of the Na-K-ATPase pump in the skeletal and heart muscle, epithelial cells of the kidney and bladder, as well as liver and fat cells.17 This results in a series of intracellular events leading to hyperpolarization of cell membranes.17 The time course for this interaction is consistent with both an increase in enzyme activity as well as the rapid recruitment of Na-K-ATPase pumps to the cellular membrane. In contrast, chronic stimulation by insulin probably increases the total number of available pump sites. This occurs through the regulation of the Na-K-ATPase pump at the transcriptional and posttranscriptional levels by inducing the synthesis of new α and β subunits.1 McDonough and Youn,18 using a potassium clamp, have recently shown that after 10 days of potassium deprivation in rats Na-K-ATPase activity decreased by more than 50% and insulin-mediated potassium shift decreased by 94%, whereas in rats deprived of potassium for only 2 days the number of pumps did not decrease, but insulin-mediated potassium shift decreased by 80%. This would indicate that insulin resistance precedes a decrease in the number of pump expression during hypokalemia. The molecular mechanism underlying this response, however, remains poorly understood.19 Several in vitro studies, including one study in humans, have shown that insulin-driven potassium uptake by both muscle and the liver is independent of glucose uptake.15,20
with continued infusion, fell and remained lower than baseline. Other investigators have shown increased potassium tolerance in animals infused with pharmacologic doses of epinephrine22,23 despite a pancreatectomy or nephrectomy.24 Brown and coworkers25 have shown that the infusion of stress-level doses of epinephrine resulted in a decrease in serum potassium by 0.4 to 0.6 mEq per liter. Because epinephrine inhibits the renal excretion of potassium,26,27 the decline in potassium concentration is entirely accounted for by enhanced cellular potassium uptake.
for each 0.1 U change in serum pH, the serum potassium changes in the opposite direction by 0.6 mEq per liter. However, the relationship between serum potassium and serum pH is much more complex and depends on the type and severity of the acid-base disorder, the anion accompanying hydrogen, the duration of acidosis, changes in plasma bicarbonate concentration independent of changes in pH and the extent of intracellular buffering, and renal adaptation as well as hormonal changes in response to the disorder.54 In addition, in clinical settings, there are often other physiologic and pathophysiologic processes that may be present, which would affect both transcellular as well as the renal and extrarenal handling of potassium. The following generalizations should therefore be used with caution.
renal function facing sudden hyperglycemia.71 These clinical observations support an independent role of sudden osmolar shifts in the regulation of serum potassium.
1.5 to 6.0 mg per deciliter) not receiving drugs that interfered with potassium homeostasis, 55% were noted to have hyperkalemia (K+ ≥ 5.0 mEq per liter).80 Treatment with drugs that interfere with potassium handling would be expected to further increase the development of hyperkalemia (see the following). Serum potassium rises with decreasing GFR; however, it often remains within normal range with GFR above 40 mL per minute.75 In this study, the rate of hyperkalemia ([K+] > 5.0) was 17% and was primarily limited to patients with CKD stage 4 and 5. However, under certain conditions, hyperkalemia may occur in patients with mild-to-moderate renal failure (Table 72.1). In a longitudinal study of patients with CKD, hyperkalemia ([K] > 5.5] was reported in only 8% of patients and, surprisingly, hypokalemia ([K] < 4.0) was more frequently seen in 15% of patients. Hypokalemia was not related to nutrition and was most likely secondary to the use of diuretics.78 This observation would indicate that electrolyte disturbances in patients with CKD are partly related to the underlying disease and partly to medications used in the management of concomitant comorbidities such as fluid overload and hypertension. However, it should be emphasized that the risk of hyperkalemia in patients with CKD, including those treated with renin-angiotensin-aldosterone system (RAAS) blockers, is relatively small.81
TABLE 72.1 Etiologies of Hyperkalemia in Patients with Renal Insufficiency | |||||||
---|---|---|---|---|---|---|---|
|
liter at 60 minutes).110 The administration of glucose with potassium stimulates insulin secretion and attenuates the rise in potassium in patients on dialysis as well as normal controls.105
ammonium ion (NH4+) and bicarbonate excretion.135,136,137 Careful metabolic studies by Goodman and colleagues137 documented that patients with chronic renal failure have a daily bicarbonate deficit of approximately 13 to 19 mEq. It is notable that despite this persistent deficit, serum bicarbonate in patients with CKD after an initial drop remains stable over long periods of time.138,139 This is due chiefly to the buffering of excess hydrogen ions by bone buffers, including calcium carbonate.138
often with HCA, our discussion is limited to the disturbances associated with renal insufficiency.
TABLE 72.2 Etiology of Chronic Hyperkalemia Due to Disturbances in Renal Potassium Excretion | |||||||||||||||||||||||||||||||||||||||||||||||||||||||||||||||||||||||||||||||||||||
---|---|---|---|---|---|---|---|---|---|---|---|---|---|---|---|---|---|---|---|---|---|---|---|---|---|---|---|---|---|---|---|---|---|---|---|---|---|---|---|---|---|---|---|---|---|---|---|---|---|---|---|---|---|---|---|---|---|---|---|---|---|---|---|---|---|---|---|---|---|---|---|---|---|---|---|---|---|---|---|---|---|---|---|---|---|
|
physiologic doses of fludrocortisone. This study,155
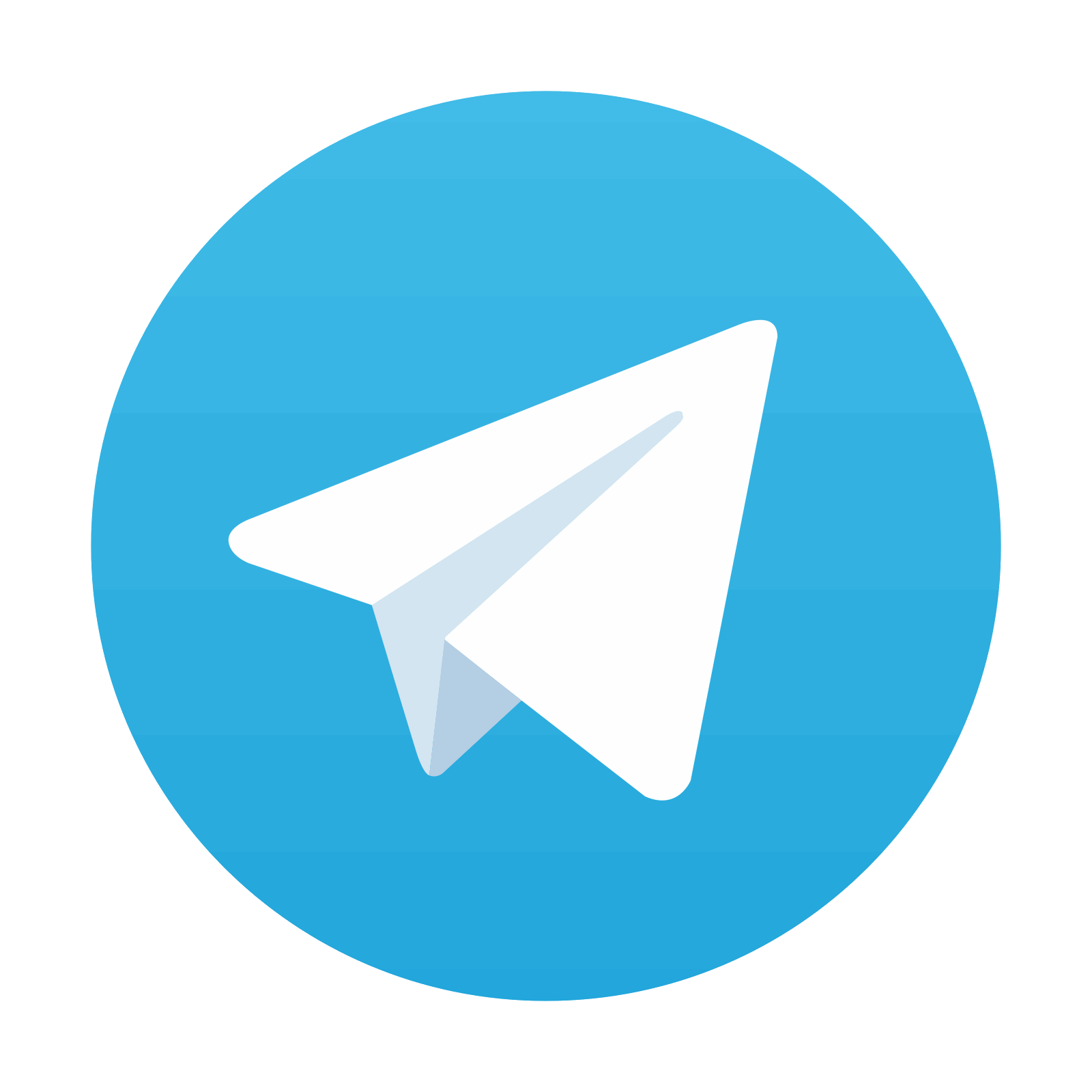
Stay updated, free articles. Join our Telegram channel

Full access? Get Clinical Tree
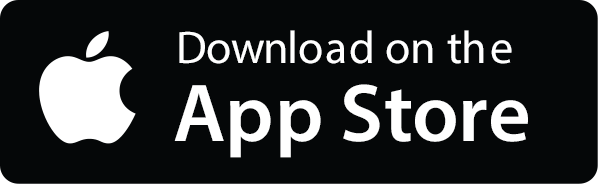
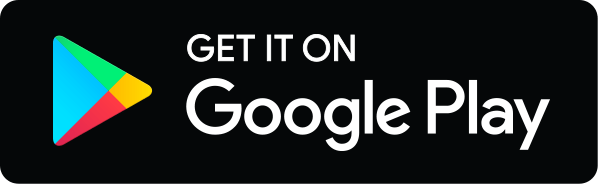
