The SLC4 ( s o l ute c arrier 4) family is a group of a membrane proteins that share sequence homology and in general mediate the transport of bicarbonate. It should be noted that bicarbonate transport is not unique to the SLC4 family. The structurally unrelated SLC26 family has at least three proteins that mediate CI − HCO − 3 exchange. In this chapter, the biology of SLC4 Na + -dependent bicarbonate transporters will be highlighted. A word of caution: whether SLC4 transporters mediate bicarbonate and/or carbonate transport is unknown and therefore when the word bicarbonate or the chemical symbol HCO − 3 is used throughout the chapter with reference to SLC4 mediated ion transport, it refers to both HCO − 3 and/or CO 2 3 . Interestingly, the outlier in the SLC4 family is the SLC4A11 gene product (NaBC1) that lacks the ability to transport bicarbonate and is currently characterized as an electrogenic sodium-borate transporter.
In this chapter, while extra-renal studies will be highlighted, an emphasis will be placed on the biology of specific sodium-coupled SLC4 proteins in the kidney.
Keywords
SLC4, bicarbonate, kidney, renal tubular acidosis, sodium, transport, acid–base, pH
CA 2+ Channels in the KIDNEY
The kidney plays an eminent role in the maintenance of the Ca 2+ balance by regulating the Ca 2+ excretion of the body. Renal epithelial cells are unique in their ability to mediate vectorial transport of Ca 2+ between the luminal and basolateral compartments. The initial step in this process is the Ca 2+ influx across the luminal membrane. In addition, Ca 2+ influx is crucial to facilitate Ca 2+ -dependent signal transduction processes in the renal cell. In general, Ca 2+ is postulated to enter the epithelial cell via Ca 2+ -permeable channels present at the plasma membrane under the influence of a steep inwardly-directed electrochemical gradient. In the last decade a series of cation channels, including voltage-dependent and independent channels, has been identified that could facilitate the entry of Ca 2+ ions in the epithelial cells of the kidney.
Voltage-Dependent Ca 2+ Channels
Voltage-dependent Ca 2+ channels have been well characterized in excitable tissues and at least four different types including, L, N, T and P, can be distinguished on the basis of electrophysiological and pharmacological properties. The molecular identity and precise localization of these Ca 2+ channels along the nephron is not well understood. Previous studies from Yu and colleagues have demonstrated the existence of voltage-dependent Ca 2+ channels in the kidney ( Table 62.1 ). They identified a gene transcript encoding the pore-forming alpha 1-subunit of a Ca 2+ channel (alpha 1A) in the glomerulus and the distal convoluted tubule (DCT). Skott and coworkers assessed the distribution of the identified alpha(1G)-subunit encoding a voltage-dependent Ca 2+ channel with T-type characteristics. RT-PCR analysis of microdissected rat nephron segments revealed alpha(1G) expression in DCT, CNT, cortical collecting duct (CCD), and inner medullary collecting duct (IMCD) ( Table 62.1 ). Immunohistochemistry on sections of rat kidneys using an anti-alpha(1G) antibody demonstrated labeling at the apical plasma membrane domains of DCT, CNT and IMCD principal cells. Another study from Bacskai and Friedman examined the parathyroid hormone (PTH)-sensitivity of Ca 2+ influx in single cultured cells originating from distal renal tubules by measuring the intracellular Ca 2+ concentration. They found that PTH activates dihydropyridine-sensitive Ca 2+ channels. Tan and Lau, described a 25 pS, cAMP-sensitive apical Ca 2+ channel in rabbit kidney CNT cells, that was inhibited by dihydropyridine agonists and depolarization. The properties of these Ca 2+ channels are largely conferred by their pore-forming alpha1 subunit. In general, voltage-sensitive Ca 2+ channels are heteromeric, multi-subunit proteins, as has been clearly demonstrated for the skeletal muscle and brain channels, and their properties are not only determined by the pore-forming alpha1-subunit itself, but also depend on the presence of regulatory beta subunits. To identify the accessory beta subunit of DCT/CNT Ca 2+ channels, degenerate primers based on published beta subunit sequences were used to amplify rat kidney cDNA by the polymerase chain reaction (PCR). Alternatively, spliced transcripts of three beta-subunit genes (beta-2,3,4) were identified. Northern blot analysis indicated that beta 4-subunit is preferentially expressed in kidney cortex. Transcripts of all three beta-subunit genes were detected by PCR in microdissected nephron segments, but only the beta 4-subunit was found in DCT/CNT. At present, the physiological significance of these voltage-dependent Ca 2+ channels in transepithelial Ca 2+ transport remains unclear. Functional Ca 2+ influx measurements were only performed in non-polarized single cell systems. In addition, pharmacological studies in polarized primary cultures of rabbit CNT and CCD have demonstrated that voltage-dependent Ca 2+ channel blockers do not inhibit transepithelial Ca 2+ transport. Additional studies are needed to address the functional relevance of voltage-dependent Ca 2+ channels in the process of transepithelial Ca 2+ transport in the kidney.
Channel Isotype | Selectivity pCa/pNa | Segmental distribution | |
---|---|---|---|
Voltage-dependent Ca 2+ channels | Alpha 1A | ~1000 | G, DCT |
Alpha 1G | ~1000 | DCT, CNT, CD | |
Other Ca 2+ permeable channels | TRPC1 | 1 | G, PT, TAL, RV |
TRPC3 | 1.5 | G, CD, RV | |
TRPC4 | 1.1; 7 | G, RV | |
TRPC5 | 1.8; 9 | G, RV | |
TRPC6 | 5 | G, CD, RV | |
TRPV4 | 6 | ATL, TAL, DCT, CNT | |
TRPV5 | 107 | DCT, CNT | |
TRPV6 | 130 | DCT, CNT, CD | |
TRPM3 | 1.6 | CNT, CD | |
TRPM6 | 7 | DCT | |
TRPP2 | 5 | DCT, CD |
Other Ca 2+ Channels
Non-voltage-gated Ca 2+ channels in the kidney are mainly represented by the transient receptor potential (TRP) superfamily of proteins consisting of Ca 2+ permeable channels. TRP channels can be divided by sequence homology into at least six subfamilies, designated TRPC (canonical), TRPV (vanilloid), TRPM (melastatin), TRPP (polycystins, PKD-type), TRPA (ankyrin), TRPML (mucolipin), and the more distant subfamily TRPN (no mechano-receptor potential or nomp). Mammalian homologues of the first identified Drosophila TRP gene encode a family of at least 20 ion channel proteins ( Figure 62.1 ). Mutations and/or dysfunction of TRP proteins produce many renal diseases, including Mg 2+ wasting, Ca 2+ -related disorders and polycystic kidney diseases.

TRPC Channels
TRP channels are widely expressed and facilitate diverse functions, ranging from thermal, tactile, taste, osmolar, and fluid flow sensing to transepithelial ion transport. TRPC1 was the first member of the mammalian TRP family purported to form an ion channel. TRPC channels are ubiquitously expressed in mammalian tissues. TRPC1, 3, 4, 5 and 6 mRNA and protein expression was detected in freshly isolated rat renal resistance vessels and glomeruli suggesting that these channels may play a role in mediating voltage-independent Ca 2+ entry to facilitate cellular signal transduction processes. It should be noted that members of the TRPC family are non-selective divalent ion channels ( Table 62.1 ). Interestingly, TRPC1 dysfunction might be important in the development of diabetic nephropathy while TRPC6 seems to be an important target for the treatment of both genetic and acquired glomerular diseases. Finally, TRPC3 plays a key role in the influx of Ca 2+ into principal cells.
TRPM Channels
The discovery and subsequent characterization of members of the TRPM family of cation channels has highlighted new insights into unknown aspects of ion (cellular) homeostasis regulation. The groups of Konrad and Sheffield reported that hypomagnesemia associated with secondary hypocalcemia (HSH) is an autosomal recessive disease that is genetically linked to TRPM6. This disease is primarily due to defective intestinal Mg 2+ absorption and affected individuals show neurologic symptoms of hypomagnesemic hypocalcemia, including seizures and muscle spasms during infancy. Renal Mg 2+ conservation has been reported to be normal in most patients. In some cases, however, a renal leak has been documented suggesting impaired renal Mg 2+ reabsorption. Patients studied by Konrad et al . and others showed inappropriately high fractional Mg 2+ excretion rates with respect to their low serum Mg 2+ levels. Hypocalcemia is secondary to parathyroid failure resulting from Mg 2+ deficiency. TRPM6 is a member of the long TRPM family and is similar to TRPM7 (also known as TRP-PLIK ), a unique bifunctional protein known as a Mg 2+ and Ca 2+ -permeable cation channel with protein kinase activity. TRPM6 has a~4 fold higher affinity for Mg 2+ compared to Ca 2+ , but is clearly permeable for Ca 2+ ( Table 62.1 ). TRPM6 is exclusively expressed in the early part of the DCT consistent with its role being the gatekeeper of active transepithelial Mg 2+ transport. In addition, other TRPM channels have been detected in kidney. Northern and immunoblot analysis demonstrated abundant expression of TRPM3 in human kidney. Functional studies indicated that TRPM3 is permeable for Ca 2+ and activated by reduction of extracellular osmolarity ( Table 62.1 ). Immunohistochemical localization studies should provide more information about the physiological function of TRPM3 in the kidney.
TRPP Channels
Growing evidence has emerged that polycystins are ion channels or regulators of ion channels. This suggests that autosomal-dominant polycystic kidney disease (ADPKD), which arises from mutations in polycystins, is a form of ion channel disease. ADPKD is a major, inherited nephropathy affecting over 1:1000 of the world wide population. The disease is caused by mutations in two genes, PKD1 and PKD2, which encode for the proteins, polycystin-1 and polycystin-2 (TRPP2), respectively. Although disease-associated mutations have been identified in these two proteins, the sequence of molecular events leading to clinical symptoms is still unknown. Polycystin-1 resides in the plasma membrane and it is proposed to function in cell-cell and cell-matrix interactions, whereas TRPP2 is concentrated in the endoplasmic reticulum ( Table 62.1 ). In contrast to the nonselective cation channel TRPP2, TRPP1 is a large, integral membrane protein that structurally resembles a receptor or adhesion molecule. TRPP1 and TRPP2 form a protein complex in cilia, which are microtubule-based organelles that function as cellular mechanosensors. Flow of fluid causes cilia to bend, which in turn results in a TRPP1/TRPP2 complex-dependent increase in intracellular Ca 2+ levels, directing attention to the regulation of intracellular Ca 2+ as a possible misstep that participates in cyst formation. Immunostaining of human and mouse renal tissues has demonstrated widespread and developmentally regulated TRPP2 expression, with the highest level in thick ascending limb (TAL) and DCT.
TRPV Channels
Plant and colleagues identified TRPV4 as a Ca 2+ -permeable, non-selective cation channel that exhibits spontaneous activity in isotonic media and is rapidly activated by a decrease in extracellular osmolarity, cell swelling, heat or phorbol esters. TRPV4 is expressed at high levels in kidney and here subtle changes in osmolarity result in significant changes in the intracellular Ca 2+ concentration. Here, TRPV4 is candidate for a molecular sensor that confers osmosensitivity. Liedtke and Friedman showed that TRPV4 knockout mice have reduced water intake and become more hyperosmolar compared to wild-type littermates. The response to both hyper- and hypoosmolar stimuli was impaired in these knockout mice. Immunohistochemical studies have shown that the expression of this osmotically-responsive cation channel is restricted to water-impermeant nephron segments. The strongest expression of TRPV4 was observed along the basolateral membrane in the ascending thin limb (ATL) and the thick ascending limb (TAL) as was DCT and CNT. TRPV4 was absent from the proximal tubule (PT) and decending thin limb (DTL). These data imply a role for TRPV4 in the response of renal epithelial cells to anisotonicity, particularly in the hypotonic luminal milieu of TAL and DCT, and the potentially hypotonic luminal milieu of the CD.
The most Ca 2+ -selective channels of the TRP superfamily are the epithelial Ca 2+ channels TRPV5 and TRPV6. These latter channels are predominantly expressed in organs that mediate transepithelial Ca 2+ transport. Unique properties distinguish them from other TRP channels and studies by several groups have demonstrated that TRPV5 and TRPV6 exhibit all the characteristics being the gatekeepers of active Ca 2+ transport in kidney and small intestine, respectively. These epithelial Ca 2+ channels of the TRPV family have been studied in great detail and are, therefore, outlined in the following paragraphs.
Epithelial C A 2+ Channels: TRPV5 and TRPV6
cDNA and Primary Protein Structure
The epithelial Ca 2+ channel family is restricted to two members and genomic cloning demonstrated that these Ca 2+ channels are transcribed from distinct genes juxtaposed on human chromosome 7q35, which suggests an evolutionary gene duplication event. TRPV5 and TRPV6 are completely conserved in terms of exon size in the coding regions and they share similar intron-exon structures with the other TRP genes. To date, epithelial Ca 2+ channels have been cloned from several species including pufferfish, rabbit, rat, mouse and human. TRPV5 and TRPV6 exhibit an overall homology of about 75%. Remarkably, several domains in TRPV5/6 are completely conserved within these species including the core structure of the protein consisting of 6 TM segments and the pore region, ankyrin repeats, PDZ motifs and putative protein kinase phosphorylation sites ( Figure 62.2 ). Four TRP-channel subunits will form the functional Ca 2+ channel. In addition, it was demonstrated that both epithelial channels are high mannose- and complex-glycosylated. PDZ motifs and ankyrin repeat domains are present in a diverse range of receptors and ion channels including other members of the TRPV subfamily. PDZ motifs are recognized by PDZ domains that are modular protein interaction domains playing a role in protein trafficking and protein complex assembly. In general, ankyrins connect ion channels, transporters and cell adhesion molecules to the spectrin-based cytoskeletal elements in distinct membrane domains.

Distribution of TRPV5 and TRPV6
The renal expression profile of TRPV5 has been studied in detail. In different species, it was demonstrated that TRPV5 co-localizes with the Ca 2+ transport proteins, including calbindin-D 28K and the extrusion proteins, the plasma membrane ATPase 1b (PMCA1b) and the Na + -Ca 2+ exchanger type 1 (NCX1) in the late DCT (DCT2) and the CNT ( Figure 62.3 ). Transepithelial Ca 2+ transport is generally envisaged as a three step process consisting of passive entry of Ca 2+ across the apical membrane, cytosolic diffusion of Ca 2+ bound to calbindin-D 28K and active extrusion of Ca 2+ across the opposite basolateral membrane via PMCA1b and NCX1. The highest immunocytochemical abundance of TRPV5 was found in DCT2 with a gradual decrease along CNT. A minority of cells along CNT lacked immunopositive staining for TRPV5 and the other Ca 2 + -transporting proteins. These negative cells were identified as intercalated cells.

In contrast to TRPV5, which is mainly expressed in the kidney, TRPV6 shows a more ubiquitous expression pattern. TRPV6 expression was predominantly found in the brush-border membrane of the small intestine where it co-localizes with calbindin-D 9K and PMCA1b in agreement with the expected properties being the gatekeeper in active Ca 2+ absorption. Recently, TRPV6 was detected in mouse kidney along the apical domain of DCT2 through inner medullary collecting duct (IMCD). TRPV6 co-localizes with TRPV5 and the other Ca 2+ transport proteins in DCT2 suggesting a role in Ca 2+ reabsorption. In addition, the protein is also detected in the intercalated cells and the IMCD that are not involved in transepithelial Ca 2+ transport pointing to additional functions of TRPV6. Thus, the precise role of this epithelial Ca 2+ channel in the kidney remains to be established, but given the widespread distribution of TRPV6 throughout the nephron segments functions could include Ca 2+ reabsorption, Ca 2+ signaling and others.
Biophysical Properties
In Human Embryonic Kidney (HEK) 293 cells heterogeneously expressing TRPV5, currents through the channel can be activated under conditions of high intracellular Ca 2+ buffering by hyperpolarizing voltage steps. In divalent cation free solutions, large inward currents are observed, which show a typical time-dependent increase (gating) that remains constant for more than one minute. Outward currents are negligible indicating that the channel is nearly completely inwardly rectifying. In analogy to voltage-dependent Ca 2+ channels, permeation through TRPV5/6 can by described by “repulsion” pore models considering a pore consisting of two high affinity binding sites, whereby the double occupation of the two binding sites by either Na + or Ca 2+ provides the “drive” for Ca 2+ conduction due to mutual repulsion of the two cations or by a three binding-site model in which two low affinity sites flank a high affinity binding site for Ca 2+ . Importantly, the current through TRPV5 and TRPV6 is carried exclusively by Ca 2+ at physiological extracellular Ca 2+ concentrations. In the situation where Ca 2+ is absent single channel Na + currents through TRPV5 can be measured in inside-out patches. Given the low conductance of these channels in the presence of extracellular Ca 2+ reliable single channel measurements are technically not feasible.
A characteristic aspect of currents through the epithelial Ca 2+ channels is the inactivation at negative membrane potentials. This inactivation is nearly complete if Ca 2+ is the charge carrier and is significantly delayed when Ca 2 + is substituted for Ba 2+ or other divalents indicating that the process is Ca 2+ -dependent. Furthermore, currents of monovalent cations through these Ca 2+ channels do not inactivate. Repetitive stimulation of TRPV5 and TRPV6 currents by short hyperpolarizing pulses consequently result in a decay of the current. The slow recovery from inactivation in divalent cation free solution after the Ca 2+ -dependent decay is a further typical hallmark of these Ca 2+ channels. Although the above described structural aspects and basic electrophysiological properties of TRPV5 and TRPV6 are rather similar, some differences exist between these homologous channels including permeability of divalent cations, kinetics of Ca 2+ -dependent inactivation and recovery from inactivation. For instance, TRPV5 shows a higher permeability for Ba 2+ compared to TRPV6, e.g., the current ratio I Ba /I Ca is ~0.9 for TRPV5, but only ~0.4 for TRPV6. TRPV6 clearly shows a fast component of inactivation which is less prominent for TRPV5, e.g., time to 10% inactivation at hyperpolarizing steps to–100 mV is ~25 ms for TRPV5 but only ~40 ms for TRPV6. Interestingly, these typical differences could be explained by sequence differences between TRPV5 and TRPV6. Interestingly, the structural determinants of these differences appear not to be located in either the amino- or carboxyl-terminus, but in the intracellular TM2-TM3 linker sequence. Substitution of this TM2–TM3 linker of TRPV6 to TRPV5 confers the kinetic and permeation phenotype of TRPV6 to TRPV5, whereas exchanging the amino- or carboxyl-termini is ineffective. Mutation studies by Suzuki and co-workers demonstrated that a histidine residue at position 587 in the carboxyl-terminus close to TM segment 6 of TRPV6 affect the inactivation behavior of TRPV6. In addition some distinct pharmacological differences exist, e.g., ruthenium red is a 100-fold more potent blocker for TRPV5 than TRPV6 (IC 50 ~9 μM for TRPV6 but~100 nM for TRPV5). Furthermore, TRPV5 is approximately four times more sensitive to Cd 2+ blockage than TRPV6 (IC 50 ~70 nM for TRPV5 but~260 nM for TRPV6).
Ion Selectivity
To date, TRPV5 and TRPV6 are the most Ca 2+ -selective channels in the TRP superfamily. The unique permeation property in the epithelial Ca 2+ channels is not conserved in the TRPV subfamily, which shares the highest homology with TRPV5 and TRPV6. TRPV5 and TRPV6 display P Ca /P Na values of more than 100 whereas a permeation sequence of Ca 2+ >Ba 2+ >Sr 2+ >Mn 2+ has been reported. The permeation profile of monovalent cations is Na + >Li + >K + >Cs + .
Nilius and co-workers have demonstrated that the molecular determinants of the Ca 2+ selectivity and permeation of TRPV5 and TRPV6 reside at a single aspartate residue in the pore-forming region (TRPV5-D542 and TRPV6-D541) ( Figure 62.4 ). Neutralization of these negatively charged amino acid residues affects the high Ca 2+ selectivity of the epithelial Ca 2+ channels. These data suggest that high Ca 2+ selectivity in TRPV5 and TRPV6 depends on a ring of four aspartate residues in the pore region, similar to the ring of four negative residues (aspartates and/or glutamates) in the pore of voltage-dependent Ca 2+ channels. Most likely, D542/D541 is the narrowest part of the selectivity filter with a diameter of approximately 5.2 Å and forms part of the high affinity-binding site for Ca 2+ . A detailed analysis of the structure of the TRPV5 and TRPV6 pores has been recently published ( Figure 62.4 ). The amino acid residues facing the pore were determined by cysteine scanning mutagenesis (SCAM). Cysteines introduced in a region preceding D542 for TRPV5, and D541 for TRPV6, displayed a cyclic pattern of reactivity to cysteine reacting agents indicative of a pore helix. This pattern of covalent modification of cysteines supports a KcsA-homology based 3-D model ( Figure 62.4 ). In addition, the pore region of TRPV5 and TRPV6 contains additional negatively charged amino acid residues that only have minor effects on the Ca 2+ permeation properties. In another study, the role of D542 for blockage of monovalent currents through TRPV5 by Ca 2+ and Mg 2+ was supported, however, not for determining Ca 2+ permeability. However, all of our data including recent findings from concatemers and a detailed study of TRPV6 pore properties clearly demonstrated that the Ca 2+ selectivity is determined by D542/D541.

Mechanism of High Ca 2+ Selectivity
The high Ca 2+ selectivity of TRPV5 and TRPV6 refers to a mechanism of permeation as proposed previously for voltage-dependent Ca 2+ channels. In the absence of Ca 2+ , Mg 2+ will bind at a site within the pore-region, which is mainly determined by D542/D541. At low driving forces, e.g. less negative membrane potentials, the driving force is not sufficient to displace Mg 2+ out of the pore and the channel is blocked. At hyperpolarisation, Mg 2+ will be moved from this high affinity site thereby allowing monovalent cations to permeate the channels. Because of the higher affinity of the binding-site for Ca 2+ versus Mg 2+ , Ca 2+ will outnumber Mg 2+ at this site and is now blocking the pore for a movement of monovalent cations. If the inward driving force is sufficient enough to remove Ca 2+ from the high affinity site, a fast inward permeation occurs supported by the low affinity sites, which flank the high affinity Ca 2+ -binding site. All the data so far support the crucial role of D542/D541 in the channel pore region of TRPV5 and TRPV6 channels.
Rectification and Gating
A predominant inward rectification is a clear hallmark of the TRPV5 and TRPV6 channels besides the high Ca 2+ selectivity. This inward rectification is only partially due to blockage by Mg 2+ , which is removed by hyperpolarization. Thus, the most prominent part of rectification remains in the absence of intracellular Mg 2+ and is not due to blockage by endogenous polyamines. The intrinsic rectification of these channels is, however, dramatically reduced by neutralization of the negatively charged D542/D541 residues indicating that the permeation site is also involved in rectification. In mammalian cells heterogeneously expressing the epithelial Ca 2+ channels, TRPV5 and TRPV6 are constitutively open at a low intracellular Ca 2+ concentration and negative membrane voltage. The above described mechanism of removing channel blockage by Ca 2+ or Mg 2+ is necessarily part of the gating mechanism. At this moment other mechanisms that might influence gating of TRPV5 and TRPV6 cannot be excluded. Huang and colleagues showed that PIP 2 activates TRPV5 and that activation of TRPV5 by PIP 2 decreases the sensitivity of TRPV5 to inhibition by intracellular Mg 2+ .
Modulation of the Channel Activity
Intracellular Ca 2+
TRPV5 and TRPV6 are controlled by a Ca 2+ -dependent feedback inhibition. Both Ca 2+ channels rapidly inactivate during hyperpolarizing voltage steps and this inhibition is reduced when Ca 2+ is substituted for Ba 2+ or Sr 2+ as charge carriers. The inactivation is dependent on the extracellular Ca 2+ concentration and occurred also in cells buffered intracellularly with BAPTA. In addition, the current disappeared during repetitive activation by short hyperpolarizing pulses. This current decay was significantly diminished when Ca 2+ was replaced by Ba 2+ as charge carrier and abolished when extracellular Ca 2+ was lowered to 1 nM, again indicating that a Ca 2+ -dependent process inhibits channel activity. Increase of the extracellular Ca 2+ concentration significantly stimulated the rate of current decay. Ca 2+ influx is a prerequisite for this phenomenon because the Ca 2+ -impermeable D542A mutant lacks a monovalent current decay in response to repetitive stimulation. These findings suggest that the TRPV5/6 channels are down-regulated by Ca 2+ influx through the channel and thus likely by increasing the Ca 2+ concentration in a micro-domain near the pore region, thereby inducing feedback inhibition of the channel. This could be a crucial mechanism for the regulation of TRPV5 and TRPV6 under physiological conditions. The inhibition is highly Ca 2+ -sensitive with calculated affinity values around 100 nM. Considering the high affinity mechanism of Ca 2+ -dependent TRPV5/6 inhibition, the presence of intracellular Ca 2+ buffering proteins, such as calbindins, will ultimately determine the channel activity.
Pharmacology
Ruthenium red and econazole are the most effective blockers of the epithelial Ca 2+ channels identified so far. The inorganic polycationic dye ruthenium red, which binds to phospholipids, inhibits TRPV5 and TRPV6 in a voltage-dependent manner. Transepithelial Ca 2+ transport in primary cultures of immunodissected rabbit CNT and CCD are inhibited in the same concentration range. Antimycotic imidazoles such as econazole and miconazole are highly effective inhibitors with IC 50 values of the micromolar range. These compounds block the channels, however, voltage-independently. In addition, TRPV5 and TRPV6 are efficiently blocked by inorganic cations according to the following profile: Pb 2+ =Cu 2+ =Gd 3+ >Cd 2+ >Zn 2+ >La 3+ >Co 2+ >Fe 2+ >> Fe 3+ with IC 50 values between 1 and 10 μM. Blockers of store-operated Ca 2+ entry channels (SOC), e.g., SKF965 and 2-aminoethoxydipheny borate (2-APB), are nearly ineffective for TRPV5 and TRPV6. Another SOC blocker, the adenylcyclase inhibitor MDL 12330A, exerts half-maximal inhibition of TRPV5 at ~20 μM. Xestospongin, a noncompetitive inositol (1,4,5)-trisphosphate receptor antagonist seems, however, to block TRPV6. Blockers of protein tyrosine kinases such as tyrphostin B46 and genistein, CaM antagonists calmidazolium R24571 and trifluoperazine, and voltage-dependent Ca 2+ channel blockers such as mibefradil, quinidine, nifedipine, niflumic acid and verapamil, have only small or no effects on TRPV5 activity. Interestingly, blockage by ruthenium red and Cd 2+ is much more sensitive for TRPV5 than TRPV6 which can, therefore, be used to differentiate both Ca 2+ channels.
pH
Previous studies indicated that acidification of the apical medium inhibits transepithelial Ca 2+ transport across primary cultures of rabbit CNT and CCD cells. 45 Ca 2+ -uptake in TRPV5-expressing Xenopus laevis oocytes was inhibited by acidification of the incubation medium. Extracellular acidification reduced currents through TRPV5 carried by either monovalent or divalent cations, which was confirmed by Peng and colleagues Additionally, extracellular pH also affected current kinetics, extracellular Mg 2+ blockage and Ca 2+ affinity. The gating component of monovalent cation currents through TRPV5 was delayed at alkaline pH and as well as blockage by extracellular Mg 2+ . For voltage-dependent Ca 2+ channels and cyclic nucleotide-gated channels, it was found that protonation of a single glutamate residue in the pore region is responsible for the dramatic changes in divalent cation affinity by extracellular pH. A similar mechanism might be applicable for TRPV5 and TRPV6 in which a single aspartate residue in the pore determines the Ca 2+ permeation and Mg 2+ blockage of the channel. Extrapolating these findings to the in vivo situation, the pH influence could at least in part provide the molecular basis of acidosis-induced calciuresis. Interestingly, the molecular mechanism of TRPV5 blockage by protons is mechanistically understood taking into account the pore structure described above. Mutation of the glutamate residue at position 522 to glutamine preceding the pore helix decreased the inhibition of the channel by extracellular protons. Thus, pH sensitivity is mainly mediated by glutamate at position 522 and may act as the “pH sensor” of TRPV5.
Molecular Structure
The molecular architecture of TRPV5 and TRPV6 channels has recently been unraveled. Co-immunoprecipitations and molecular mass determination of TRPV5 and TRPV6 complexes using sucrose gradient sedimentation showed that these channels form homo- and heterotetrameric channel complexes ( Figure 62.2 ). Niemeyer and co-workers identified the third ankyrin repeat being a stringent requirement for physical assembly of TRPV6 subunits. It was proposed that the third ankyrin repeat initiates a molecular zippering process that proceeds past the fifth ankyrin repeat and creates an intracellular anchor that is necessary for functional subunit assembly. Subsequent studies by Chang et al . confirmed the importance of the ankyrin repeats in TRPV5 assembly. Functional studies have shown that ankyrin repeat 1 fulfills a predominant role in the oligomerization of TRPV5.
Interestingly, several studies indicated that certain tissues co-express TRPV5 and TRPV6 which allows oligomerization of these channels in vivo . Immunohistochemical studies demonstrated co-expression of TRPV5 and TRPV6 in DCT. Heteromeric complex formation can modify the activity of members of the TRP family. Likewise, hetero-oligomerization of TRPV5 and TRPV6 might influence the functional properties of the Ca 2+ channel. Because TRPV5 and TRPV6 exhibit different channel kinetics with respect to Ca 2+ -dependent inactivation, Ba 2+ selectivity and sensitivity for inhibition by ruthenium red, the influence of the heterotetramer composition on channel properties was investigated. Concatemeric channels were constructed consisting of four TRPV5 and/or TRPV6 subunits configured in a head-to-tail fashion. Differences in the ratio of TRPV5 and TRPV6 subunits in these concatemers showed that the phenotype resembles mixed properties of TRPV5 and TRPV6. Thus, regulation of the relative expression levels of TRPV5 and TRPV6 may be a mechanism to fine-tune the Ca 2+ transport kinetics in kidney or other TRPV5/6-co-expressing tissues.
The tetrameric organization of TRPV5 and TRPV6 resembles that of the voltage-dependent Ca 2+ channel and the Shaker potassium channel that are composed of four tandemly associated homologous domains. Clustering of four subunits is assumed to construct an aqueous pore-region centered at the four-fold symmetry axis. This tetrameric architecture of TRPV5/6 implies that four of the aspartic residues (D542, D541) form a negatively charged ring structure that functions as a selectivity filter for Ca 2+ in analogy with voltage-dependent Ca 2+ channels. Protein crystallography of these channels will be the most challenging approach to determine the three-dimensional structure in the near future.
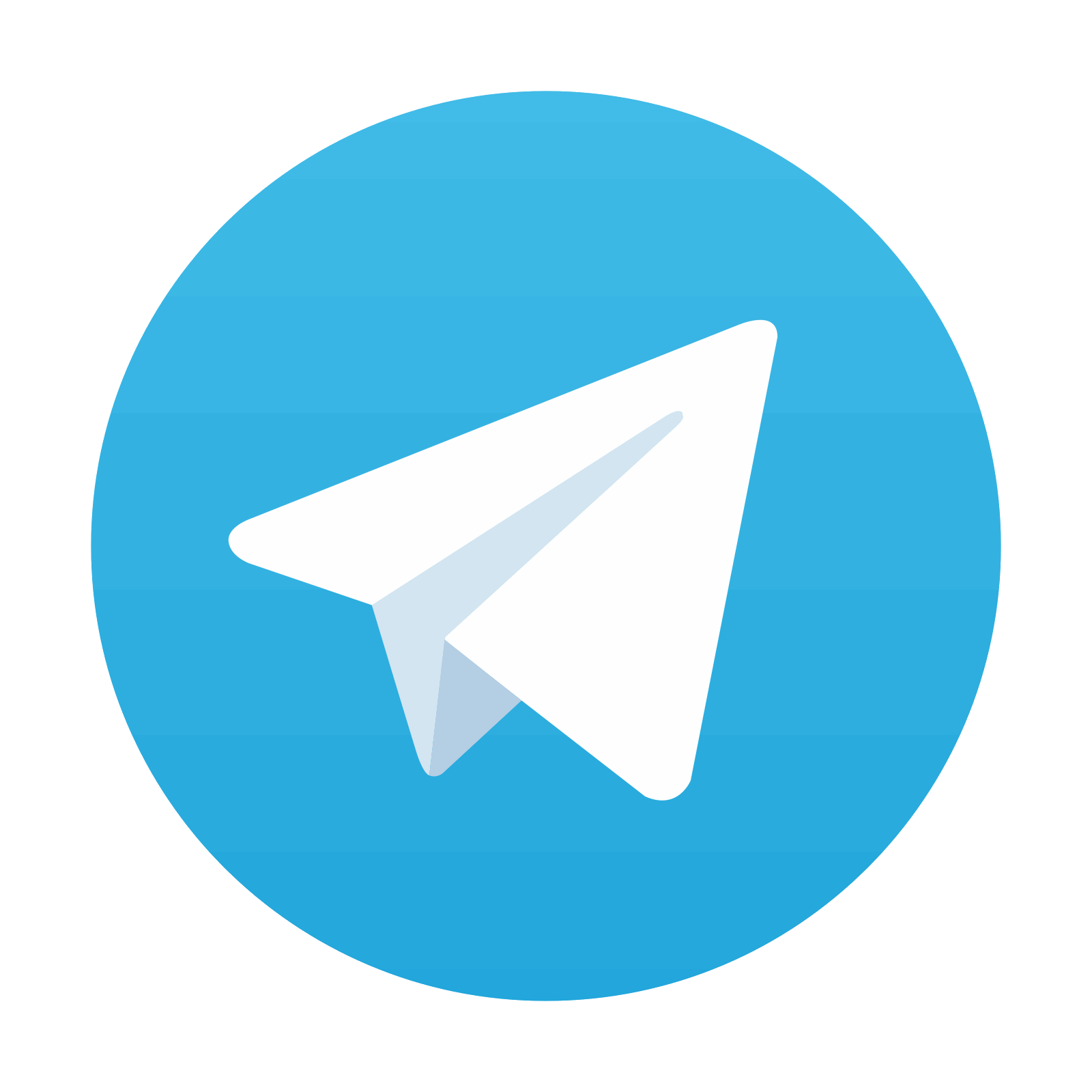
Stay updated, free articles. Join our Telegram channel

Full access? Get Clinical Tree
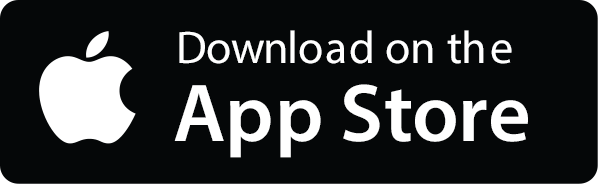
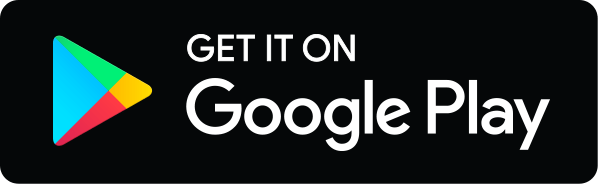