to be structurally normal.Variants on the classical histology have been described.
Genetics and Pathophysiology
For many years efforts to understand the mechanism of proteinuria in CNF focused on the composition on the GBM, particularly proteins contributing to the negative charge of GBM, such as heparan sulfate proteoglycan. However, the results of genetic mapping studies carried out in the 1990s, culminating in positional cloning of the NPHS1 gene encoding nephrin, brought about a dramatic shift in focus to the podocyte slit diaphragm.
Nephrin, an integral membrane protein with a single transmembrane domain and 8 extracellular immunoglobulin-like domains, is expressed at the slit diaphragms between glomerular visceral epithelial cell (podocyte) foot processes ; it is the cardinal member of the nephrin family of proteins. The critical role of nephrin in maintaining glomerular permselectivity was confirmed in nephrin-knockout mice, which develop massive proteinuria in utero and exhibit absence of podocyte foot processes and slit diaphragms. Nephrin strands from adjacent foot processes appear to interact to form pores in the slit diaphragm. Nephrin interacts with several proteins, including podocin, CD2-associated protein (CD2-AP) and the related adaptor protein CIN85/Ruk L , ion channels, junctional proteins and the nephrin analogs Neph1 and Neph2. The cytoplasmic tail of nephrin undergoes phosphorylation at several tyrosine residues in a tightly regulated process involving Src family protein kinases. Covalent modifications of nephrin and interactions with its binding partners have been implicated in a variety of slit diaphragm functions including barrier formation, turnover, cell signaling and cytoskeletal regulation. Further studies in model systems have shown that nephrin can activate transcription, and that this function is enhanced by the interaction of podocin with the tyrosine-phosphorylated cytoplasmic tail of nephrin.
Disease-causing mutations have been identified throughout the NPHS1 gene, attesting to the functional importance of both the intracellular and extracellular domains of the protein. Two mutations in NPHS1 , Fin major (nt121delCT) and Fin minor (R1109X), were found to account for >90% of Finnish NPHS1 patients. A variety of other mutations in NPHS1 (>140) have been found in non-Finnish patients. Milder forms of kidney disease may occur in some homozygotes and compound heterozygotes in whom at least one mutation is “mild,” resulting in a protein that traffics to the cell membrane and retains partial function.
Diagnosis
NPHS1 mutations are the predominant, but not sole, cause of nephrotic syndrome in the first month and account for an estimated 40 to 60% of nephrotic syndrome cases in the first three months of life. Some infants with clinical CNF have mutations in podocin, the product of the NPHS2 locus, and a congenital form of focal segmental glomerulosclerosis (FSGS) has been described in patients with NPHS1 mutations in conjunction with mutations in NPHS2 (see below). Other conditions that may present with early nephrotic syndrome include congenital infections, renal disorders that typically present in late infancy and childhood, such as minimal change nephrotic syndrome and FSGS, and diffuse mesangial sclerosis (DMS), with or without other features of Denys-Drash syndrome (see below). Features consistent with a diagnosis of CNF include placental enlargement (placental weight greater than 25% of birth weight), positive family history of early nephrotic syndrome and intrauterine proteinuria, indicated by elevated amniotic fluid levels of α-fetoprotein. Hypoalbuminemia is generally severe at presentation (<1 gm/dL).Infants with CNF characteristically maintain a normal GFR through at least the first six months of life, so rapid deterioration of renal function should suggest another diagnosis. Renal biopsy after three to six months will reveal the typical histology of CNF which, while not necessarily specific, differs substantially from the histology of DMS, FSGS and nephropathies associated with congenital infection.The cloning of NPHS1 and NPHS2 and the availability of commercial mutation analysis have enhanced diagnostic precision in infants with nephrotic syndrome. Because of the clinical and histological overlap between NPHS1 – and NPHS2 -related disease, molecular diagnostic approaches should incorporate analysis of both genes. Screening for less frequent mutations in the WT1 , LAMB2 and PLCE1 genes can be considered in cases in which NPHS1 / NPHS2 analysis is non-diagnostic or kidney biopsy demonstrates DMS.
Prenatal diagnosis of CNF due to NPHS1 mutations is complex. Fetuses with CNF due to NPHS1 mutations exhibit elevated amniotic fluid α-fetoprotein (AFP) levels during the second trimester. However, amniotic fluid AFP may be elevated in other conditions, such as neural tube defects, and there is overlap between the amniotic fluid AFP levels of fetuses with CNF and healthy carriers of NPHS1 mutations. Prenatal diagnosis of CNF is greatly facilitated if the disease-causing mutation in the family is known. If the mutation is not known, sequencing of the entire NPHS1 gene within the required time interval may not be possible. In this situation, the finding of elevated amniotic fluid AFP should prompt a fetal ultrasound to exclude a neural tube defect, followed by serial measurement of amniotic fluid AFP during the second trimester. In fetuses with CNF AFP levels will remain elevated, while levels will decrease in healthy carriers of NPHS1 mutations.
Treatment
With appropriate management, infants with CNF can thrive sufficiently to allow successful renal transplantation. However, such treatment is complex, requiring meticulous attention to nutrition, albumin replacement, correction of hypothyroidism, prophylaxis and prompt recognition and treatment of bacterial sepsis, and prevention of thrombosis. Elective bilateral nephrectomies are performed once the child’s real weight reaches about 7 kg. A period of hemo- or peritoneal dialysis for 6–8 weeks allows for optimization of nutrition and normalization of plasma proteins, and then the child undergoes transplantation. While this approach has resulted in excellent rates of patient and graft survival, others have advocated unilateral nephrectomy combined with captopril and indomethacin therapy and later renal transplantation.
Autosomal Recessive Steroid-Resistant Nephrotic Syndrome
Clinical Features
Fuchshuber and colleagues first classified familial autosomal recessive SRNS as a subgroup of idiopathic nephrotic syndrome for genetic mapping studies that culminated in the identification of the NPHS2 gene and its gene product podocin. The defining clinical features included: familial occurrence, childhood-onset of nephrotic syndrome, resistance to steroid therapy, rapid progression to ESRD and absence of recurrence after kidney transplantation. It has since become evident that SRNS due to NPHS2 mutations is the single most common cause of nephrotic syndrome during the first year of life, accounting for 30 to 40% of cases. Furthermore, 10 to 30% of patients presenting as sporadic cases of childhood-onset SRNS represent homozygous or compound heterozygous offspring of unaffected heterozygous parents, with estimated frequencies dependent on racial and ethnic background.
Among large cohorts of childhood-onset SRNS, age at presentation has been reported to range from <months to 24 years, with estimated means of 3 to 6 years. Age at ESRD has been reported to range from 4 to >27 years, with estimate means of 8 to 10 years. Patients with SRNS and homozygous or compound heterozygous NPHS2 mutations appear to suffer accelerated progression of disease, in comparison to patients with SRNS but without these mutations.
Variable clinical presentations associated with pathogenic NPHS2 mutations are well described. There are several reports of infantile SRNS demonstrating clinical overlap with CNF; these cases result from NPHS2 mutations that may be associated with digenic inheritance of NPHS1 mutations. Familial forms of adult-onset focal glomerulosclerosis also result from NPHS2 mutations. By comparison to childhood-onset cases, adult-onset cases demonstrate variability in penetrance and clinical course, and association with compound NPHS2 mutations including common polymorphisms (see below). Viewed in this light, childhood-onset SRNS may represent the most severe manifestation of NPHS2 -linked glomerular disease, modified by other as yet incompletely undefined genetic and environmental factors. The overall contribution of NPHS2 mutations to sporadic cases of focal glomerulosclerosis is uncertain but almost certainly lower in adults than children.
Extrarenal manifestations of SRNS associated with NPHS2 mutations have not been described. Indeed, multisystem findings suggest the likelihood of an alternative primary diagnosis. Additional clinical features of familial SRNS are described below.
Pathology
In familial SRNS, more than 60% of kidney biopsy specimens demonstrate focal glomeruloscerosis as the principal finding. Variable findings include mesangial expansion, and IgM, IgG and/or complement component deposition. Remaining biopsy specimens generally demonstrate minimal change, although other histological findings have been described.
Genetics and Pathophysiology
Fuchschuber and colleagues first established linkage of a gene for autosomal recessive SRNS in eight families to chromosome 1q-25-q31. Boute and colleagues later reported identification of the NPHS2 gene and pathogenic mutations in these and several additional families. The NPHS2 gene spans eight exons over 25 kb, producing a 1853-base pair transcript and a 383-amino acid protein, termed podocin.
Podocin is a member of the prohibitin homology (PHB)-domain family, a family of membrane proteins that associate preferentially with cholesterol-rich lipid rafts in which they play specialized roles. Podocin contains a 104-amino acid amino-terminal intracellular domain, a 17-amino acid amino-terminal-adjacent intramembranous domain and a 262-amino acid carboxy-terminal intracellular domain; the intramembranous domain mediates attachment to the inner leaflet of the cell membrane and thus confers a hairpin structure.
Several important aspects of podocin function have recently been elucidated. Podocin is expressed in the kidney exclusively, within podocyte foot process plasma membranes at sites of insertion for slit diaphragms. Podocin forms homo-oligomers and interacts with nephrin and other slit diaphragm proteins within specialized plasma membrane domains, suggesting roles in slit diaphragm formation, maintenance and/or signaling. Like other PHB-domain proteins, podocin binding to cholesterol is required for important cellular effects: in particular, podocin regulates the activity of TRPC6 ion channels, calcium-permeable cation channels associated with the slit diaphragm and implicated themselves in inherited glomerular disease (see below). The phenotypes of podocin-mutant mice have confirmed the importance of podocin in slit diaphragm development and maintenance.
To date, over 100 NPHS2 mutations have been identified. The majority are missense mutations, with the remainder nonsense, frameshift and splicing mutations. Large genetic rearrangements have not been described. NPHS2 mutations are distributed throughout the gene, with genotype-phenotype correlations suggesting that protein-truncating mutations correlate with earlier onset of disease. Disease-associated amino acid-substitutions may alter expression levels and/or intracellular trafficking and produce disturbances in homo-oligomerization, protein-protein interactions and/or recruitment of nephrin and other slit diaphragm protiens to specialized plasma membrane domains. The R229Q variant of podocin has attracted particular attention. Present in various ethnic populations at a frequency of 1-6%, this variant may contribute to disease pathogenesis, most convincingly in the presence of additional genetic mutations.
Diagnosis
SRNS is a clinicopathological entity diagnosed a posteriori . A positive family history can indicate a genetic basis for SRNS.The role of NPHS2 mutational analysis in the diagnosis and management of nephrotic syndrome is evolving. On the basis of available data, a significant percentage of children and an unknown but much smaller percentage of adults will have NPHS2 mutations at first presentation with nephrotic syndrome, suggesting that genetic testing may not be useful in adults. Nonetheless, identification of NPHS2 mutations can minimize patient exposure to ineffective and potentially harmful treatments.Several investigators have advocated genetic testing in familial cases of nephrotic syndrome or concomitant with first-time empiric steroid therapy in non-familial cases. Others have argued that knowledge of genetic status serve as an entry criterion for clinical trials. NPHS2 testing may reveal polymorphisms of uncertain clinical significance, posing challenges in diagnosis and management.
Treatment
Resistance to steroid therapy is a diagnostic feature of SRNS. Criteria for steroid resistance itself have been outlined. Importantly, retrospective studies have now confirmed steroid resistance in large groups of patients with homozygous or compound heterozygous NPHS2 mutations. Partial response patterns with steroid-dependence have also been reported for a small number of patients with heterozygous NPHS2 mutations.
There are no established treatments for familial SRNS. In retrospective analyses of small groups of patients with NPHS2 mutations, cytotoxic agents have not been effective. Reports on the use of cyclosporine A are limited.
Kidney transplantation is the preferred treatment for patients near or at ESRD. Recurrence of proteinuria after kidney transplantation for familial SRNS is infrequent. Weber and colleagues reported the recurrence of proteinuria in only one of 32 patients with pathogenic NPHS2 mutations, and Ruf and colleagues in only two of 24 patients; in none of these cases were biopsy findings reported. By contrast, Ruf and colleagues reported recurrence of proteinuria in seven of 20 patients (35%) with SRNS but without NPHS2 mutations. In a contrasting series of reports, Bertelli, Caridi and colleagues reported recurrence of proteinuria in patients with NPHS2 mutations comparable in frequency to that in patients with SRNS but without NPHS2 mutations. However, several of these patients were NPHS2 heterozygotes with polymorphisms of unclear significance, raising uncertainty regarding the primary diagnosis. In addition, the majority of patients classified with recurrence of proteinuria underwent empiric treatment for recurrent primary disease without report of biopsy findings.
Winn and colleagues have emphasized caution in selecting first-degree relatives of patients with familial focal glomerulosclerosis as donors for kidney transplantation, suggesting genetic risks for poor outcome in donor and recipient. Outcomes of kidney transplantation in patients with homozygous or compound heterozygous NPHS2 mutations and related donors have not been reported.
Nephrotic Syndrome, Early-Onset, Type 3
Clinical Features
In 2006, Hinkes et al. described linkage by homozygosity mapping to chromosome 10q23 in seven of 26 consanguineous families with SRNS. Candidate-gene sequencing identified homozygous mutations in the PLCE1 gene encoding phospholipase Cε, thus the putative cause of NPHS3. Twelve affected children were proteinuric by four years of age and nine progressed to ESRD by age five. Remarkably, two children remitted in association with treatment by steroids or cyclosporine A. Homozygous and compound heterozygous mutations have since been identified in 30% of cases of early-onset SRNS with biopsy findings of diffuse mesangial sclerosis (DMS) ; additional case series have also suggested a close association with this specific clinicopathological entity. Disease-associated mutations have been identified in unaffected family members as well, indicating that the mutations are incompletely penetrant, perhaps variably expressive, and thus confounding on the matter of treatment-responsiveness. Extrarenal manifestations of this disorder have not been described.
Pathology
DMS with its hallmark features, described below in connection with DDS, is the pathological entity most closely associated with NPHS3.Indeed, PLCE1 mutations are those most frequently identified in DMS. Focal glomerulosclerosis has been described in the balance of NPHS3 cases undergoing biopsy.
Genetics and Pathophysiology
Human PLCE1 is a large gene spanning 34 exons over >300 kb.It encodes at least two major forms of phospholipase Cε—PLCε1a of 2,303 amino acids and PLCε1b of 1,994 amino acids—that arise by alternative splicing of exons 1 and 2 to exon 3 and thus differ at their amino termini. The PLCE1 gene was cloned by homology to other phospholipase C family members. Accordingly, phospholipase Cε serves canonical signaling pathways, hydrolyzing phosphatidyl inositol 4,5-bisphosphate to generate inositol 1,4,5-triphosphate and diacylglycerol, involved respectively in downstream mobilization of intracellular Ca 2+ stores and activation of protein kinase C Phospholipase Cε is one of 13 known human phospholipase C family members, and is alone in one of six subgroups encompassed by this family.
Like other phospholipase C family members, phospholipase Cε contains core X and Y domains constituting the catalytic domain, and upstream pleckstrin-homology and EF-hand domains. In recent years, it has become evident that phospholipase Cε is otherwise unique, integrating a multitude of signals that emanate from extra- and intracellular-sources and are mediated by major classes of heterotrimeric and monomeric G proteins. As investigated in a variety of cell culture and reconstituted systems, phospholipase Cε: (1) Binds activated Ras family members at a Ras association (RA) site downstream from the catalytic domain; these interactions direct patterns of subcellular localization and enzyme activation, and thus mediate cellular responses to receptor tyrosine kinase stimulation by ligands such as epidermal growth factor and platelet derived growth factor. (2) Binds Rho family members independent of Ras, by which dual modulation of signaling inputs is achieved. (3) Respond to stimulation via G protein-coupled receptors, through Gα subunits Gα 12/13 or Gβγ subunits, and/or monomeric G-protein signaling intermediates including Rho and Rap. (4) Catalyzes, by way of an amino-terminal CDC25 homology domain, guanine nucleotide exchange (GEF), by which GDP bound to G protein is exchanged for GTP, the activity of G proteins is regulated, and the possibilities for autoregulatory pathways expanded.
Phospholipase Cε is in podocytes, where its roles are as yet poorly understood but with preliminary indications of specialized interactions with the IQ motif-containing GTPase-activating protein 1 (IQGAP1), a multifunctional protein implicated in slit diaphragm signaling, and B-RAF, a serine/threonine kinase effecting diverse pathways of G protein signaling.
Over 20 disease-associated PLCE1 mutations have been reported, the majority homozygous, truncating mutations. Mutations span the gene and genotype-phenotype correlations are not clearly evident. Inasmuch as mutations have been identified in unaffected individuals, future considerations will include modifier genes and oligogenic inheritance patterns, mechanisms compensating for mutation effects, environmental modifiers, etc., accounting for the multifunctional nature of the protein.
Diagnosis
The frequency of PLCE1 mutations in childhood-onset SRNS is uncertain. At present, it seems prudent to reserve genetic testing for patients with biopsy findings of DMS, parental consanguinity or early-onset (age<4 years) manifestations with unrevealing NPHS1/NPHS2 testing.The frequency of identified mutations may decrease with age of onset and appears to be negligible among adults. Heterozygous mutations have been identified and remain of unclear significance.
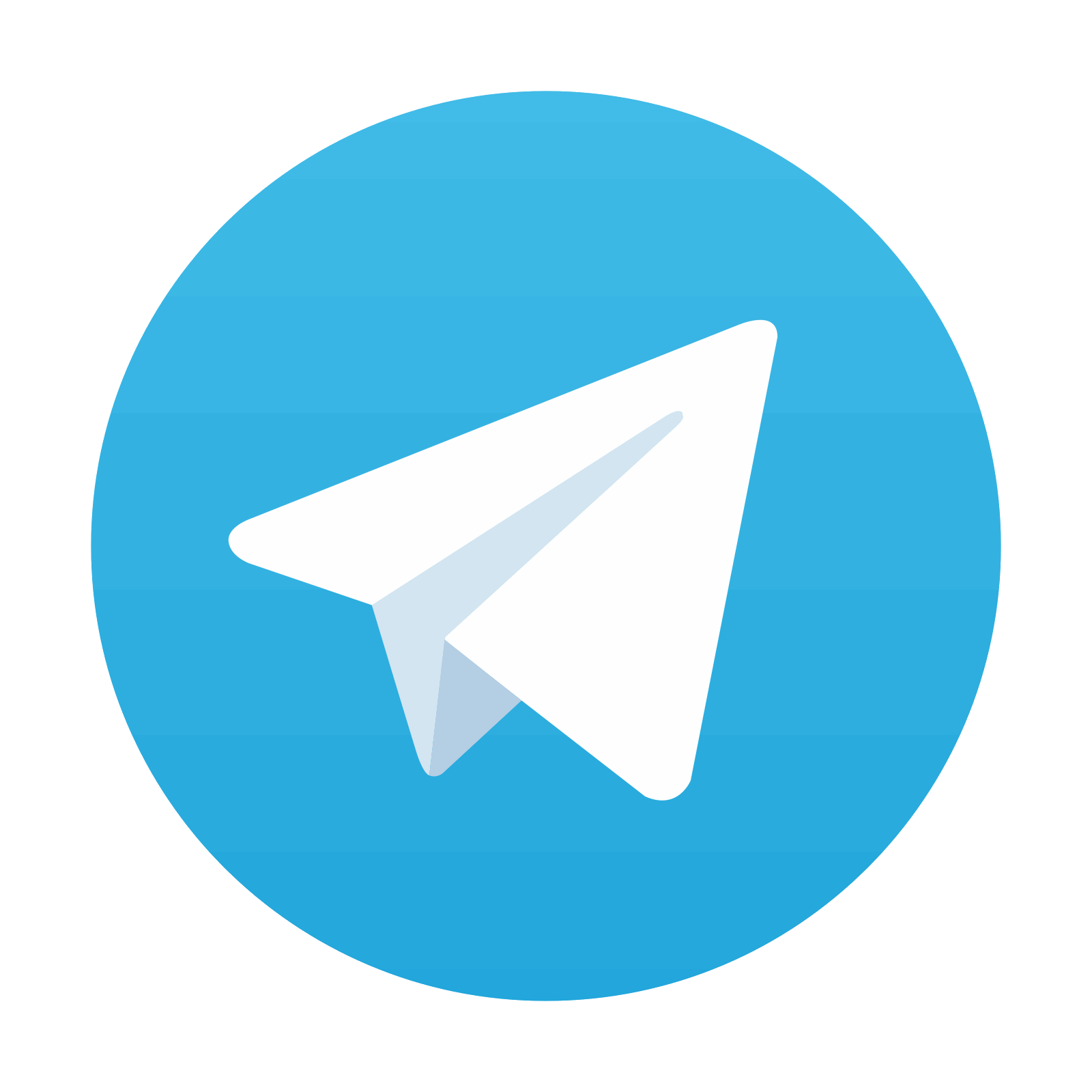
Stay updated, free articles. Join our Telegram channel

Full access? Get Clinical Tree
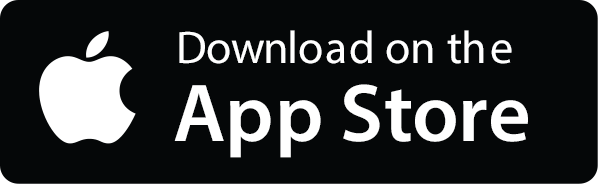
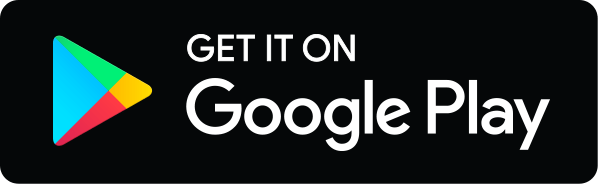