Cardiac Disease in Chronic Kidney Disease
Patrick S. Parfrey
Sean W. Murphy
INTRODUCTION
Acute or chronic dysfunction in the heart or kidneys can cause dysfunction in the other organ, producing cardiorenal syndromes that were classified in 2008 by Ronco and colleagues1 into five different types. In type I, or acute cardiorenal syndrome, an abrupt worsening of cardiac function leads to acute kidney injury. In type II, or chronic cardiorenal syndrome, chronic cardiac dysfunction causes progressive and potentially permanent chronic kidney function impairment. In type III, or acute renocardiac syndrome, an abrupt worsening of kidney function causes acute cardiac dysfunction. In type IV, or chronic renocardiac syndrome, chronic kidney dysfunction contributes to cardiac dysfunction. In type V, or secondary cardiorenal syndrome, a systemic condition such as diabetes mellitus or sepsis causes both cardiac and renal dysfunction. This chapter will focus on type IV, chronic renocardiac syndrome.
Both estimated glomerular filtration rate (eGFR) and albuminuria are independent predictors of cardiovascular (CV) events.2 In over a million enrollers in the California Kaiser Permanente health maintenance organization (HMO), the degree of kidney function impairment independently and incrementally predicted subsequent cardiac events, hospitalization, and all-cause mortality.3 Among 11,640 patients with type 2 diabetes, a tenfold increment in urinary albumin to creatinine ratio independently increased the risk of CV events by 2.5-fold and that of CV death by 3.9-fold.4 Every halving of baseline eGFR was associated with a 2.2-fold increase in a risk of CV events and a 3.6-fold increase in risk of CV death.4 In a recent meta-analysis, mortality risk was doubled at eGFR levels of 30 to 45 mL/min/1.73 m2 or urine albumin to creatinine ratio > 11.3 mg per millimole as compared with their normal ranges.5 Furthermore, patients with higher levels of proteinuria within a given level of eGFR had an increased risk of adverse outcomes.6
Not only is the baseline eGFR predictive of CV events, but so is the rate of decline of eGFR. The Cardiovascular Health Study demonstrated that those that had a more rapid decline in eGFR over a 7-year period had increased risk of CV events during the subsequent 8-year period: an increased risk of heart failure of about 30% and an increased risk of myocardial infarction (MI) of about 40%.7 In the U.S. Atherosclerosis Risk in Communities (ARIC) study, individuals with greatest annual declines in eGFR had higher risk of incident coronary heart disease and of all-cause mortality at 3 and 9 years than those with stable eGFRs.8
In the Cardiovascular Health Study, the 3-year probability of CV events increased as the GFR declined below 70 mL per minute.9 However, the probability decreased substantially after adjustment for traditional risk factors. Below a eGFR level of 30 mL per minute, the CV event rate is very high and likely influenced by uremia-related risk factors. After starting dialysis therapy, atheromatous event rates are much higher than before dialysis. In the United States, the incidence of de novo coronary events, stroke, and peripheral vascular disease of hemodialysis patients, after 2.2 years follow-up from the start of dialysis, were 10.2%, 2.2%, and 14%, respectively.10 In addition, the uremic state is certainly cardiomyopathic and predisposes the patient to heart failure. This is supported by numerous studies: a high incidence of heart failure in CKD,11 a high prevalence of cardiac failure on starting dialysis,12 a higher rate of de novo symptomatic heart failure in renal transplant recipients than in the general population,13 and a high incidence of de novo cardiac failure in hemodialysis patients (13.6% over a 2.2-year mean follow-up in the United States and 17% over a 3.4-year follow-up in Canada).14,15
The rate of CV death in patients on dialysis is substantially higher than that of the general population in all age groups, particularly in the younger group (Fig. 79.1).16 This enormous burden of cardiac disease is related to the high prevalence of different cardiac/vascular diseases, the high rate of traditional cardiac risk factors, and the cardiopathic and vasculopathic hemodynamic and metabolic milieu associated with chronic kidney disease (CKD).
The Pathogenesis of Cardiac Disease
The CV disorders associated with CKD are multiple and often present in the same patient (Fig. 79.2). Atherosclerotic events occur frequently among patients with CKD11
because of the high prevalence of diabetes, hypertension, dyslipidemia, and the likelihood that uremia-related factors increase atherosclerotic risk, particularly when eGFR falls below 30mL/min/1.73 m2. Arteriosclerosis, a diffuse disorder of large conduit arteries, is characterized by dilated, ectatic, noncompliant vessels. Such noncompliance can be exacerbated by metastatic vascular calcification.17
because of the high prevalence of diabetes, hypertension, dyslipidemia, and the likelihood that uremia-related factors increase atherosclerotic risk, particularly when eGFR falls below 30mL/min/1.73 m2. Arteriosclerosis, a diffuse disorder of large conduit arteries, is characterized by dilated, ectatic, noncompliant vessels. Such noncompliance can be exacerbated by metastatic vascular calcification.17
![]() FIGURE 79.2 The pathogenesis of cardiovascular disease in chronic kidney disease (CKD). LV, left ventricular; LVH, left ventricular hypertrophy. |
Concentric left ventricular hypertrophy (LVH) also occurs frequently because of LV pressure overload caused by hypertension, arteriosclerosis, and sometimes aortic stenosis.18 Eccentric LVH results from LV volume overload associated with hypervolemia, arteriovenous fistula, and anemia. During years on dialysis LV growth occurs,19 although it regresses following renal transplantation.20 LVH can progress
to LV systolic dysfunction through progressive myocyte loss, but LV diastolic dysfunction also frequently results in symptomatic pulmonary edema.21
to LV systolic dysfunction through progressive myocyte loss, but LV diastolic dysfunction also frequently results in symptomatic pulmonary edema.21
Ischemic Heart Disease
Coronary artery disease. Symptomatic myocardial ischemia usually results from flow-limiting critical coronary atherosclerotic disease, but may be present in the absence of significant angiographic disease in approximately 25% of patients (Fig. 79.3).22 In the latter case, small-vessel disease or a decrease in myocardial capillary density consequent to LVH or fibrosis may contribute to the ischemic symptoms.23,24,25 LVH itself may predispose the patient to ischemia, and it may be associated with increased diastolic coronary blood flow caused by arteriosclerosis.
In non-CKD populations, the initiating event of atherosclerosis appears to be endothelial injury caused by mechanical stress (e.g., hypertension) or endothelial toxins (nicotine, oxidative stress, hyperlipidemia, and inflammation) that alter the endothelial phenotype to a more permeable, procoagulant state.26 Endothelial denudation or, more commonly, alterations in cell surface receptor expression, permits the access of lipoproteins and macrophages into the subintimal space.26,27 The oxidative modification of lipoproteins, particularly of low-density lipoprotein (LDL), is chemotactic for macrophages and facilitates the uptake of oxidized lipids by macrophages, resulting in the formation of foam cells.28 Oxidized LDL stimulates the elaboration of growth factors that are mitogenic for smooth muscle and are profibrotic.29 These processes result in the accumulation of oxidatively modified lipids and inflammatory cells at the center of a fibrous “cap” of variable thickness. This cap may rupture, causing subocclusive thromboses that may be minimally symptomatic or associated with acute coronary syndromes (e.g., unstable angina, myocardial infarction). These thrombi eventually become organized in the wall of the vessel. Calcific deposits may develop. The mature atheroma thus may contain, in addition to lipids, inflammatory cells, collagen, an organized thrombus, and calcium.30 The propensity for rupture varies with the composition of the atheroma. Lipid and inflammatory cell-rich atheromas with thin fibrous caps are thought to be more
unstable than those with a greater degree of fibrosis and those that are less lipid.31
unstable than those with a greater degree of fibrosis and those that are less lipid.31
![]() FIGURE 79.3 The etiology of ischemic heart disease in chronic kidney disease. Dis, disease; ADMA, asymmetric dimethyl arginine; LV, left ventricular |
The histologic classification of coronary artery disease (CAD) lesions is outlined in Figure 79.4.32 Initial lesions, type I through III, are small and silent, whereas types IV to VI may produce clinical events or may remain silent. The riskiest lesion is type VI, where surface defects and hematoma and a thrombus occur. The anatomic study of carotid plaque composition in 46 CKD patients with critical stenosis was compared to plaques in 56 CAD patients with normal renal function.33 In CKD patients compared to controls, plaques were more frequently unstable (83% versus 52%), ruptured (59% versus 36%), and calcified (17% versus 7%), and the fibrous component was reduced (40% versus 57%).
Postmortem data from patients with CKD show increased vascular medial thickness and a smaller lumen area relative to control subjects matched for age and gender.31 Although carotid artery intima-media thickness is a marker of atherosclerotic plaques in the general population, in CKD it may be increased by factors unrelated to atherosclerosis. In 406 patients with CKD and without overt CV disease (CVD), intima-media thickness was inversely correlated with eGFR, but had no association with traditional CV risk factors.34 Furthermore, following a renal transplantation, intimamedial thickness normalized within 90 days. It is possible that shear stress associated with fluid overload, endothelial dysfunction, or other factors induce the increase in intima-media thickness seen in CKD.
Theoretically, renal failure can modify the atherogenic process at multiple levels. Hypertension and flow overload may increase stresses on the vascular wall at bifurcations. In many patients on dialysis, a prooxidant and chronic inflammatory state pertains, and both may contribute to endothelial dysfunction.35 Hyperhomocysteinemia may promote endothelial activation and thrombosis by mechanisms that are yet unclear.36 Hyperparathyroidism and divalent ion abnormalities may promote vascular calcification and medial hypertrophy.17 The presence of calcium identified by electron beam tomography correlates with advanced atherosclerosis and appears to be more prevalent in patients with end-stage renal disease (ESRD).37,38
Asymmetric dimethyl arginine (ADMA) is an endogenous competitive inhibitor of nitric oxide synthase (NOS) and reduces nitric oxide generation, thus inhibiting the beneficial affect of nitric oxide on vasodilation, arterial stiffness, and endothelial function. The accumulation of ADMA inhibits NOS in endothelial cells and induces endothelial dysfunction, vasoconstriction, and atherosclerosis. ADMA levels are inversely related to GFR in patients with mild-to-moderate CKD. In renal transplant recipients, ADMA levels were an independent and a significant risk factor for major cardiac events and all-cause mortality.39 A similar association was previously reported for dialysis patients.40
Matrix metalloproteinases and their specific tissue inhibitors regulate the proteolysis of the vascular extracellular matrix, and their balance is important in atherosclerosis and plaque destabilization. A cross-sectional study of 111 patients with stage 1 through 4 CKD, 217 dialysis patients, and 50 healthy controls demonstrated elevated levels of circulating metalloproteinase-10 associated with a severity of CKD.41 A composite atherosclerosis score was highest in dialysis patients, and the severity of atherosclerosis was associated with the elevated levels of circulating metalloproteinase-10. It is possible that the riskier plaques seen in CKD may be caused, at least in part, by the high levels of metalloproteinases.
Microvascular disease. In the absence of flow-limiting CAD, ischemic symptoms may result from a reduction in coronary vasodilator reserve and altered myocardial oxygen delivery. Intracoronary ultrasonography has shown that angiographically normal vessel segments may contain areas of nonencroaching atheroma. Endothelial function is impaired in these vessels and may reduce coronary vasodilator reserve (i.e., the ability of the vessel to dilate above baseline in response to increased myocardial oxygen demand).42 Vasodilator reserve is clearly impaired in vessels with lumenencroaching disease.43 The resulting mismatch of supply and demand may give rise to symptomatic ischemia.
Small vessel disease may occur in LVH and diabetes, and because of uremia per se. In LVH, small vessel smooth muscle hypertrophy and endothelial abnormalities can diminish coronary reserve. Diabetes may be associated with microvascular disease characterized by endothelial proliferation, subendothelial fibrosis, and exudative deposits of hyaline in the intima.44 In uremic rats, LVH is associated with a severe reduction in myocardial capillary density compared with hypertensive rats that were matched for weight and blood pressure (BP).25 Small vessel calcification may also impair coronary reserve.
Dysregulation of energy metabolism. An impairment in energy supply in the myocyte may increase cellular susceptibility to ischemia. A reduced myocardial phosphocreatineadenosine triphosphate ratio under stress has been observed in uremic animals.45 Hyperparathyroidism may play a critical role in the dysregulation of cellular energetics, and may thus exacerbate ischemic damage to the myocardium.46
Impaired myocardial fatty acid metabolism and insulin resistance, both of which reduce the synthesis of myocardial adenosine triphosphate, cause a deficiency in the myocardial energy supply. Visualization of impaired fatty acid metabolism is possible using single-photon emission computed tomography (SPECT) with the iodinated fatty acid analog iodine, 123-B-methyliodophenyl pentadecanoic acid (BMIPP). Uptake of this analog on SPECT images has been graded in 17 segments using a 5-point scale, and provides a measure of recurrent myocardial ischemia.47 Among 155 prevalent hemodialysis patients without obstructive coronary artery disease on angiography, subsequent cardiac death was associated with both BMIPP score > 12 and also with increased insulin resistance scores, suggesting an important role for impairment in
myocardial energy supply in the occurrence of cardiac death in these patients.47 In a prospective study of a larger group (N = 318) of asymptomatic hemodialysis patients without a clinical history of MI or coronary revascularization who were followed for 3.6 ± 1 year, a BMIPP score > 12 was again strongly associated with cardiac death (hazard ratio [HR]: 22; 95% confidence interval [CI] = 8.5 to 56.1).48
myocardial energy supply in the occurrence of cardiac death in these patients.47 In a prospective study of a larger group (N = 318) of asymptomatic hemodialysis patients without a clinical history of MI or coronary revascularization who were followed for 3.6 ± 1 year, a BMIPP score > 12 was again strongly associated with cardiac death (hazard ratio [HR]: 22; 95% confidence interval [CI] = 8.5 to 56.1).48
Arteriosclerosis
Increased CV morbidity and mortality have been correlated, either directly or indirectly, with various estimates of elevated LV afterload in patients with CKD.49,50,51 Postulated etiologic factors include increased arterial wall stiffness,49 raised sympathetic tone mediated by elevated noradrenaline levels,52,53 cardiac natriuretic peptides,54 nocturnal hypoxemia,55 and autonomic dysregulation.56 These factors are associated with two established components of LV afterload: hypertension and reduced arterial wall compliance. Before examining these, however, it is important first to recognize the pathophysiologic similarities and differences by which they are characterized.
Hypertension has usually been attributed to a reduction in the caliber or number of muscular arteries (150 to 400 µm diameter), resulting in an increase in peripheral resistance. This approach, however, does not take into account the fact that BP fluctuates during the cardiac cycle, and that systolic and diastolic levels represent only the limits of this fluctuation. A Fourier analysis of the BP curve can determine both its steady state (mean BP) and oscillatory (fluctuation about the mean) components. The former is determined exclusively by cardiac output and peripheral resistance (pressure and flow considered constant over time). The oscillatory component is determined by the pattern of LV ejection, the viscoelastic properties of large conduit arteries, and the reflection of pulse waves.57 A faster pulse wave velocity (PWV) is primarily associated with arterial stiffness, which, in CKD, is an acceleration of the normal aging process with vessel dilatation and a diffuse, nonocclusive medial and intimal wall hypertrophy (arteriosclerosis). It has been correlated with shortened stature, male gender, smoking, BP, diabetes, volume overload, humoral imbalance, and age.58,59,60
The clinical characteristics of hypertension therefore will depend on the predominant abnormality. Increased peripheral resistance is characterized principally by an increased diastolic and mean BP, whereas increased arterial stiffness and early wave reflections are indicated by an increased systolic and widened pulse pressure. Because the peripheral resistance of most dialysis patients is within the normal range, it is likely that effects from an accelerated PWV contribute more significantly to CV morbidity than an elevation in mean BP. Increased systolic BP and pulse pressure are observed in dialysis patients and are closely correlated with LV hypertrophy.61,62
Vascular calcification. An increased calcium X phosphate product, elevated levels of promoters of calcification, and reduced levels of inhibitors of calcification, in addition to hyperparathyroidism, insulin resistance, oxidant stresses, and dyslipidemia, promote metastatic vascular calcification and heart fibrosis in patients with ESRD.63 Multiple inhibitors of vascular calcification have been identified, the transcription and synthesis of which are downregulated during inflammation. A study in a mouse model revealed that fetuin-A, an inhibitor of vascular calcification, protects against vascular calcification in CKD, suggesting that the patients who develop calcification might be those with inflammation and low levels of circulating inhibitors of vascular calcification.64
Vascular smooth muscle cells have the ability to transform into cells with chondrocytelike or osteoblastlike phenotypes, the consequences of which include the local production of collagen and noncollagenous proteins in intimal and medial layers, the incorporation of calcium and phosphorus into matrix vesicles, and vascular mineralization. This active process is associated with CKD, aging, diabetes, and inflammation.63
The cellular origin of medial vascular calcifications is suggested by ultrastructural analysis of the iliac arteries in 30 dialysis patients, obtained before renal transplantation, which demonstrated that arterial microcalcifications seem to originate from nanocrystals, and they often exhibit a coreshell structure.65
Two types of vascular calcification occur: patchy calcification of the intima associated with atherosclerotic plaques and diffuse calcification of the media, in the absence of cholesterol deposits, associated with arteriosclerosis. Metastatic vascular calcification decreases compliance of large conduit vessels and thus increases PWV. An early rebound of pressure waves from the distal vessels increases systolic pressure and predisposes the patient to LVH, and low diastolic pressure predisposes the patient to diminished coronary flow during diastole.
Controversy exists as to whether these are two distinct entities in CKD or rather are a continuum of advanced vascular pathology consistent with accelerated atherosclerotic calcification.66,67 Coronary artery calcification scoring by computed tomography is used to estimate the likelihood of coronary artery disease in the general population and is a predictor of all-cause mortality. In 225 diabetic patients with proteinuria, coronary artery calcification was diagnosed in 86% of the patients, and it was not associated with eGFR, serum calcium, phosphate, parathyroid hormone, or 25-hydroxy vitamin D.69 This suggests that the coronary calcification was associated with atherosclerotic disease rather than medial calcification induced by CKD. The severity of coronary artery calcification early in the course of CKD was an independent predictor of all-cause mortality.68
A recent autopsy study69 of patients at different stages of CKD reported the occurrence of coronary intimal sclerosis in all CKD stages, but medial sclerosis in patients with stage 4 and 5 CKD (18%). Moreover, medial calcification was generally associated with intimal sclerosis, which is supportive
of the belief that medial calcification is an amplification of preexisting atherosclerosis.
of the belief that medial calcification is an amplification of preexisting atherosclerosis.
Cardiomyopathy
Left ventricular hypertrophy. Ventricular growth occurs in response to mechanical stresses, primarily volume or pressure overload (Fig. 79.5). LV volume overload results in the addition of new sarcomeres in series, leading to an increased cavity diameter.70 A larger diameter results in increased wall tension, a direct consequence of the Laplace law, which states that all wall tension (T) is proportional to the product of intraventricular pressure (P) multiplied by the ventricular diameter (D), divided by the wall thickness (M), or T = PD/M. An increase in wall tension secondarily stimulates the addition of new sarcomeres in parallel. This remodeling thickens the ventricular wall, distributing the tension over a larger cross-sectional area of muscle and returning the tension in each individual fiber back to normal, thereby alleviating the stimulus to further hypertrophy. This combination of cavity enlargement and wall thickening is called eccentric hypertrophy. Pressure overload increases wall tension by increasing the intraventricular pressure, resulting directly in the parallel addition of new sarcomeres and their functional consequences, as described. Because sarcomeres are not added in series, isolated pressure overload does not lead to cavity enlargement (concentric hypertrophy).
![]() FIGURE 79.5 The evolution of cardiomyopathy in chronic kidney disease. A-V, atrioventricular; LV, left ventricular; Art Dis, artery disease; Dis, disease. |
Both eccentric and concentric hypertrophies are initially beneficial. Dilation permits an increase in stroke volume without an increase in the inotropic state of the myocardium and, as such, is an efficient adaptation to volume overload.70 It also permits the maintenance of normal stroke volume and cardiac output in the presence of decreased contractility. Muscular hypertrophy returns the tension per unit muscle fiber back to normal, thereby decreasing ventricular stress.
The uremic milieu of chronic renal disease can potentiate many of these processes. Anemia, salt and water excess, and arteriovenous fistulae in patients on dialysis are prevalent causes of volume overload, whereas hypertension is a major cause of pressure overload. These disturbances are probably the primary stimuli to ventricular remodeling in uremia. These same stimuli promote arterial remodeling in the large
and resistance arteries, which is characterized by diffuse arterial thickening and stiffening (arteriosclerosis), which can increase the effective load on the left ventricle independently of mean arterial pressure.71,72 Secondary hyperparathyroidism and raised calcium phosphate product may be associated with aortic valve calcification and, in some cases, stenosis, which is a less frequent cause of pressure overload.73
and resistance arteries, which is characterized by diffuse arterial thickening and stiffening (arteriosclerosis), which can increase the effective load on the left ventricle independently of mean arterial pressure.71,72 Secondary hyperparathyroidism and raised calcium phosphate product may be associated with aortic valve calcification and, in some cases, stenosis, which is a less frequent cause of pressure overload.73
Progressive concentric LVH and hyperkinesis occur in hemodialysis patients, which is partly explained by hypertension but not by a wide array of potential risk factors, including moderate anemia.19 Another explanation may be that primary stimuli independent of LV load might initiate or contribute to LVH and fibrosis in patients with CKD. Several signaling pathways could have a role in this process, including defects in insulin signaling through the protein kinase, Akt, and downstream, the mammalian target of rapamycin (mTOR) pathway.74 Siedlecki et al.75 created normotensive CKD using a mouse model in which kidney parenchyma was resected so as to avoid excessive renin-angiotensin system (RAS) activation. Progressive LVH and fibrosis developed, which was associated with de novo protein synthesis and activation of the mTOR pathway. The administration of rapamycin, which inhibits the mTOR pathway, prevented cardiac hypertrophy.
Myocyte death. Ultimately, in persistent LV overload, LVH becomes maladaptive (Fig. 79.5). Muscular hypertrophy is associated with several progressive, deleterious changes in cell function and tissue architecture. Early in the evolution of LV hypertrophy, slow reuptake of calcium by the sarcoplasmic reticulum leads to abnormal ventricular relaxation. Combined with decreased passive compliance of a thickened ventricular wall, these changes may precipitate diastolic dysfunction.76 More advanced sarcoplasmic reticulum dysfunction is associated with calcium overload and cell death. Decreased capillary density, impaired coronary reserve, and abnormal relaxation may decrease subendocardial perfusion, promoting ischemia.25,77 The frequent coexistence of CAD may exacerbate ischemia and myocyte attrition. Fibrosis of the cardiac interstitium also occurs and appears to be more marked in pressure than in volume overload.77 Myocyte apoptosis, ischemia, and neurohormonal activation (e.g., increased catecholamines, angiotensin II, and aldosterone) are thought to contribute to myocardial dysfunction.78,79 In the late phases of chronic and sustained overload, oxidative stress may contribute to cellular dysfunction and demise.35 Together, these various processes lead to progressive cellular attrition, fibrosis, pump failure, and, ultimately, death.
The attrition of myocytes in chronic uremia may be exacerbated by several factors. An underlying coronary artery disease promotes ischemia and infarction. Hyperparathyroidism increases susceptibility to ischemia through dysregulation of the cellular energy metabolism,46 and appears to promote myocardial fibrosis directly.80 Malnutrition, oxidative stress, and inadequate dialysis may additionally promote myocyte death. Such cell death in the presence of LVH and continuing pressure and volume overload may be catastrophic, leading to a severe overload cardiomyopathy and, ultimately, death.81
Pathologic studies support this etiologic pathway. Cardiac pathology in patients who had diabetes, heart failure, and no evidence of coronary artery disease on angiography revealed cell loss as a result of apoptosis and necrosis of cardiac myocytes and endothelial cells, with the extent of fibrosis, apoptosis, and hypertrophy all greater in hypertensive patients than in those without hypertension.82 Myocardial biopsies of dialysis patients with dilated cardiomyopathy revealed abnormal cardiac myocyte anatomy and interposition of dense fibrosis.83 The extent of myocardial fibrosis in patients with ESRD was more marked than in patients with diabetes mellitus or essential hypertension with similar LV mass.84
Myocardial stunning. Another cardiopathic mechanism that may predispose to a patient arrhythmia or to heart failure is recurrent reperfusion injury. The coronary arteries of dialysis patients are often narrowed, the microcirculation is often underdeveloped, and the LV may be hypertrophied. In this scenario, transient hypoperfusion could produce severe ischemia, followed by reperfusion necrosis.85 The frequent, although minor elevations in troponin observed in ESRD patients may reflect this myocardial damage. A phenomenon called myocardial stunning supports this hypothesis.
Myocardial stunning has been defined as a 20% reduction in regional wall motion in two or more segments and hyperkinesis as either a > 20% or a > 50% increase in shortening fraction. This was measured using echocardiography before hemodialysis, during hemodialysis, and 15 minutes after hemodialysis in 12 children without structural cardiac disease. Eleven of the 12 developed myocardial stunning with varying degrees of compensatory hyperkinesis in unaffected segments, thus maintaining LV ejection fraction throughout dialysis.86 This regional hypokinesis is associated with reduced myocardial blood flow. Four patients without coronary artery disease had myocardial blood measured during dialysis using serial intradialytic H215O positron emission tomography scanning and had their regional wall motion assessed using serial concurrent echocardiography.87 Segmental myocardial blood flow was reduced to a significantly greater extent in areas that developed regional wall motion abnormalities compared with those that did not.
Valvular Disease
Most valvular lesions observed in patients with CKD are acquired and develop from dystrophic calcification of the valvular annulus and leaflets, particularly the aortic and mitral valves.88 The prevalence of aortic valve calcification in dialysis patients is up to 55%, similar to that in the elderly general population, although it occurs 10 to 20 years earlier.88,89 Aortic valve orifice stenosis in CKD evolves from valve sclerosis, which itself is now generally recognized to be associated with an increased cardiovascular mortality.90 In dialysis patients, the prevalence of aortic stenosis is 3% to 13%.45 It
may sometimes evolve rapidly (within 6 months) to hemodynamically significant stenosis, with a worsening of LVH and rapidly evolving symptoms. Age, duration of dialysis, a raised phosphate level, and an elevated calcium phosphate product appear to be the most important risk factors for the development of aortic stenosis.88
may sometimes evolve rapidly (within 6 months) to hemodynamically significant stenosis, with a worsening of LVH and rapidly evolving symptoms. Age, duration of dialysis, a raised phosphate level, and an elevated calcium phosphate product appear to be the most important risk factors for the development of aortic stenosis.88
Mitral valve calcification is not as common as aortic valve disease in CKD and may have a somewhat different pathophysiology. In one study88 evaluated by echocardiography, mitral annulus calcification was present in 45% of 92 hemodialysis patients (compared to 10% of age-and gender-matched controls). In a study91 of 135 peritoneal dialysis patients with low parathyroid hormone levels, a constant involvement of the posterior cusp, together with left atrial enlargement, was observed. Valve calcification has also been associated with rhythm and cardiac conduction defects, valvular insufficiency, and peripheral vascular calcification. Although most studies have identified abnormalities in calcium phosphate metabolism as the predominant underlying risk factor, additional factors include the duration of dialysis and the duration of predialysis systolic hypertension. Factors associated with decreased survival include the severity of calcification, mitral regurgitation, and reduced LV function.92
Diagnosis
Coronary Artery Disease
Symptoms of myocardial ischemia in patients with CKD are, in general, similar to those in the nonuremic population. However, the prevalence of silent myocardial ischemia in this group of patients is very high.93,94 This has been best demonstrated in diabetic patients with ESRD because they often are subjected to a screening coronary angiography before renal transplantation. In one series95 of 100 diabetic patients with ESRD, for example, 75% of the patients with angiographically demonstrated CAD had no typical angina symptoms. The prevalence of asymptomatic CAD in nondiabetic patients with ESRD is not as well studied. Exertional dyspnea is also less specific for cardiac disease in patients with CKD than in the general population. Such symptoms may be attributable to anemia, acidosis, heart failure, or fluid overload; therefore, a careful interpretation within the clinical context is required.
Patients with CKD and symptomatic ischemic heart disease (IHD) should be investigated in a manner similar to patients with normal renal function, provided that revascularization will be considered should critical coronary artery disease (CAD) be identified. Routine screening for CAD in asymptomatic patients with CKD therefore is not recommended.96 It is appropriate to apply clinical practice guidelines for a CAD screening of patients before noncardiac surgery to the CKD population as well.97 Generally, CAD screening is recommended before surgery when the combination of risk factors and the nature of the operation place the patient at moderate or higher risk of a cardiovascular event. Patients being evaluated for renal transplantation are an exception to the aforementioned recommendations. The adverse prognostic implications of CAD, the desire to avoid allograft injury from posttransplantation invasive cardiac testing, and the need to ration transplantable organs are justification for screening all but the lower risk patients. The American Society of Transplant Physicians has published guidelines for the evaluation of renal transplantation candidates that include recommendations for the investigation of CAD.98
Biomarkers for ischemic heart disease. Cardiac troponin T and troponin I are currently the standard biomarkers of myocardial injury. These tests are more specific for myocardial damage than creatinine kinase-myocardial band but still are not always indicative of an ischemic mechanism of injury.99 First-generation troponin T levels in ESRD patients are often high, whereas troponin I levels are high less frequently.100 Although there is no difference between the diagnostic and prognostic accuracy of troponin T versus troponin I in the general population in suspected acute coronary syndrome,101 the lower incidence of increased troponin I in the renal failure population suggests that this is the preferred test in this clinical setting.100
Despite concerns about falsely positive tests, troponins are clearly very useful in the evaluation of suspected ACS. In one retrospective analysis,102 troponin I was shown to be the best performing marker in suspected acute coronary syndrome (ACS) in patients with CKD or ESRD when compared to alternatives such as creatine kinase or myoglobin. Although troponin T is more likely to be elevated at baseline in patients with renal impairment, a normal level has useful negative predictive value. A sequential rise in either of the serum troponins is consistent with new myocardial damage regardless of symptoms.103
Noninvasive testing for coronary artery disease. Exercise electrocardiography (ECG) has been the traditional method of a noninvasive diagnosis of CAD. The sensitivity of this test is only 50% to 60% for single vessel disease but is greater than 85% for triple vessel CAD in the general population.104 These figures are based on the assumption that the patient reaches an adequate exercise level (i.e., 85% of the age-adjusted predicted maximal heart rate). A large proportion of patients with ESRD are unable to achieve this target because of poor exercise tolerance, anemia, poorly controlled hypertension, or the use of cardiac medications. One study105 of 85 diabetic uremic patients showed that only 6 achieved an adequate exercise level. Pharmacologic agents, therefore, often are used for noninvasive testing for CAD in these patients. The diagnostic utility of dipyridamole-thallium testing in patients with ESRD is poor,106 whereas that of dobutamine stress echocardiography is better,107 and therefore may be the method of choice where it is available.
Nuclear scintigraphic scanning. Nuclear scintigraphy can be used both for the assessment of myocardial systolic function and for ischemia. The former method examines the
ejection fraction of the left and/or right ventricles and relies upon gated analysis techniques. Care must be taken with regard to associated valve regurgitation, which when present, can substantially confound functional estimates. If valve function is intact, accurate estimates of systolic function at rest and with exercise can be achieved.
ejection fraction of the left and/or right ventricles and relies upon gated analysis techniques. Care must be taken with regard to associated valve regurgitation, which when present, can substantially confound functional estimates. If valve function is intact, accurate estimates of systolic function at rest and with exercise can be achieved.
The predominant role for nuclear scanning techniques, however, is in the assessment of myocardial ischemia, both as a screening tool in the work-up for transplantation and in cases of diagnostic uncertainty. Exercise-based studies as well as the use of dipyridamole to enhance vasodilation are commonly used, together with one or other of technetium-99m (99mTc)-labeled thallium, methoxyisobutylisonitrile (MIBI), or metaiodobenzylguanidine (MIBG). Inherent problems with scintigraphy must be taken into consideration. BP may be too high or too low to permit the safe administration of a vasodilatory agent; high endogenous circulating levels of adenosine may blunt the efficacy of dipyridamole; coronary flow reserve may be reduced due to LV hypertrophy and small vessel disease; and symmetrical coronary disease and/or a blunted tachycardic response due to autonomic neuropathy can mask significant pathology.108,109
Dahan and colleagues110 found a positive and negative predictive value of 47% and 91%, respectively, for dipyridamole-thallium combined imaging in the diagnosis of coronary disease by coronary angiography in a study of 60 asymptomatic hemodialysis patients. It is likely that both on-site expertise and the recognized testing limitations in patients with CKD influence the utility of nuclear scanning and therefore dictate the interpretation and screening strategy for a particular center.
Computed tomography. Electron-beam ultrafast computed tomography-derived coronary artery calcification assessment relies upon the principle that coronary artery calcification is a reliable surrogate for significant coronary atherosclerosis, but this is far from certain in patients with CKD.66,67 It has been used recently to demonstrate a reduction in coronary artery calcification after treatment with the non-calcium-containing medication sevelamer and with cinacalcet.111,112 The role of this technique in evaluating risk or disease in transplant patients is unknown. Severe coronary artery calcification measured by electron beam-computed tomography in stage 3 through 5 CKD occurs much more frequently in diabetics (56%) than in nondiabetics (4%). In CKD stages 1 and 2, the prevalence was 10% in diabetics and 1% in nondiabetics.113
In the Multi-Ethnic Study of Atherosclerosis, 562 adults with eGFR < 60 mL per minute had assessments of coronary artery calcification.114 The prevalence of coronary artery calcification at baseline was 66%, and the incidence was 6.1% per year in women and 14.8% in men. Progression occurred in 17% of subjects per year across all subgroups, and diabetes was associated with a 65% adjusted risk of progression.
The accuracy and clinical utility of noninvasive testing for coronary artery disease was evaluated in 517 Dutch patients referred for an evaluation of chest pain symptoms, using coronary arteriography as the gold standard.115 Stress testing was sufficient as a diagnostic test for patients with a low pretest probability of coronary disease based on their Duke classification clinical score. Computed tomography coronary angiography was useful in patients with intermediate pretest probability, because it could distinguish which patients required invasive angiography. In patients with high pretest probability, proceeding directly to invasive angiography, without a noninvasive test evaluation, was recommended.
Coronary angiography. Cardiac catheterization and coronary angiography remain the gold standard for the diagnosis of CAD. A major disadvantage associated with this mode of investigation is the potential for renal toxicity from radiocontrast agents. CKD, especially in diabetic patients, is a major risk factor for contrast-induced acute renal failure.116 The risk of clinical nephrotoxicity is related to the severity of prior renal impairment. Although most patients who develop nephropathy eventually recover renal function, there is a risk that contrast administration may precipitate ESRD in patients with severe impairment in renal function at baseline. The risk of contrast nephropathy in high-risk patients may be reduced by using nonionic contrast media and a saline infusion. Many interventions to reduce the risk of nephropathy have been studied, but none have been shown to be consistently effective.116 A number of trials using N-acetylcysteine in CKD patients have demonstrated a reduction in the incidence of contrast nephropathy, whereas others have not. A meta-analysis of this topic indicated that the relative risk of nephropathy is reduced by N-acetylcysteine (relative risk 0.65; 95% CI, 0.43 to 1.00), but there has been significant heterogeneity in studies to date.117 Given its low cost and lack of adverse effects, it is reasonable to use N-acetylcysteine in high-risk patients prior to angiography. A large trial with clinically meaningful outcomes is still required before it can be universally recommended.
Arteriosclerosis
Aortic PWV is the best available measure of aortic stiffness and correlates well with a subsequent risk for CVD.118
Cardiomyopathy
Because echocardiography is widely available, simple, and reproducible, it has become the method of choice for the assessment of LVH. Systolic dysfunction is defined as an ejection fraction of less than 40%, which indicates impaired myocardial contractility. It often is associated with LV dilation (LV end-diastolic diameter ≥ 5.6 cm), which is defined echocardiographically as an LV cavity volume index greater than 90 mL per square meter.119 Concentric LVH is characterized by a thickened LV wall (≥ 1.2 cm during diastole) with normal cavity volume. LV mass index is a calculated parameter that reflects the degree of muscular hypertrophy in the LV. Epidemiologic studies in nonrenal patients have
established that the upper limits of LV mass index are 130 g per square meter for adult men and 102 g per square meter for adult women.120 Values above these limits indicate hypertrophy. The calculation of LV mass and volume are not independent of volume status. As a result, patients should be euvolemic when the echocardiogram is performed. In patients on hemodialysis, it is important to standardize the time and conditions of the study in relation to the dialysis session and to have patients at their dry weight.
established that the upper limits of LV mass index are 130 g per square meter for adult men and 102 g per square meter for adult women.120 Values above these limits indicate hypertrophy. The calculation of LV mass and volume are not independent of volume status. As a result, patients should be euvolemic when the echocardiogram is performed. In patients on hemodialysis, it is important to standardize the time and conditions of the study in relation to the dialysis session and to have patients at their dry weight.
Cardiac magnetic resonance is a volume-independent technique to assess cardiac structure and LV mass. This technique is now used as a surrogate outcome measure in randomized controlled trials (RCTs).121 Of 246 Scottish hemodialysis patients, 64% had LV hypertrophy using this technique, and the principal associations of LV mass were end-diastolic volume, predialysis BP, and calcium X phosphate product.122
In the Cardiovascular Risk Reduction by Early Anemia Treatment with Epoetin Beta (CREATE) trial,123 in patients with stage 3 and 4 CKD, the prevalence of LV hypertrophy using echocardiography at baseline was 47%, with eccentric hypertrophy more prevalent than concentric. During the study, LVH prevalence and mean LV mass index did not increase significantly, but LV geometry fluctuated considerably within 2 years in both groups.
Levin et al.124 have reported a prevalence of LVH in 27% of patients with a creatinine clearance rate greater than 50 mL per minute, in 31% of those with clearances of 25 to 49 L per minute, and in 45% of those with clearances less than 25 mL per minute. In the prospective arm of this study, an association between rising LV mass index and falling GFR was observed. The overall prevalence of LVH among patients beginning dialysis was 75%.125 In a large prospective cohort study, only 16% had normal echocardiograms at inception. Fifteen percent (15%) had systolic dysfunction, 28% had dilation with preserved contractility, and 41% had concentric LVH.126 In a subset of these patients on dialysis who underwent yearly consecutive echocardiograms, LV mass index and LV cavity volume progressively increased, and the biggest increase occurred between baseline and 1 year.127 This progressive LV growth was confirmed in a recent prospective study.19
In patients about to undergo transplantation, the distribution of echocardiographic abnormalities is similar to those in patients on dialysis: normal, 17%; concentric LVH, 41%; dilation, 32%; and systolic dysfunction, 12%.128 In this longitudinal study, the proportion of patients with normal studies doubled (36%) and systolic function normalized in all patients with fractional shortening of less than 25% at 1 year posttransplantation.128
Clinical Manifestations
Myocardial Infarction or Angina
The most frequent manifestations of ischemic heart disease are MI and angina. The incidence rate of atherosclerotic events (MI, CV events, peripheral vascular disease) in predialysis CKD is very high. In a Medicare population of nondiabetic CKD patients, it was 36 per 100 patient years, and in diabetic CKD, it was 49 per 100 patient years.11
In Canada, a prospective study12 demonstrated that by the time patients reach ESRD, the prevalence of angina was 21% and of patients who had had a MI, it was 18%. The annual incidence of angina or MI among Canadian patients on hemodialysis is 10%.129 Among renal transplant recipients, the annual incidence of MI, revascularization, or death from MI was 1.5%.130 In another study, the rate of de novo angina or MI was 1.22 per 100 patient years.13
Both traditional and nontraditional risk factors predict coronary heart disease in CKD. Results from the atherosclerosis risk in communities (ARIC) study131 identified that independent of age, gender, and diabetes the following are risk factors: smoking, hypertension, hyperglycemia, and hypercholesterolemia. In addition to these traditional risk factors, nontraditional risk factors identified were increased waist circumference, hyperlipoproteinemia B, anemia, hypoalbuminemia, and hyperfibrinogenemia (Table 79.1).131
TABLE 79.1 Traditional and Nontraditional Risk Factors as Predictors of Coronary Artery Disease in Chronic Kidney Disease (Results from the ARIC study)131 | ||||||||||||||||||||||||||||||||||||||||||||||||
---|---|---|---|---|---|---|---|---|---|---|---|---|---|---|---|---|---|---|---|---|---|---|---|---|---|---|---|---|---|---|---|---|---|---|---|---|---|---|---|---|---|---|---|---|---|---|---|---|
|
In an incident cohort of dialysis patients, independent predictors of de novo ischemic heart disease events were diabetes, hypertension, and hypoalbuminemia.132 In an incident cohort of renal transplant recipients,13 the major predictors were diabetes and hypertension, and in a more recent cohort study,133 hypercholesterolemia was identified as a predictor of cardiac events.
Congestive Heart Failure
Congestive heart failure (CHF) may result from systolic dysfunction or diastolic dysfunction,21 the latter occurring because of concentric or eccentric hypertrophy (Fig. 79.2). IHD is an additional independent predictor. Among patients with diastolic dysfunction, CHF results from impaired ventricular relaxation; this leads to an exaggerated increase in LV end-diastolic pressure for a given increase in end-diastolic volume. As a result, a small excess of salt and water can rapidly lead to a large increase in LV end-diastolic pressure, culminating in pulmonary edema. The development of CHF, even in the presence of salt and water overload, suggests an underlying cardiac abnormality. Because the management of diastolic dysfunction differs from that of systolic dysfunction, an echocardiogram of the left ventricle is useful in planning management.
Approximately half of patients with CHF have CKD, as defined by a GFR ≤ 60 mL per minute.134 Differentiating type II chronic cardiorenal syndrome from type IV chronic cardiorenal syndrome may be difficult, because chronic heart failure may cause CKD, and CKD predisposes a patient to heart failure. In predialysis, CKD heart failure events occur as frequently as atherosclerotic events, particularly in patients with diabetic nephropathy; in nondiabetic CKD patients, the event rate was 31 per 100 patient years and in diabetic CKD patients it was 52 per 100 patient years.11
On starting dialysis, 35% of patients have had a previous episode of CHF.12 A baseline history of CHF carries a twofold risk of death independent of age, diabetes, and heart disease.125 The risk for the development of pulmonary edema requiring hospitalization or ultrafiltration after starting maintenance hemodialysis is 10% annually.129 Among patients free of CHF at the initiation of dialysis, de novo CHF developed in 25% over 41 months of observation.15 In a cohort of 244 CKD patients, 20% developed new or worsening cardiac symptoms, including a change in their CHF symptoms.134 Renal transplant recipients who were free of cardiac disease 1 year after transplantation developed de novo CHF as frequently as de novo IHD (1.26 versus 1.22 events per 100 patient years, respectively).13
In an incident cohort of dialysis patients without a previous history of CHF, the significant predictors of the de novo development of CHF, independent of age, diabetes, and systolic dysfunction at baseline, were hypertension, anemia, hypoalbuminemia, and hypocalcemia.15 It appears that factors that predispose a patient to volume or flow overload (e.g., anemia), pressure overload (e.g., hypertension), and cell death (e.g., malnutrition, hypocalcemia/hyperparathyroidism, and IHD) are associated with CHF in dialysis.
The presence of concentric LV hypertrophy, LV dilation, or systolic dysfunction at the time of ESRD therapy has been associated with progressively higher risks of congestive heart failure independent of age, sex, diabetes, and ischemic heart disease.126 Furthermore, changes in echocardiographic measurements following the initiation of dialysis also predicted the development of heart failure.135
In a large cohort of renal transplant recipients (RTRs) without cardiac disease 1 year after transplantation, age, diabetes, gender, high BP, and anemia were identified as independent risk factors for de novo CHF.13
Arrhythmias
In patients without renal failure, LVH and CAD appear to be associated with an increased risk of arrhythmias. As outlined earlier, these cardiac diseases occur frequently in patients with CKD. In addition, serum electrolyte levels that can affect cardiac conduction, including potassium, calcium, magnesium, and hydrogen, are often abnormal or undergo rapid fluctuations during hemodialysis.
In cross-sectional studies, the prevalence of arrhythmias is high: between 68% and 88% for atrial arrhythmias, 56% to 76% for ventricular arrhythmias, and premature ventricular complexes were found in 14% to 21%.136,137 Older age, preexisting heart disease, LVH, and digoxin therapy were associated with a higher prevalence and a greater severity of cardiac arrhythmias. However, because of the considerable variation in the frequency and severity of arrhythmias during and after dialysis, the clinical significance in a given patient is unclear.
Most atrial arrhythmias are of low clinical significance. However, a sustained, rate-related (fast or slow) impairment of LV filling can certainly produce hemodynamic consequences. The majority of the premature ventricular contractions are unifocal and number less than 30 per hour. The finding of high-grade ventricular arrhythmias in the presence of CAD has been associated with an increased risk of cardiac mortality and sudden death.138 Dialysis-associated hypotension may precipitate high-grade ventricular arrhythmias.
Arrhythmias in peritoneal dialysis patients appear different from those in hemodialysis patients. Severe cardiac arrhythmias occurred in only 4% of 27 peritoneal dialysis patients compared to 33% of 27 hemodialysis patients.139 Patients in both groups were matched for age, sex, duration of treatment, and etiology of chronic renal failure. The lower frequency of LVH, the maintenance of a relatively stable BP, the absence of sudden hypotensive events, and the significantly lower incidence of hyperkalemia in patients on peritoneal dialysis may explain the lower incidence of severe arrhythmias.
Atrial Fibrillation
The prevalence of atrial fibrillation in ESRD is extremely high, and it is associated with increased mortality in hemodialysis patients.140 Of 17,513 randomly sampled patients in the
Dialysis Outcomes and Practice Patterns Study (DOPPS),141 2,188 (12.5%) had preexisting atrial fibrillation and the incidence of de novo atrial fibrillation during follow-up was 1 per 100 patient years. Advanced age, non-black race, higher facility mean dialysate calcium, prosthetic heart valves, and valvular heart disease were associated with a higher risk of de novo atrial fibrillation. The risk of ischemic stroke in the general population is best estimated with the CHADS2 score (1 point each for congestive heart failure, hypertension, age ≥ 75 years, and diabetes; 2 points for prior stroke or transient ischemic attack). The CHADS2 score identified approximately equal-sized groups of hemodialysis patients at low (< 2) and high risk (> 4) for subsequent strokes.
Dialysis Outcomes and Practice Patterns Study (DOPPS),141 2,188 (12.5%) had preexisting atrial fibrillation and the incidence of de novo atrial fibrillation during follow-up was 1 per 100 patient years. Advanced age, non-black race, higher facility mean dialysate calcium, prosthetic heart valves, and valvular heart disease were associated with a higher risk of de novo atrial fibrillation. The risk of ischemic stroke in the general population is best estimated with the CHADS2 score (1 point each for congestive heart failure, hypertension, age ≥ 75 years, and diabetes; 2 points for prior stroke or transient ischemic attack). The CHADS2 score identified approximately equal-sized groups of hemodialysis patients at low (< 2) and high risk (> 4) for subsequent strokes.
The prevalence of atrial fibrillation in predialysis CKD is also high and likely related to comorbidity rather than severity of CKD. Of 2010 nondialysis patients with CKD in two community hospitals in Chicago, 21% had atrial fibrillations.142 This was associated with older age, white race, increasing left atrial diameter, lower systolic BP, and congestive heart failure, but was not associated with eGFR.
Registry data in the United States revealed that the cumulative incidence of new onset atrial fibrillation was 3.6% at 12 months and 7.3% at 36 months after renal transplantation, and declined below the demographics adjusted cumulative incidence on the waiting list by about 17 months.143 Baseline independent predictors of atrial fibrillation included older recipient age, male gender, white race, renal failure from hypertension, and coronary artery disease. Transplant factors included donor age, delayed graft function, posttransplantation hypertension, anemia, new onset diabetes, MI, and graft failure.
Sudden Death
The proportion of deaths designed as sudden is similar in both patients with CKD and the general population. In a British community-based study,144 approximately 70% of deaths were the result of cardiac disease, and roughly half of those cardiovascular deaths were sudden. Among diabetic hemodialysis patients in the Die Deutsche Diabetes Dialysis (4D) study in 178 German dialysis centers,145 26% of adjudicated cardiac deaths were sudden, whereas coronary artery disease, heart failure, and other cardiac etiologies were the cause of 9%, 6%, and 3% of the adjudicated deaths, respectively. A study of 4,120 deaths in the United States during a 2-year follow-up of 12,833 prevalent hemodialysis patients showed that the greatest percentage of all deaths (27%) was caused by sudden cardiac arrest, whereas other cardiovascular conditions (including CAD, vascular heart disease, cardiomyopathy, arrhythmia, pericarditis/cardiac tamponade, and pulmonary edema) accounted for 20% of all deaths.146
A high rate of sudden cardiac death in 5,830 dialysis patients who underwent coronary artery bypass (CABG) surgery in the United States was reported by Herzog et al.147 All-cause and arrhythmias-related mortality were 290 and 76 deaths per 1,000 patient years, respectively. Deaths from sudden cardiac arrest or arrhythmia accounted for approximately 25% of all-cause deaths.
The rate of sudden cardiac death was also examined in 19,440 U.S. patients with CKD who had undergone cardiac catheterization at a single institution148: 522 sudden cardiac deaths occurred and 25% of cases had an eGFR < 60 mL/min/1.73 m2. The sudden cardiac death rate increased with increasing severity of CKD; the hazard ratio (HR) for each 10 mL/min/1.73 m2 decline in eGFR was 1.11 (95% CI = 1.06 to 1.7).
Among patients undergoing dialysis, the frequency of sudden cardiac death increased both with the duration of time that the patient had been undergoing dialysis and with the duration of time since their previous dialysis session, and was highest among individuals with diabetes.149,150 Furthermore, the ratio of observed to expected deaths was higher than expected in the first 12 hours after the initiation of the hemodialysis session, and increased as the time from start of the dialysis session exceeded 36 hours.150 The number of observed deaths was three times higher than expected in the period 60 to 72 hours after the start of the dialysis session. Other risk factors for sudden death included hospitalization within the past 30 days and a decrease in systolic BP of 30 mm Hg during hemodialysis.150 Patients with ESRD and diabetes have a higher risk of sudden death than nondiabetic ESRD patients, with an incidence of 20% within the first 2 years after the dialysis is initiated.151
Cardiac arrest. Four hundred cardiac arrests occurred in a total of 5,744,708 hemodialysis sessions, which is equivalent to a rate of 7 arrests per 100,000 hemodialysis sessions.149 In another cohort study of 295,913 incident dialysis patients surviving at least 1 year on dialysis, the rate of cardiac arrest was 93 events per 1,000 patient years at year 1, and 164 events per 1,000 patient years at year 4.152 Among patients with diabetes who were undergoing dialysis, the rate of cardiac arrest at year 1 was 110 events per 1,000 patient years, rising to 208 events per 1,000 patient years at year 4.152
The survival rates following cardiac arrest reported by Herzog152 were 32% at 30 days and 17% at 1 year after arrest, respectively, and dropped to 13% at year 1 in patients with diabetes. On the other hand, 60% of patients with ESRD who experienced cardiac arrest in the dialysis unit died within 48 hours of cardiac arrest; 13% of these deaths occurred while in dialysis units.149
Mechanisms of sudden death. Clearly, the adverse cardiomyopathic and vasculopathic milieu predisposes individuals with CKD to arrhythmias and conduction abnormalities, which are manifestations of cardiac disease associated with sudden cardiac death. This predisposition is likely exacerbated by electrolyte shifts, increased ultrafiltration volumes, divalent ion abnormalities, diabetes, and sympathetic overactivity, in addition to inflammation and possibly iron deposition (Fig. 79.6). Impaired baroreflex effectiveness and sensitivity, as well as obstructive sleep apnea, might also
contribute to the risk of sudden death. Each of these risk factors is discussed in greater detail in the following.
contribute to the risk of sudden death. Each of these risk factors is discussed in greater detail in the following.
Prolonged corrected QT (QTc) intervals in patients with CKD and ESRD usually result from inhomogeneity of both myocardial depolarization and repolarization that occurs secondary to LVH and intercardiomyocytic fibrosis. Measures of the temporal variability in myocardial repolarization include QT dispersion and QT variability index. The former is defined as the difference between the maximal and minimal QT intervals on a standard ECG (QTmax – QTmin) and is associated with an increased risk of ventricular arrhythmias and mortality in patients with congestive heart failure and in the general population.153 The latter is calculated as the logarithm of the ratio between the variances of the normalized QT and RR intervals. It can predict the subsequent risk of sudden cardiac death or ventricular arrhythmia in patients who present for electrophysiologic investigation.154
Left ventricular hypertrophy. LVH could predispose individuals to sudden death through the prolongation of the QTc interval or by increasing arrhythmogenesis. The QTc interval is substantially longer in hemodialysis patients than in those who have near normal kidney function, and is associated with several manifestations of uremic cardiomyopathy, including increased LV mass index and end-diastolic volume, and a reduced LV ejection fraction.155 In addition, more premature ventricular complexes (PVCs) occur during hemodialysis in patients with LVH compared with those without LVH.138
Ischemic heart disease. In the general population, coronary heart disease is an important cause of sudden death. In patients undergoing hemodialysis, CAD probably causes arrhythmogenesis because severe coronary stenosis is associated with the induction and lengthy persistence of ventricular arrhythmias during and after hemodialysis.156 Furthermore, the number of PVCs during and after hemodialysis is higher in patients with ischemic heart disease than in those without it.138,156
Novel markers of coronary ischemia can identify patients who are at a high risk of cardiac death. Ischemia modified albumin (IMA) is a novel biomarker of acute ischemia that has high sensitivity and moderate specificity.157,158 In 114 patients with ESRD, an IMA level of 95 KU per liter predicted all-cause mortality with a sensitivity and specificity of 76% and 74%, respectively, whereas an elevated cardiac troponin level ≥ 0.06 µg per liter predicted mortality with a sensitivity of 75% and a specificity of 72%.159 Cardiac mortality risk was increased sevenfold in patients with combined elevated IMA and cardiac troponin levels (odds ratio [OR] = 7.12; 95% CI, 4.14 to 10.12).
QT dispersion and variability. Elevated QT dispersion was evident in 20 hemodialysis patients and 20 patients treated with continuous ambulatory peritoneal dialysis, and was significantly higher than in healthy controls.160 The difference in QT dispersion rates between patients undergoing hemodialysis and those on chronic ambulatory peritonial dialysis (CAPD) was not statistically significant. In a retrospective cohort study of 147 adult patients undergoing dialysis, a QTc interval dispersion that occurred for longer than 74 ms was an independent predictor of all-cause mortality (relative risk [RR], 1.5; 95% CI, 1.2 to 2.0), and of cardiovascular mortality (RR, 1.6; 95% CI, 1.2 to 2.4).161
The QT variability index was increased by 47% in 163 patients with advanced CKD (43 individuals with stage
4 CKD, 67 patients undergoing hemodialysis, and 43 patients on CAPD) during a 30-minute rest period compared with 39 age-matched healthy controls.162 The variability index was similar in patients with stage 4 CKD and in those on dialysis, whereas it was higher in patients with diabetes compared with nondiabetic patients with renal failure. Furthermore, in a multiple linear regression analysis, a history of diabetes or CAD were the only independent predictors of the QT variability index in patients with advanced CKD. The elevated index in patients with advanced CKD was the result of both reduced RR interval variance (secondary to reduced autonomic control of heart rate) and increased QT interval variance.162
4 CKD, 67 patients undergoing hemodialysis, and 43 patients on CAPD) during a 30-minute rest period compared with 39 age-matched healthy controls.162 The variability index was similar in patients with stage 4 CKD and in those on dialysis, whereas it was higher in patients with diabetes compared with nondiabetic patients with renal failure. Furthermore, in a multiple linear regression analysis, a history of diabetes or CAD were the only independent predictors of the QT variability index in patients with advanced CKD. The elevated index in patients with advanced CKD was the result of both reduced RR interval variance (secondary to reduced autonomic control of heart rate) and increased QT interval variance.162
It should be noted that several drugs (such as typical and atypical antipsychotics, sotalol, and antiarrhythmic agents) could prolong cardiac repolarization (QT interval) and trigger torsades de pointes, and could increase the risk of sudden cardiac death in patients with CKD.163
Baroreflex effectiveness and sensitivity. Impaired arterial baroreflex function is associated with an increased risk of ventricular arrhythmia and sudden cardiac death.164 In healthy individuals, an appropriate baroreflex response is usually obtained in 25% of all systolic BP ramps during the day and in 15% of ramps during the night.165 The ability of the arterial baroreflex to preserve short-term BP homeostasis— defined as arterial baroreflex sensitivity—has been identified as a prognostic marker of cardiovascular mortality in patients with MI.166 The baroreflex effectiveness index is defined as the ratio between the number of systolic BP ramps, followed by baroreflex-mediated changes in heart rate, and the total number of systolic BP ramps during the recording period.167
In 216 patients with hypertension and stage 4 or 5 CKD, the baroreflex sensitivity was reduced by 51% and the baroreflex effectiveness index was reduced by 49% compared with age-matched healthy controls (n = 43).164 Although the treatment modality for renal failure had no effect on baroreflex sensitivity or effectiveness, patients with CKD and diabetes had a greater reduction in both baroreflex sensitivity and effectiveness than patients with CKD who did not have diabetes. During the 41-month follow-up period, 69 patients with hypertension died.167 Sudden cardiac death occurred in 15 of these patients (22% of all deaths). The reduced baroreflex effectiveness index was an independent predictor of all-cause mortality, whereas reduced baroreflex sensitivity was an independent predictor of sudden cardiac death.167
Inflammation. Inflammation has been associated with sudden cardiac death independently of traditional cardiovascular risk factors.168 After adjusting for demographic characteristics, comorbidities, and laboratory factors, the highest tertiles of the inflammatory markers C-reactive protein (CRP) and interleukin 6 (IL-6) were associated with a doubled risk of sudden cardiac death compared with the lowest tertiles, whereas a decrease in serum albumin level was associated with a 1.35-fold increased risk of sudden cardiac death in the highest tertile compared with the lowest tertile.169 Inflammation could trigger sudden cardiac death through premature atherosclerosis and cytokine-induced plaque instability or by a direct effect on the myocardium and the electrical conduction system.
Impact of the hemodialysis prescription. A rapid change in the extracellular concentration of electrolytes during a dialysis session leads to a secondary shift of electrolytes between the intracellular and extracellular milieu, which depends on the electrochemical gradient. As a result, cellular membrane polarization and stability may be affected. Data from patients treated at Fresenius Medical Care North America-affiliated centers showed that dialysis with a potassium dialysate concentration of zero or 1 mmol per liter was a significant risk factor for cardiac arrest.149 This prescription had been used in 17.1% of patients who experienced a cardiac arrest compared with 8.8% of controls (P < .0001).149 Cardiac arrests were more frequent during dialysis sessions carried out on a Monday as compared to Wednesday (P = .001) and Friday (P = .004). Although the mechanism for this observation was not studied, it may be due to increased fluctuations in potassium concentrations or perhaps increased ultrafiltration volumes.
Further evidence to support modifiable risk factors associated with the hemodialysis prescription causing sudden cardiac arrest has been reported recently. From the DaVita/Gambro Healthcare database of 43,200 outpatient dialysis patients, 502 patients who experienced a sudden cardiac arrest were compared to 1,632 age-and dialysis vintage-matched controls.169 Independent factors associated with sudden cardiac arrest included low potassium dialysate < 2 mEq per liter, increased ultrafiltration volume, exposure to low calcium dialysate, and low serum creatinine levels. This study suggests that modification of the hemodialysis prescription may reduce the risk of sudden cardiac arrest, although the case-control research design is of low evidentiary power.
Evidence to support increased ultrafiltration volume as a CV risk factor was reported in a cohort of 1,846 patients. Rates over 13 mL/hr/kg, compared to ultrafiltration rates up to 10 mL/hr/kg, were significantly associated with all-cause and CV-related mortality (adjusted hazard ratio = 1.59 and 1.71, respectively).170 In the subset of patients with congestive heart failure, ultrafiltration rates between 10 and 13 mL/hr/kg were also significantly associated with all-cause mortality.
In addition to low potassium dialysate, a decreasing potassium profile during hemodialysis may be harmful; complex arrhythmias were observed more frequently during and after hemodialysis in those with a decreasing potassium profile compared with individuals whose potassium levels were kept constant (2.5 mmol per liter).171
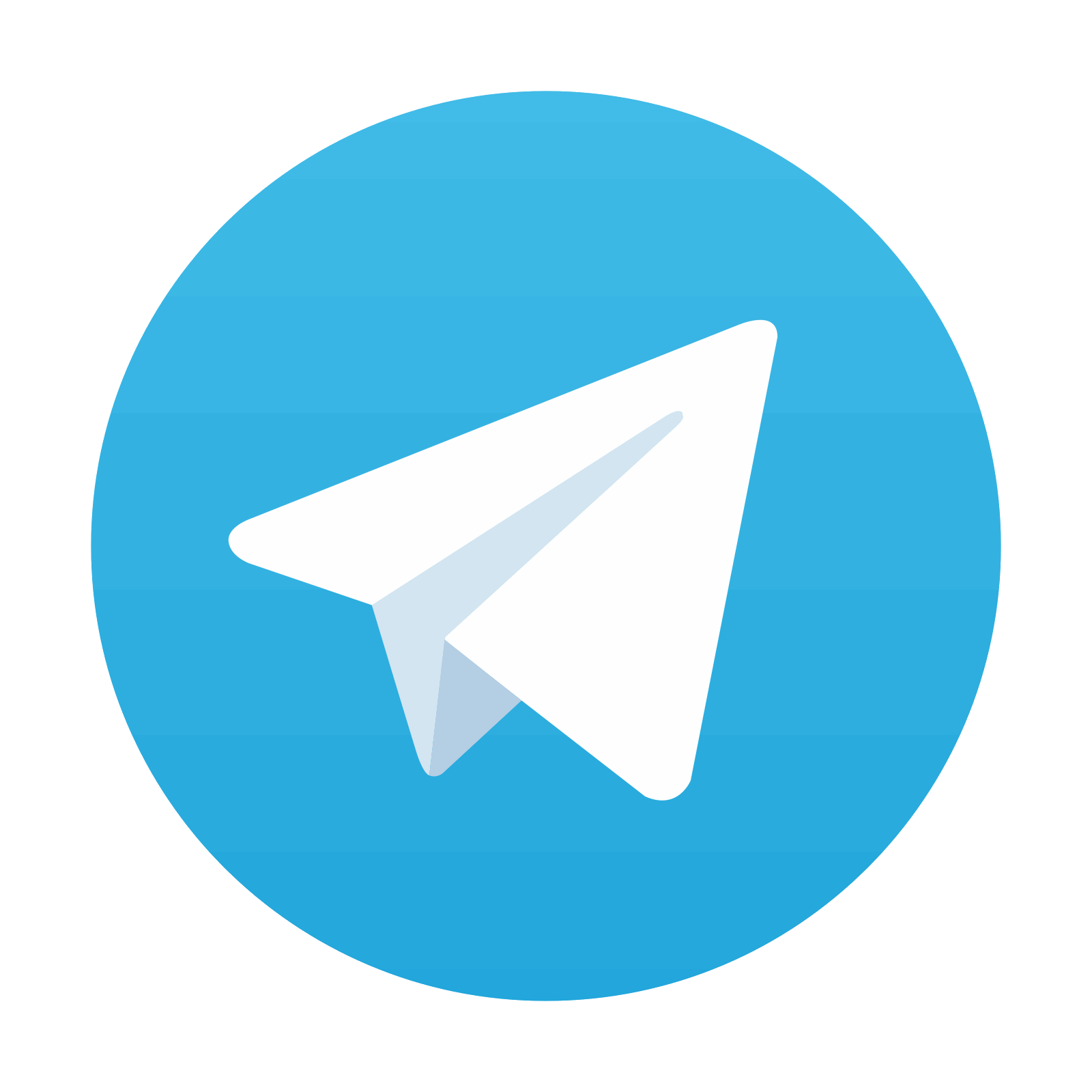
Stay updated, free articles. Join our Telegram channel

Full access? Get Clinical Tree
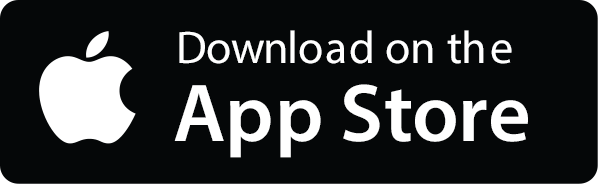
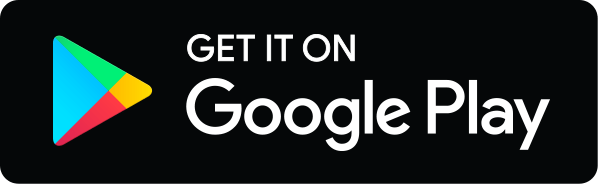
