Introduction
Despite advances in understanding the pathophysiology of acute kidney injury (AKI) and chronic kidney disease, treatment for kidney disease remains unsatisfactory. In this chapter, we briefly recount the importance of acute kidney injury and chronic kidney disease, then provide a brief description of labile iron, and, finally, summarize the role of labile iron in acute and chronic kidney disease. The availability of iron chelators provides new therapeutic tools to prevent and/or treat kidney disease.
Acute kidney injury is an independent risk factor for morbidity and mortality. A modest increase (0.3 mg/dL) in serum creatinine is associated with high in-hospital mortality, and AKI is associated with post-hospital discharge mortality and progression to end-stage kidney disease (ESKD).
Chronic kidney disease (CKD) affects approximately 10-15% of the adult population worldwide, is an independent risk factor for cardiovascular disease, and carries a high economic cost. There is a worldwide increase in the incidence and prevalence of diabetes, which is the most common cause of CKD. These observations, coupled with the increasing incidence of end-stage kidney disease despite the use of angiotensin receptor blockers (ARBs) and the multiplier effect of CKD on cardiovascular disease, indicate a major importance of CKD as a global public health problem. The failure of recent studies to halt diabetic nephropathy highlights an urgent need for new therapeutic modalities to halt progression of kidney disease.
Definition of Catalytic (Labile) Iron and Its Importance in Tissue Injury
Iron is the most abundant transitional metal in the body. The term “labile iron pool” was first used to denote a transient iron pool of low-molecular-weight, weakly chelated iron that passes through the cell. Methodological approaches for detection of this pool of iron ions are based on the use of metal chelators. Critical to iron’s importance in biological processes is its ability to cycle reversibly between its ferrous and ferric oxidation states. This precise property, which is essential for its functions, also makes it very dangerous, because free iron can catalyze the formation of free radicals that can damage the cell. Thus, from a pathophysiological standpoint, the broadest definition of a labile iron pool is that it consists of chemical forms that can participate in redox cycling, and is therefore often referred to as catalytic iron.
The catalytic iron pool is estimated to be less than 100 mg compared to the total iron in the body, which is approximately 4 g. In most cells iron homeostasis consists of iron uptake, utilization, and storage. The process of iron uptake is carried out by a transferrin receptor (TFR) and a divalent metal transporter 1 (DMT1, also called DCT1; NRAMP2), whereas ferritin is an intracellular, iron-sequestering protein. Studies are beginning to yield information on the pathways of iron transport, its export from the cell via the divalent iron ion exporter ferroportin 1, and its regulatory mechanisms including hepcidin. Since uptake and storage of iron is carried out by different proteins, the pool of accessible iron ions constitutes a crossroad of metabolic pathways of iron-containing compounds.
Studies using a variety of methods have begun to define intracellular distribution of labile iron (for reviews see Kruszewski and Esposito ). Using several techniques including laser scanning microscopy, the concentration and distribution of chelatable iron has been estimated to be about 5.0 to 15 μM in the cytoplasm and subcellular organelles including mitochondria and nuclei. In vivo , most of the iron is bound to heme or non-heme protein and does not directly catalyze the generation of hydroxyl radicals or a similar oxidant. The bleomycin-detectable iron assay measures catalytic iron and is based on the observation that the anti-tumor antibiotic bleomycin, in the presence of catalytic iron, binds to and degrades DNA with the formation of a product that reacts with thiobarbituric acid to form a chromogen. Thus the assay detects iron complexes capable of catalyzing free-radical reactions in biological samples. The binding of the bleomycin-iron complex to DNA makes the reaction site-specific and antioxidants rarely interfere. The bleomycin assay detects only “free” iron and not iron bound to specific transport proteins or to enzymes.
The ability of iron to participate in redox cycling makes it potentially hazardous by enabling it to participate in the generation of powerful oxidant species such as hydroxyl radical (metal-catalyzed Haber-Weiss reaction, below) and/or reactive iron-oxygen complexes such as ferryl or perferryl ion. In several systems, the amount of free-radical generation is related to the amount of labile iron present ( Figure 89.1 ).
Fe 3+ + O 2 – • → Fe 2 + + O 2 Fe 2+ + H 2 O 2 → Fe 3+ + − OH + • OH H 2 O 2 + O 2 – • ⟶ Fe 3+ − OH + • OH + O 2
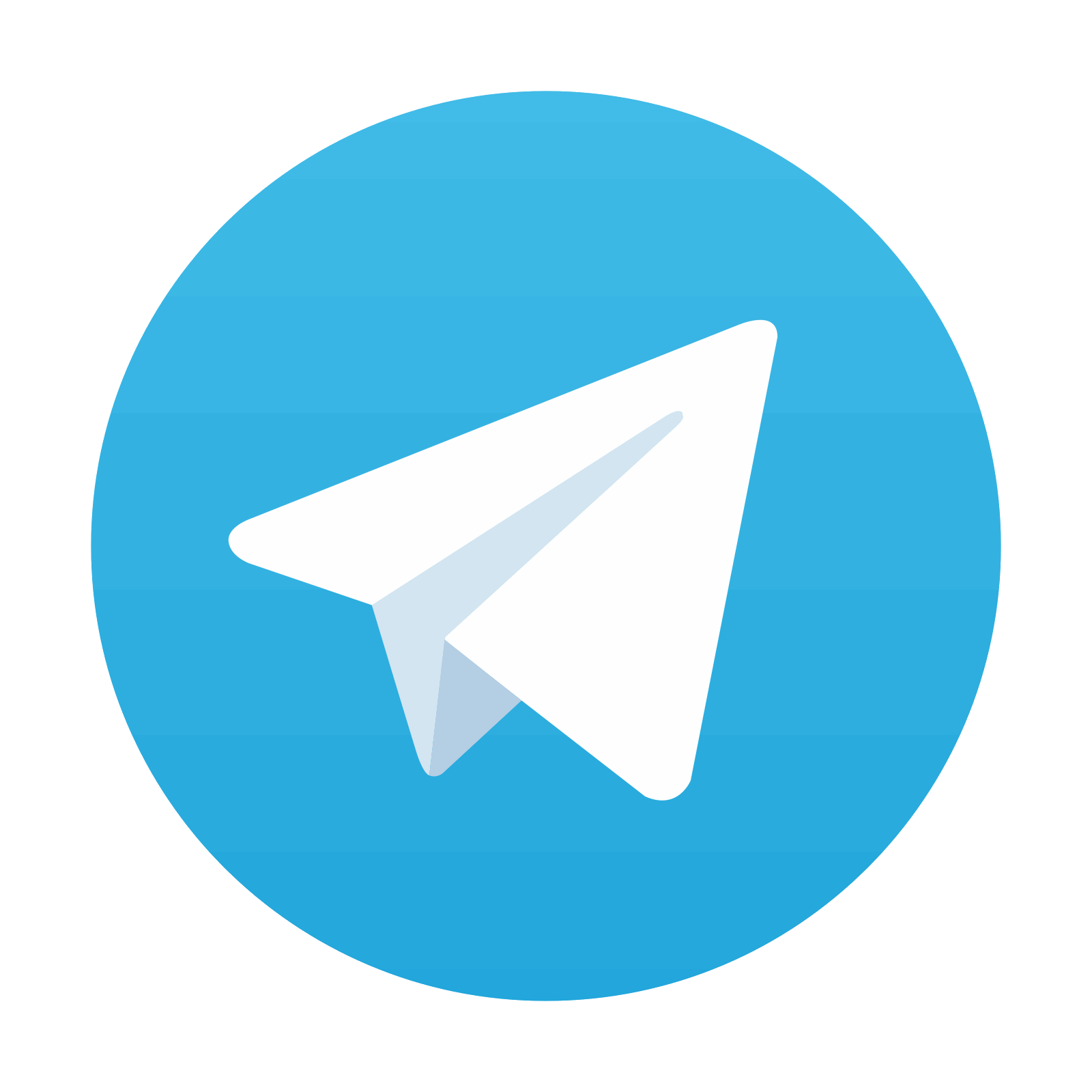
Stay updated, free articles. Join our Telegram channel

Full access? Get Clinical Tree
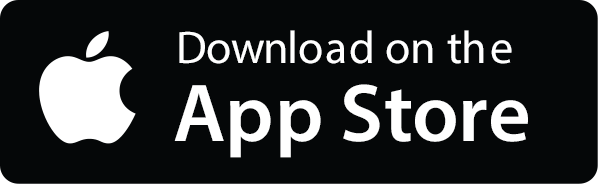
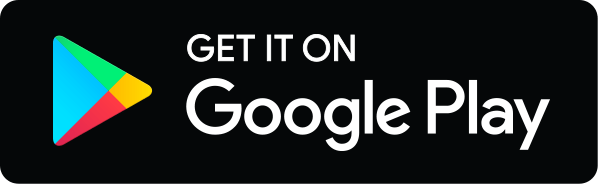