Voiding Dysfunction
Jennifer Miles-Thomas
James E. Wright
Normal voiding function relies on complex interactions between the autonomic and somatic nervous systems. Precise coordination allows the lower urinary tract to accomplish the principal tasks of storage and emptying under both volitional and unconscious control. The continuum from normal to abnormal voiding function follows a paradigm that is generally simple to reconcile if reduced to disorders of function (storage and emptying) and disorders of anatomy (the bladder, the bladder outlet/urethra). This chapter will discuss normal voiding and voiding dysfunction, paying attention to relevant aspects of neurophysiology. It will also provide a practical approach to clinical diagnosis and therapy considerations for lower urinary tract disorders.
NORMAL URINARY ANATOMY (1)
Bladder
The urinary bladder is a multifunctional organ. Principal functions include stretch in response to filling without increasing intravesical pressure, urine storage at low pressure, protection of the underlying smooth muscle and nerves from urine, and coordinated expulsion of urine.
The smooth muscle of the urinary bladder allows tension to be developed over a large range of muscle lengths (2). The contractile response is slower and longer than that of cardiac or skeletal muscle, and detrusor muscle uses less energy to maintain tension over longer periods of time. Poor electrical coupling of the bladder smooth muscle is thought to help prevent synchronous activity of the smooth muscle as a whole during the filling phase (3). Bladder contraction during voiding is mediated by parasympathetic stimulation (4).
Collagen types I, III, and IV are most commonly found in the urinary bladder. Collagen, elastin, and proteoglycans located in the stroma account for the mechanical properties of the vesicoelastic bladder wall (5). The urothelium of the bladder is made up of multiple layers. Umbrella cells form the epithelial lining and are covered by a glycosaminoglycans layer. The glycosaminoglycans may inhibit bacterial adherence and prevent large molecule damage to the underlying urothelial layers (6). An intermediate layer and a basal cell layer complete the urothelium (7). Urothelial cells express nicotinic, muscarinic, tachykinin, adrenergic, and capsaicin receptors. They also can release ATP and nitric oxide and have mechanosensitivity and sensitivity to transmitters released from local afferent and efferent nerves. These properties allow the urothelium to respond to the changing environment and communicate with other cells and nerves in the bladder (8, 9, 10, 11).
Urethra and Internal Sphincter
The urethra is composed of both striated and smooth muscle. Striated muscle bundles in the walls of the urethra form the rhabdosphincter separate from the pelvic floor musculature. Smooth muscle bundles of the urethra form the thick inner longitudinal and outer circular layer of the urethra, helping to stabilize and occlude the lumen. In females, this extends to the proximal portion. The
stroma of the urethra is composed of collagen and elastin. The mucosal lining also provides coaptation to assist in urinary storage (12). The bladder neck (internal urinary sphincter) is a smooth muscle sphincter with α-adrenergic and a few β-adrenergic receptor sites. The bladder neck is thought to be partially controlled by the sympathetic stimulation of the α-receptors.
stroma of the urethra is composed of collagen and elastin. The mucosal lining also provides coaptation to assist in urinary storage (12). The bladder neck (internal urinary sphincter) is a smooth muscle sphincter with α-adrenergic and a few β-adrenergic receptor sites. The bladder neck is thought to be partially controlled by the sympathetic stimulation of the α-receptors.
External Sphincter
In females, the urethra is reinforced by the pelvic floor musculature and connective tissue. Continence is maintained by active contraction and the anatomic compression of the urethra against the posterior pelvic floor. The external sphincter is composed of both slow and fast twitch fibers. In the female urethra, 87% are slow twitch fibers while 13% are fast twitch (6).
NEUROPHYSIOLOGY
Normal voiding is a complex, tightly orchestrated neuromuscular cascade of events coordinating low-pressure storage of urine in the bladder and efficient emptying of stored urine. Understanding the relationship between the central and peripheral nervous systems and the bladder, urethra, and pelvic floor is important for describing both normal and abnormal function of the lower urinary tract.
Central Nervous System Effects
Micturition is the result of supraspinal neurologic pathways that either inhibit or facilitate segmental reflex arcs (13, 14, 15). Each anatomic component of the central nervous system plays a role in voiding function. During sustained voluntary pelvic floor straining, the anterior frontal gyrus has been shown on positron emission tomography (PET) studies to be activated (16), supporting the postulate that the frontal lobe is involved in both voluntary micturition and inhibition of the micturition reflex. The pontine mesencephalic reticular formation, also known as Barrington’s center, is located in the anterior pons. Two separate areas in the pons play a role in coordinating urine storage and emptying. The M region in the pontine micturition center is also known as Barrington’s nucleus. Located in the dorsal pontine tegmentum, it projects to the bladder motor neurons in the sacral parasympathetic nucleus and sphincteric interneurons. Stimulation causes bladder contraction and external sphincter relaxation. Damage to this area leads to urinary retention. The L region is known as the pontine storage center. It projects to Onuf’s nucleus, where motor neurons control the external sphincter. Stimulation of this area causes contraction of the urinary rhabdosphincter and increases urethral resistance (17).
The cerebellum coordinates the force of detrusor contraction and pelvic floor activity. In addition, cerebellar impulses interact with the brain stem reflex centers, including Barrington’s nucleus, to coordinate voiding (14,18). The cerebellum plays an inhibitory role during bladder filling and with Barrington’s nucleus is involved in rapidly increasing and maintaining bladder pressure during voiding (19,20).
In concert with these central components, sensory afferents travel from the bladder to the brain stem through the spinothalamic tract, providing feedback on bladder filling and voiding cues. In this way, information from the frontal lobes to the pons coordinated in the basal ganglia and cerebellum directs the volitional control of micturition, while pathways from the brain stem to the sacral micturition center coordinate detrusor and sphincter reflexes to allow for bladder evacuation (19,21). While this complex interplay within the central nervous system provides an efficient storage and emptying cycle, it poses multiple sites for injury and subsequent dysfunction.
Autonomic Nervous System Effects
The lower urinary tract has parasympathetic, sympathetic, and somatic innervation. The pelvic nerve supplies the bladder and urethra with efferent parasympathetic input while the hypogastric nerves supply sympathetic components. Both the pelvic and hypogastric nerves return sensory afferent information to the spinal cord. The primary influence of the sympathetic innervation is control of the storage phase of the micturition, while the parasympathetic innervation controls the voiding phase (22, 23, 24).
Parasympathetics
Parasympathetic efferents originate in the gray matter of S2-S4 in the lateral aspect of the sacral intermediate matter and exist with preganglionic fibers as the pelvic nerve (6). The pelvic nerve joins the ipsilateral hypogastric nerve to form the pelvic plexus innervating the bladder and urethra. Afferent autonomic nerves travel to the dorsal column of the spinal cord through the pelvic nerve (22, 23, 24). The preganglionic neurotransmitter is acetylcholine, which affects nicotinic cholinergic receptors. The primary postganglionic neurotransmitter
is also acetylcholine, activating muscarinic receptors. These M2 and M3 receptors are distributed throughout the body, with increased expression in the bladder. They are rare in the bladder neck and urethra. M3 receptors primarily mediate bladder contraction.
is also acetylcholine, activating muscarinic receptors. These M2 and M3 receptors are distributed throughout the body, with increased expression in the bladder. They are rare in the bladder neck and urethra. M3 receptors primarily mediate bladder contraction.
Sympathetics
The sympathetic nervous system is important in bladder filling and storage. Sympathetic nerves (T11-L2) travel in the intermediolateral nuclei of the thoracolumbar spinal cord and in the hypogastric nerve (25, 26, 27, 28, 29). Stimulation of B2 receptors in the bladder body promotes relaxation, while stimulation of α-1 receptors in the bladder neck and urethra increases bladder outlet resistance and has inhibitory effects on parasympathetic transmission. Alpha-1 receptors are postsynaptic receptors that stimulate vasoconstriction and smooth muscle contraction. Alpha-2 receptors are presynaptic receptors that inhibit the release of norepinephrine through negative feedback. Beta-adrenergic receptors found in the body of the bladder modulate smooth muscle relaxation. Despite this interaction, β-agonist agents have not been found useful for modulating detrusor overactivity (30).
Afferent Pathways to the Bladder
Afferents from the pelvic nerve consist of (Adelta) myelinated axons and (C-fiber) unmyelinated axons. These fibers monitor the amplitude of bladder contraction and bladder volume. C-fibers are located in the mucosa and mucosa muscularis and are nocioreceptive, responding to stretch and overdistention. Recruitment of C-fibers during inflammation or during neuropathic changes may cause bladder pain or urge incontinence (6). Adelta fibers located in the smooth muscle sense bladder fullness and wall tension.
Neurotransmitters
There are multiple nonadrenergic, noncholinergic neurotransmitters (NANC) present in the central and peripheral nervous systems that affect bladder function. These transmitters, along with various receptor families, offer unique targets for understanding and manipulating lower urinary tract function. Opioids, serotonin, GABA, and dopamine represent a few of these transmitters. Serotonin inhibits the voiding reflex and has some role in increasing urethral tone. ATP acts on purine receptors P2X and P2Y to influence mechanosensory signaling (6,17).
Adenosine (the breakdown product of ATP) has receptors that can modulate afferent and efferent responses. Capsaicin is a vanilloid that stimulates and desensitizes unmyelinated C-fibers to produce pain and additional neuropeptides. Tachykinins (i.e., substance P, neurokinin A, neurokinin B) are released in response to capsaicin and mediate increased excitability of the bladder and bladder contractions and induce vasodilatation. Nitric oxide is a major inhibitory transmitter causing relaxation of urethral smooth muscle during voiding. In addition, it is also released from urothelium during bladder filling and may suppress afferent nerve activity (6,17). Research is ongoing to determine which of these transmitters and receptors can be used to influence lower urinary tract function and dysfunction.
VOIDING DYSFUNCTION
Many classifications exist for defining and stratifying voiding dysfunction. Some address specific neurologic insults while others focus on urodynamic features. Each of these has strengths and limitations. A clinically useful paradigm focuses primarily on defining abnormalities with reference to urine storage and emptying (31). From a functional and anatomic standpoint, the bladder and bladder outlet (bladder neck and urethra) are the key components to normal voiding, and clinical diagnosis and treatment planning can be aided by identifying dysfunction in these elements. A two-by-two matrix can be useful for orienting clinical, urodynamic, and neurologic information, as shown in Tables 18.1 and 18.2.
The following discussion touches on specific causes of voiding dysfunction, many of which are specifically addressed elsewhere in this textbook. The list is not meant to be exhaustive, but serves instead to guide further thought and inquiry into the diagnosis and treatment of voiding dysfunction.
DISORDERS OF URINE STORAGE
Stress Incontinence
Stress urinary incontinence is defined by the International Continence Society as the involuntary leakage of urine with effort or exertion or on sneezing or coughing (32). During urodynamic evaluation, leakage is seen with abdominal contraction in the absence of detrusor contraction. Stress incontinence accounts for approximately 50% of cases of incontinence and is due to urethral dysfunction characterized by urethrovesical hypermobility or intrinsic sphincter deficiency (ISD).
Urethral hypermobility is due to loss of periurethral support to the pelvic floor musculature. Loss of the native tissue structure allows for mobility, with increases in abdominal pressure preventing adequate anatomic coaptation (33, 34, 35). With increasing abdominal pressure, the bladder neck and proximal urethra can be pulled open, allowing for incontinence. ISD is present in the setting of stress incontinence where urethral support is adequate. Poor coaptation of an intrinsic mucosal and muscular seal is thought to allow leakage of urine during increased intra-abdominal pressure.
Urethral hypermobility is due to loss of periurethral support to the pelvic floor musculature. Loss of the native tissue structure allows for mobility, with increases in abdominal pressure preventing adequate anatomic coaptation (33, 34, 35). With increasing abdominal pressure, the bladder neck and proximal urethra can be pulled open, allowing for incontinence. ISD is present in the setting of stress incontinence where urethral support is adequate. Poor coaptation of an intrinsic mucosal and muscular seal is thought to allow leakage of urine during increased intra-abdominal pressure.
TABLE 18.1 Disorders of Storage | ||||||||||||||||||||||||||||||||||||||||||
---|---|---|---|---|---|---|---|---|---|---|---|---|---|---|---|---|---|---|---|---|---|---|---|---|---|---|---|---|---|---|---|---|---|---|---|---|---|---|---|---|---|---|
|
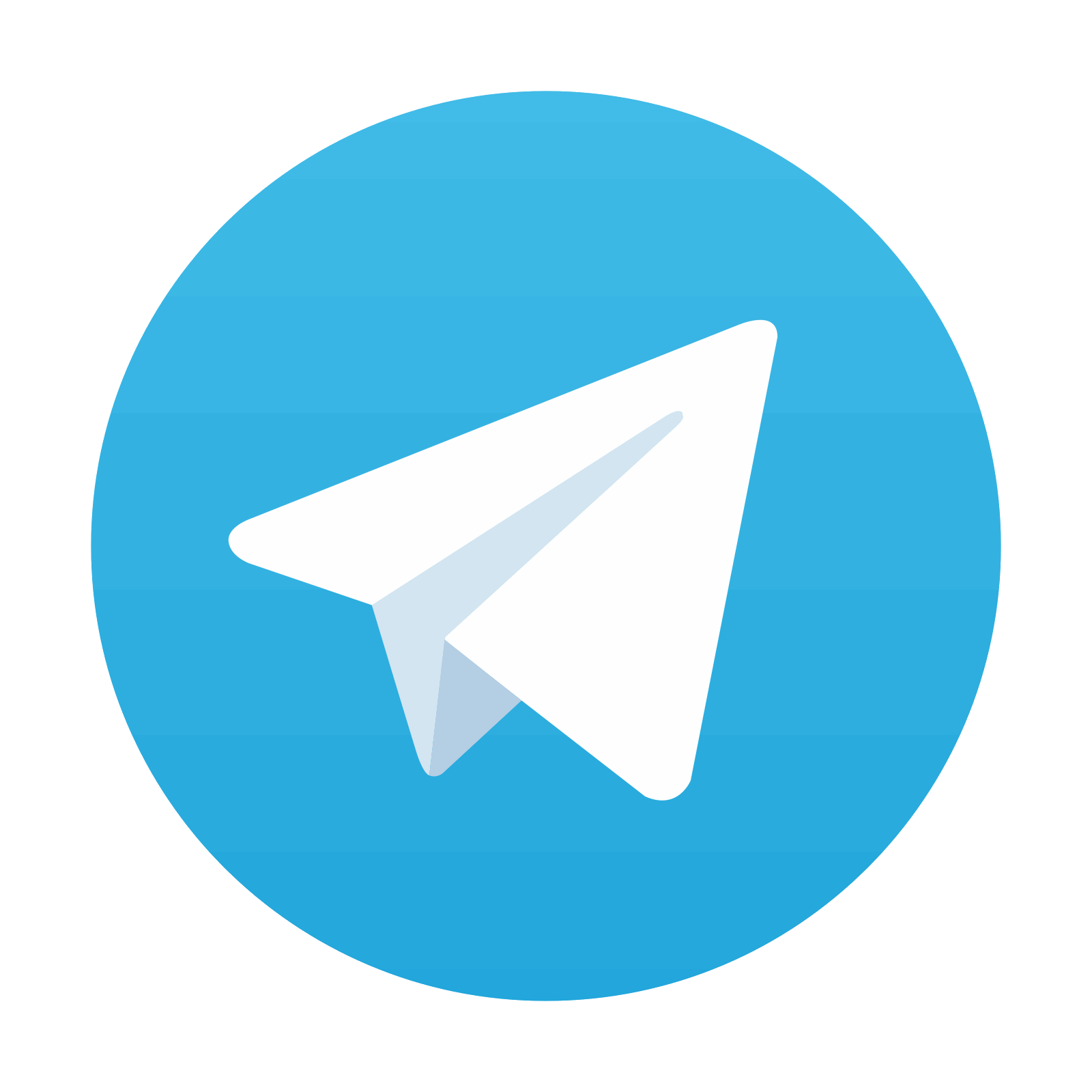
Stay updated, free articles. Join our Telegram channel

Full access? Get Clinical Tree
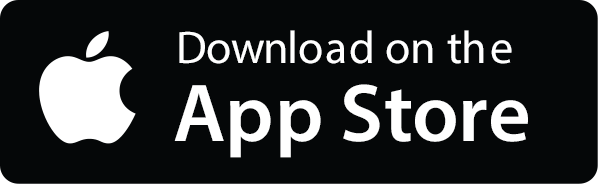
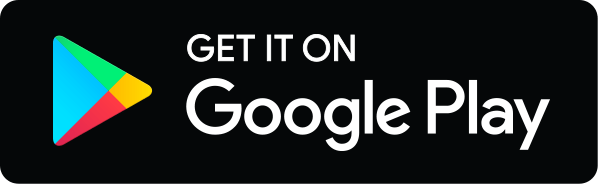