Acute tubular necrosis
Urological/surgical complications
• Urinary leak
• Lymphocele
• Ureteral obstruction
• Catheter obstruction
• Bleeding/hematoma
Vascular causes
• Hypovolemia/hypoperfusion
• CNI toxicity (vasoconstriction)
• Thrombosis of the renal artery or vein
• Thrombotic microangiopathy/hemolytic-uremic syndrome (CNI use)
Recurrent disease
Acute rejection
Acute interstitial nephritis
Thirdly, the need for dialysis is somewhat subjective, which may impact documented rates of DGF. Akkina et al. [4] found the incidence of DGF to be two- to threefold higher when comparing two transplant programs in the same US city, despite similar donor and recipient parameters, laboratory data, and graft survival. The specific indications for dialysis could not be elicited from the dataset; however, these findings suggest that physician thresholds in prescribing dialysis post-transplantation may significantly impact DGF rates. Although novel biomarker techniques for the diagnosis of DGF have been studied in order to overcome the limitations noted above, none are yet clinically available. Thus, dialysis-based definitions continue to be most commonly used. Nevertheless, it seems clear that, in the absence of an ideal biomarker, a broader definition, incorporating both function and the need/reason for dialysis, would be more accurate and useful.
Pathophysiology of DGF
The coupled effect of warm and cold ischemia, as well as reperfusion of the kidney allograft after transplantation, results in a complex cascade of biochemical and structural changes at the cellular level. These changes predispose recipients to DGF, which is typically associated with histological findings of acute tubular necrosis (ATN) and intertubular neutrophilic infiltration [5, 6]. A wide spectrum of histopathologic changes, from mild to severe, has been associated with the clinical syndrome of DGF. These changes are more likely to be present after prolonged periods of cold ischemia [5, 7].
Ischemia results in intracellular hypoxia, which triggers multiple downstream effects including activation of anaerobic glucose metabolism and cellular depletion of ATP. Due to anaerobic metabolism, lactic acid is produced and intracellular acidosis ensues. Lytic enzymes are then activated which promote the generation of reactive oxygen species and result in tissue damage. Furthermore, cellular depletion of ATP results in inhibition of the Na/K ATPase which is essential in maintaining membrane potential. This allows sodium and water to enter the cell causing significant cellular edema. The intracellular osmolality of the cell also rises due to accumulation of adenosine, inorganic phosphate, and other intermediate compounds thus exacerbating the edema. The consequences of these processes include disruption of the cytoskeleton and cellular/mitochondrial membranes.
Reperfusion also plays an important role in renal injury. As oxidative metabolism is restored, a substantial and rapid release of reactive oxygen species including hydrogen peroxide and superoxide anions occurs, resulting in apoptosis. Furthermore, the generation of reactive oxygen species triggers a profound release of inflammatory cytokines recruiting neutrophils, monocytes, NK cells, and T cells to the allograft. Recruited leukocytes bind to adhesion molecules on endothelial cells plugging capillaries and increasing permeability of the endothelium. Ultimately, as a result of this complex pro-inflammatory cascade, damage to tubular cells and the vascular endothelium occurs. Furthermore, increases in endothelin and thromboxane, coupled with a decrease in prostacyclin, result in vasoconstriction, further aggravating tissue injury [1, 6, 8, 9].
The mechanisms of injury in DGF are complex and depicted schematically in Fig. 13.1. Although an in-depth description of the cellular and molecular mechanisms of injury in DGF is beyond the scope of this chapter, comprehensive reviews by Siedlecki et al. and Perico et al. highlight these mechanisms in greater detail [1, 6].
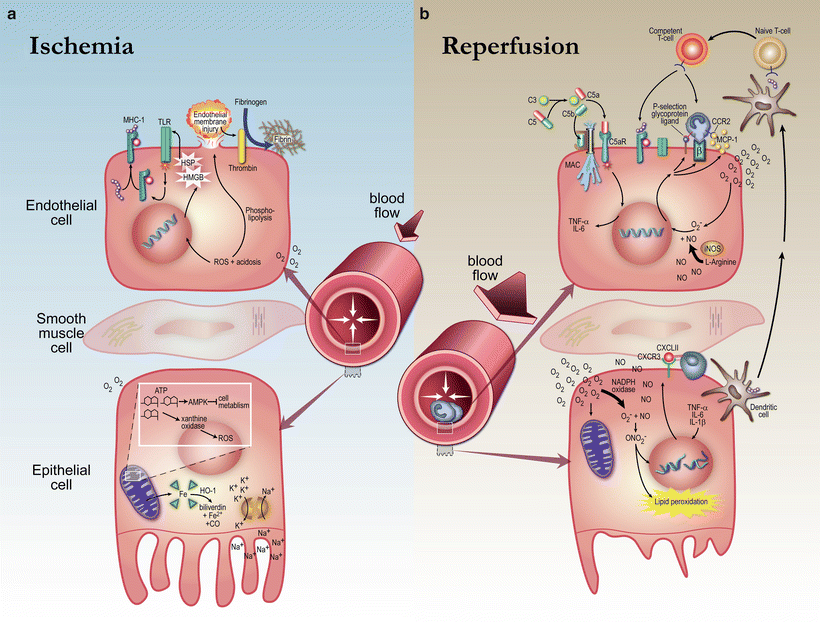
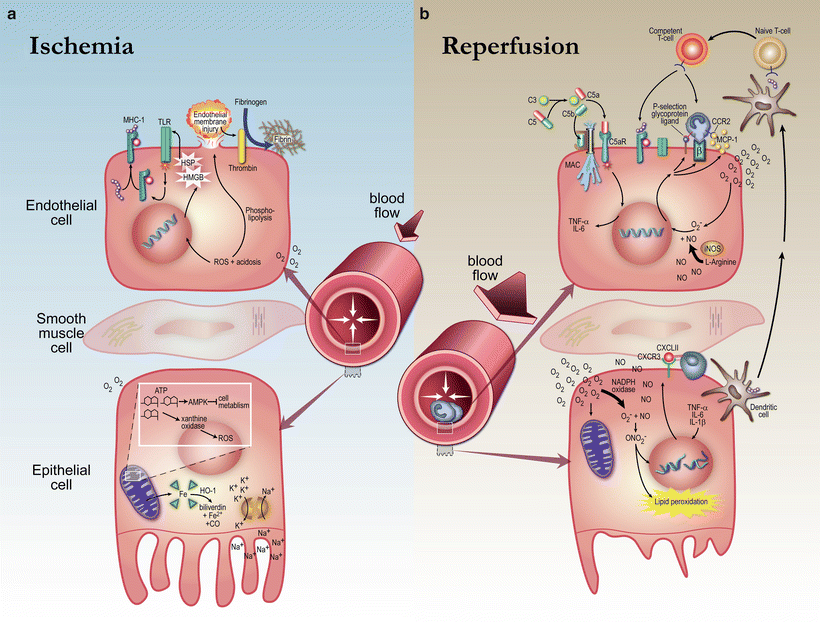
Fig. 13.1
Ischemia–reperfusion injury in delayed graft function. Cellular hypoxia triggers anaerobic metabolism resulting in cellular depletion of ATP, impairment of the Na/K ATPase, and generation of reactive oxygen species, which results in cellular edema and tissue injury. During reperfusion, reactive oxygen species trigger a profound release of inflammatory cytokines that recruit inflammatory cells causing damage to tubular cells and the vascular endothelium. Reproduced with permission from Siedlecki et al. Delayed Graft Function in the Kidney Transplant. American Journal of Transplantation, 2011. 11:p.2279–2296
Epidemiology of DGF
There is significant variability in the rates of DGF in the literature, ranging from 2 to 50 % depending on the transplant center or database [1]. This is in part due to the heterogeneity in the definitions of DGF in the literature as well as differences across donor subtype. Given the ongoing shortage of organs available for transplantation, the use of expanded criteria donor (ECD) and donation after cardiac death (DCD) kidneys has increased over time, which heavily influences rates of DGF [7, 10, 11]. Thus, despite improvements in overall graft survival, DGF rates continue to increase, in part, due to the composition of the deceased donor pool.
In 2009, the USRDS reported DGF rates of 22 % in recipients of deceased donor kidneys [10]. DCD kidneys are associated with the highest rates of DGF, occurring in 37 % of recipients [10]. The obligate warm ischemia time with DCD kidneys, defined as the time between circulatory arrest and the commencement of cold storage, enhances the ischemia–reperfusion injury resulting in the highest rates of DGF across any subgroup of donor kidneys [12]. Brain death results in a variety of hemodynamic and hormonal derangements, including a systemic inflammatory response and a surge in catecholamine levels, resulting in severe vasoconstriction and hypoperfusion of the allograft [13, 14]. Therefore, DGF also occurs in kidneys from brain dead donors, with rates of 20 and 31 % in standard criteria donor (SCD) and ECD kidneys, respectively [10].
Although DGF occurs most commonly in recipients of deceased donor kidney transplants, it also occurs in living donor kidney transplants, albeit to a much lesser degree. The USRDS reported a DGF rate in living donor kidney transplants of 3.4 % [10]. The risk factors for DGF in living donor kidney transplants are likely distinct from those in deceased donor kidney transplants [10, 15–17]. However, the implications of DGF on kidney transplant outcomes may be just as significant [16, 18, 19].
Causes and Risk Factors: Transplant, Donor, and Recipient Factors
In addition to donor subtype, various recipient, donor, and transplant factors have been associated with an increased risk of DGF. Cold ischemia time (CIT) is one of the strongest risk factors for the development of DGF [20, 21]. In a large USRDS retrospective cohort study of 37,216 patients, CIT was found to be an independent risk factor for DGF with adjusted odds ratios of 1.38–3.48. Furthermore, the longer duration of CIT resulted in worse outcomes, with an increased risk of DGF of 23 % for every 6-h increase in CIT [21]. In the same study, donor age of >50 years was also a significant risk factor for DGF with an odds ratio of 2.07 (95 % CI: 1.89, 2.27; P value <0.001) [21]. This finding has been subsequently demonstrated in several more recent observational studies. [22, 23] There is also evidence that HLA-mismatched kidneys are associated with a higher risk of DGF than zero-mismatched kidneys [23–25].
In order to elucidate the role of recipient factors as determinants of DGF, Doshi et al. conducted a retrospective cohort study of pairs of kidney transplant recipients who shared the same donor, but only one recipient developed DGF [20]. Although there are limitations to this study, including potential confounding due to unmeasured variables, significant amounts of missing data, and lack of information on potential technical problems at the time of surgery, it provides new insights into the potential role of recipient factors as determinants for the risk of DGF. In this analysis of 5,382 recipient pairs, the following recipient risk factors were independently associated with an increased risk of DGF: male sex, African American race, diabetes, prior sensitization, prolonged wait time, and recipient obesity (BMI > 30 kg/m2). As the prevalence of obesity increases, recent data have shown that obesity in the donor, in addition to the recipient, is also associated with an increased risk of DGF [26–28]. It has been hypothesized that obesity results in a pro-inflammatory state that may potentiate the ischemia–reperfusion injury in the allograft [29].
Multiple observational studies have evaluated the impact of pre-transplant dialysis modality on the post-transplant risk of DGF [30–35]. Two large registry studies have yielded conflicting results. Snyder et al. studied over 252,000 kidney transplant recipients and found that DGF rates were lower in peritoneal dialysis (PD) patients when compared to patients on hemodialysis (HD) [34]. However, more recently, a large registry study compared PD and HD patients using both conventional multivariable models and propensity score matching in a contemporary cohort of over 14,000 patients [33]. This study did not show any association between pre-transplant dialysis modality and risk of DGF. It is possible that residual confounding due to the observational nature of these studies may explain the variability in the results. The impact of pre-dialysis modality remains a largely unresolved issue at this time. It has been hypothesized that higher rates of DGF in HD patients may be attributable to the resulting state of volume depletion and predisposition to ischemic injury. Van Loo et al. demonstrated that in HD patients, ultrafiltration in the 24-h period prior to transplantation was associated with an increased incidence of DGF in comparison to HD patients without ultrafiltration [36]. Conversely, maintaining adequate intravascular volume via CVP monitoring (>8 mmHg) appears to reduce the risk of DGF [37]. Thus, avoidance of aggressive ultrafiltration prior to transplant, and the maintenance of adequate intravascular volume post-transplant, is a reasonable approach to decrease the risk of DGF.
Given the multitude of recipient, donor, and transplant risk factors identified in various reports, Irish et al. [23] derived a risk prediction model in recipients of deceased donor kidneys to quantify the risk of DGF. Using data from a cohort of 24,653 adult recipients of deceased donor kidneys captured in the UNOS database, 18 variables were included in the final predictive model. The five most significant predictor variables included CIT, terminal serum creatinine, recipient BMI, DCD kidney, and donor age. However, it is important to note that certain variables, which may be predictive of DGF, are not necessarily captured in the registry used to derive this prediction model. Nonetheless, the prediction model was externally validated using a separate dataset and showed good predictive ability with a concordance statistic (i.e., area under the receiver operating characteristic curve) of 0.704. Furthermore, the performance of the model was equally good across donor subtypes (i.e., ECD, DCD, and SCD). Given the large number of variables included in the model, the authors developed a nomogram as well as an online prediction tool to improve the usability of the model for clinicians [23].
Clinical Evaluation
The clinical manifestations of DGF are nonspecific, and therefore the clinician must exclude other potential causes of graft dysfunction and/or oliguria in the early post-transplant period. A recommended approach is depicted in Fig. 13.2 [38]. A careful volume assessment should be performed to rule out the possibility of hypovolemia and if present, volume expansion should be pursued. Renal Doppler ultrasound is an important step in the evaluation of DGF in order to rule out vascular complications such as arterial or venous thrombosis or urological complications such as urinary leak, lymphocele, hematoma, or ureteric obstruction. If urological complications are found, additional testing (e.g., cystoscopy with retrograde pyelogram) should be considered to delineate the urological abnormality prior to further surgical intervention. Additionally, if the patient is on a calcineurin inhibitor (CNI), drug levels can be measured to aid in the assessment of CNI toxicity. In the absence of an identifiable cause, a kidney transplant biopsy should be performed to rule out certain etiologies such as acute rejection or thrombotic angiopathy (see section “Management of DGF”) [38]. The timing of renal biopsy is variable depending on the clinical scenario and the transplant center. In our center, we favor a biopsy at approximately 7 days post-transplant in patients with persistent graft dysfunction. Given the low incidence of DGF in living donor kidney transplant recipients, a comprehensive search for alternate explanations in this population is of utmost importance.
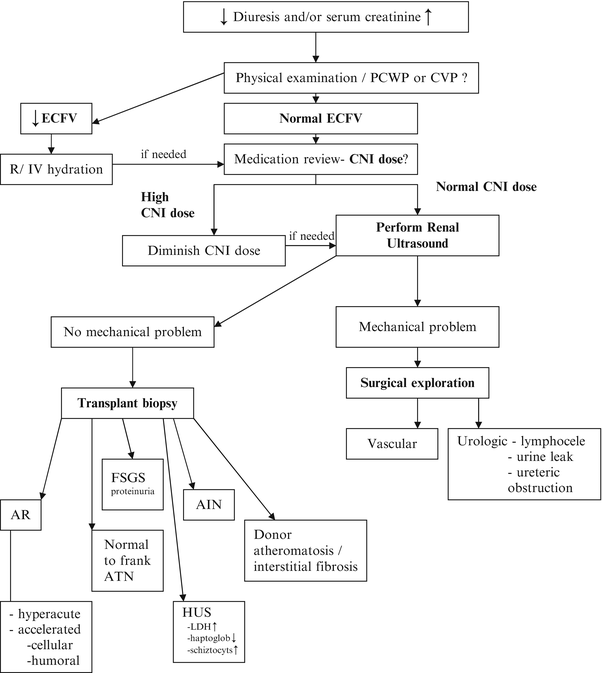
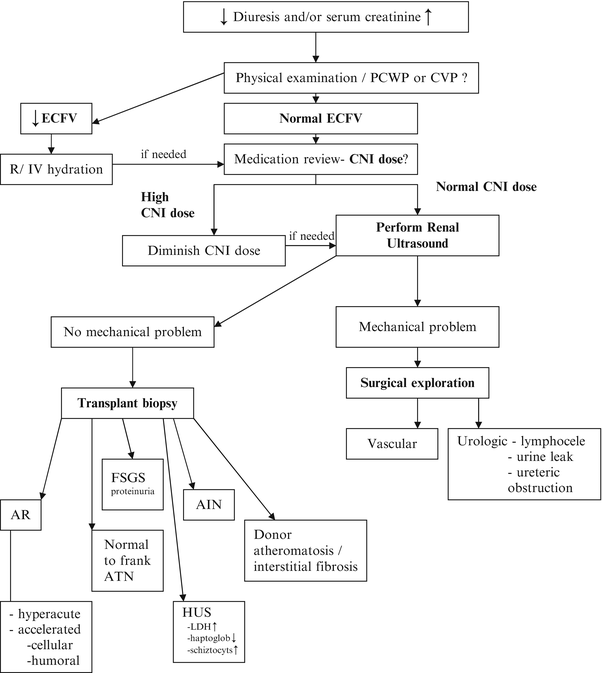
Fig. 13.2
Management of delayed graft function. AIN acute interstitial nephritis, AR acute rejection, ATN acute tubular necrosis, CNI calcineurin inhibitor, CVP central venous pressure, ECFV extracellular fluid volume, FSGS focal segmental glomerulosclerosis, HUS hemolytic-uremic syndrome, PCWP pulmonary capillary wedge pressure, Screat serum creatinine. Reproduced with permission from Peeters, P., et al., Delayed graft function in renal transplantation. Current Opinion in Critical Care, 2004. 10(6): p. 489–98
Prevention of DGF
When kidneys are recovered, they are generally flushed and cooled with a preservation solution, a process referred to as static cold storage. Cooling of the organ is protective by decreasing the metabolic rate at the cellular level during the ischemic period [6]. A number of preservation solutions have been developed to offset the biochemical changes that occur as a result of ischemia, with the intent to decrease DGF rates. Commonly used preservation solutions include the Eurocollins (EC) solution, University of Wisconsin (UW) solution, Celsior solution, and histidine-tryptophan-ketoglutarate (HTK) solution. Although the differences among these solutions at a biochemical level are beyond the scope of this chapter, multiple studies have attempted to determine the most effective solution for decreasing the rates of DGF with variable results [39–41]. A recent meta-analysis of 15 studies showed an increased risk of DGF with the EC solution when compared to UW and HTK. However, no significant difference in DGF risk was found between UW, HTK, and Celsior solutions [42]. The randomized controlled trials (RCTs) included in the meta-analysis were generally of poor quality with variable reporting of blinding, the use of intention-to-treat analysis, and allocation concealment. Although multiple RCTs of acceptable size were included in this meta-analysis, the pooling of data cannot overcome the poor quality of the individual studies; thus, the results cannot be considered definitive.
The use of pulsatile machine perfusion (PMP) rather than cold storage has been explored in a number of large, well-designed, international, multicenter RCTs. Among SCD and ECD kidneys, two RCTs have demonstrated benefit in reducing DGF rates by using PMP rather than cold storage [43, 44]. Furthermore, a cost analysis of PMP utilization in ECD kidneys was associated with a decrease in hospitalization expenditures [45]. For DCD kidneys, however, similar high quality RCTs have yielded conflicting results. Watson et al. showed no benefit in PMP in comparison to cold storage in preventing DGF in recipients of DCD kidneys [46]. However, Moers et al. [43] and Jochmans et al. [47] showed significant improvements in DGF rates with PMP. The reasons for these conflicting data are not entirely clear but may at least partially relate to differences in study design and the populations studied. Nevertheless, the weight of the evidence suggests that PMP may reduce the risk of DGF and its associated costs in select kidney transplants.
The use of vasodilatory drugs has been extensively studied in the prevention of DGF. In particular, multiple, small, randomized trials have demonstrated that calcium channel blockers are potentially effective in the prevention of DGF. However, a significant limitation in the interpretation of the results is the small number of patients included in these studies. Furthermore, many of these studies were conducted prior to the increased use of ECD and DCD kidneys, thus limiting their generalizability. In order to overcome this issue, a systematic review was conducted, which included 13 RCTs comparing calcium channel blockers to placebo or no treatment [48]. Overall, the use of calcium channel blockers was associated with a decrease in DGF (risk ratio 0.55, 95 % CI: 0.42–0.73). However, there was significant heterogeneity among the studies included for analysis and the overall quality of the studies was poor. In particular, there were substantial differences in the definition of DGF used, the type of calcium channel blocker administered, the route of drug delivery (PO, IV, or in the preservation solution), and timing of delivery (i.e., pretreatment of recipient vs. treatment of donors). In addition, whether blinding had occurred was unclear in several studies. Given the potential adverse effects of using calcium channel blockers in the post-transplant period (e.g., increase in CNI levels), as well as the lack of large, high quality, and contemporary RCTs demonstrating benefit, these agents have generally not been used for the prevention of DGF [48].
Other vasodilatory agents, such as endothelin agonists, adenosine receptor antagonists, and atrial natriuretic peptide, have also been studied but their efficacy has not been well established. Additionally, given the importance of reactive oxygen species and the inflammatory response in the pathogenesis of DGF, multiple anti-inflammatory and antioxidant therapies are also being actively studied for potential future therapeutic use [6].
Use of dopamine in the donor has also been explored as a strategy to decrease the incidence of DGF. Dopamine optimizes renal perfusion, protects endothelial cells from oxidative stress, down-regulates endothelial adhesion molecules, and alters chemokine release, which are plausible mechanisms to explain the effect of dopamine to reduce the risk of DGF. In addition to small observational studies, a multicenter RCT of 264 patients evaluated the impact of a continuous infusion of dopamine in the donor until cross clamping had occurred [49, 50]. The results of this study showed that low dose dopamine was associated with a decrease in DGF rates. A subgroup analysis demonstrated that the effect of dopamine was more pronounced in kidney transplants with mean CIT of >21.2 h. However, the small sample size and open-label design were significant limitations of this study. Thus, this observation requires further substantiation before implementing it into routine clinical practice.
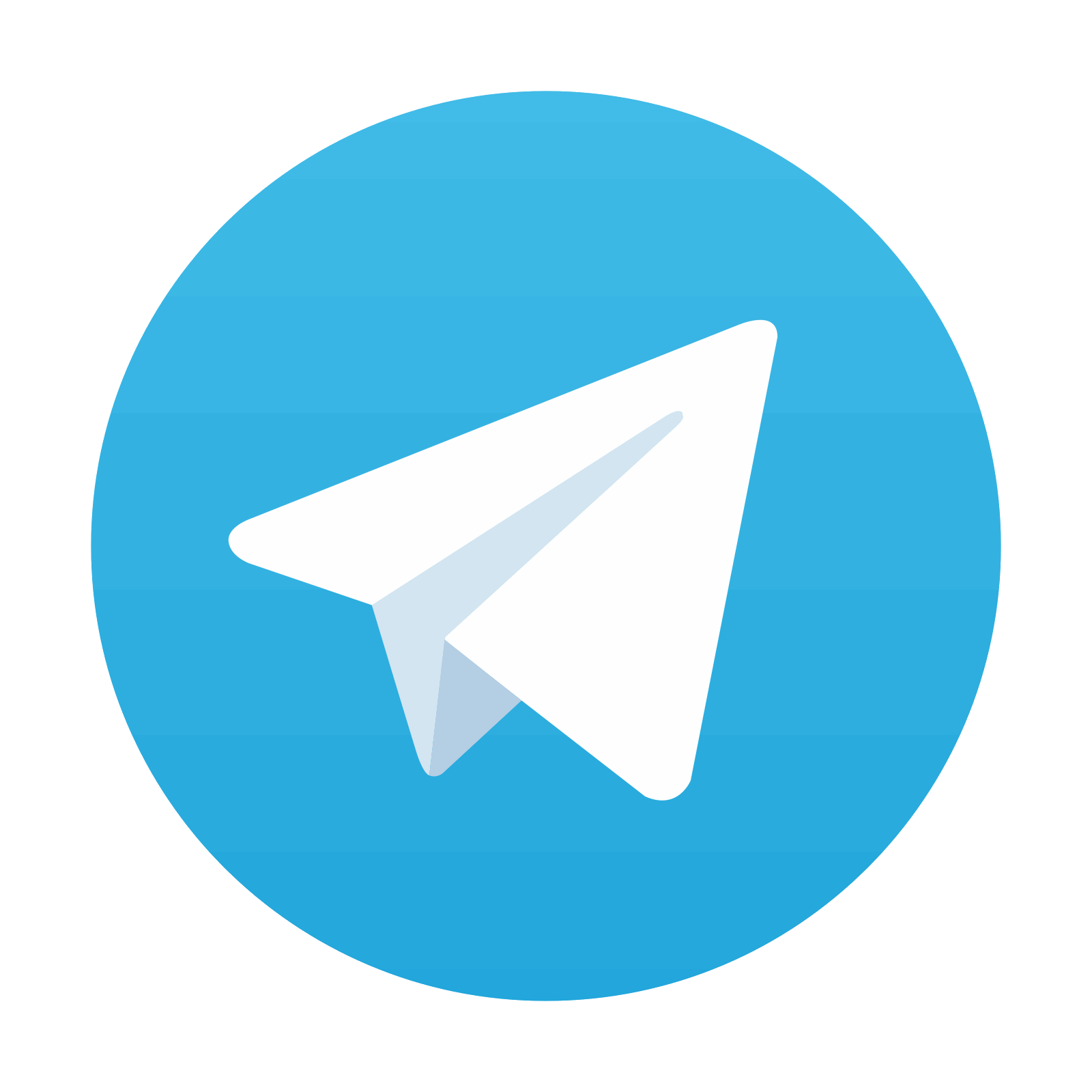
Stay updated, free articles. Join our Telegram channel

Full access? Get Clinical Tree
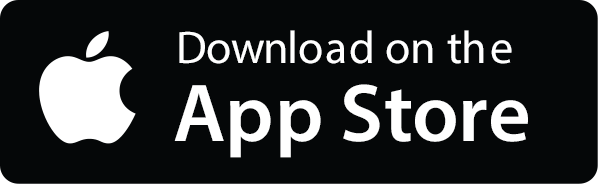
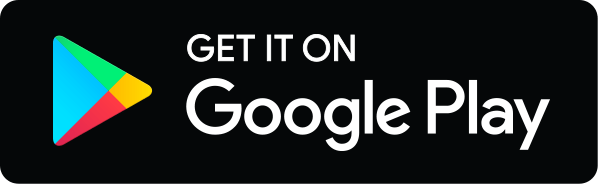