8
The Effects of Aging, Hypogonadism, and Nutrition on Endurance and Strength
“… come to the grave in full vigor, like sheaves gathered in season.” Job 5:26
“Living systems are worn out by inactivity and developed by use.” Albert Szent-Gyorgyi
“A hospital bed is a parked taxi with the meter running.” Groucho Marx
The interaction between physical activity and aging is complex, but antithetical. In many instances the perception of aging is due to inactivity and self-perpetuating. The decline in testosterone and other trophic hormones with age contributes in a myriad of ways to the perceived acceleration of physical decline in men, not only by minimizing the benefits of activity, but also by contributing to an apathetic senescence, making the pursuit of physical activity more difficult. Nutritional issues complicating the elderly man further augment this maelstrom, and can push the older man into andropause and physical decline by minimizing testosterone production and enhancing estrogen formation (zinc deficiency) or increasing atherogenic substances, such as homocysteine (folate or B12 deficiencies). Which factors dominate this downward spiral are difficult to dissect, but the outcome is enhanced frailty, diminished quality of life, and accelerated senescence. What society wants is enhanced vitality with aging and natural augmentation of life expectancy, with eradication of diseases of lifestyle, even though we are not yet at the point of augmenting life span, the maximal obtainable age of a particular member of the human species. It remains to be seen if the optimization of hormonal status, enhanced physical activity, coupled with a scientific nutrition base, will make this happen.14
Working Case Report in Andropause, Activity, and Nutrition
E.B. was a 75-year-old retired university president who had had a successful prostatectomy 10 years ago for well-confined prostate cancer. His postsurgical prostate-specific antigens (PSAs) remained low and he had retained minimal sexual function. He had gained weight and was on a statin for lipid control. He did not exercise. He was seen by me for mild anemia (hemoglobin 13.2 g%) and lassitude, thought to be related, which previously had been evaluated at two university medical centers, but iron studies, B6, B12, folate, and bone marrow studies were normal.
The patient did not engage in regular aerobic activity. Why is this point important? Regular aerobic activity is essential in treating modest anemia. Athletes experience plasma volume expansion, with no decrement in red blood cell mass, secondary to a heightened renin-angiotensin-aldosterone activation. This is a principal cause of “runner’s anemia,” which is a normal adaptation to regular aerobic exercise. The patient did complain of loss of energy and some decline in sexual function over the past 3 years (one sexual episode/month versus two to three before that time). He attributed this to age. He was quite hirsute and had significant male pattern baldness. His body fat was 29% and mesenteric. His cholesterol/high-density lipoprotein (HDL) ratio was 4.2.
I initiated a hormonal evaluation. His total testosterone was 564 ng/dL and his free testosterone was 103.0 pg/mL (normal range is 50–210). The concept of relative hypogonadism is important. A person’s free testosterone may have been very high years before he is seen for treatment. A man whose free testosterone falls from 200 to 100pg/mL will experience more symptoms than will a man whose free testosterone falls from 70 to 50! The patient’s follicle-stimulating hormone (FSH) was 62.9 (normal 1–8). His luteinizing hormone (LH) was 19.7 (normal 2–12). I found this result surprising. His baseline free testosterone may have been higher than 210 pg/mL! He is, by definition, in andropause. Both LH and FSH are extremely high, which tells you his hypothalamus detects a deficiency in androgens. He was placed on 5 g 1% of Androgel and started on a high-protein, low-carbohydrate (HPLC) diet. A regular aerobic and weight program was started.
With regard to his prostate cancer, no evidence exists that testosterone causes prostate cancer or that replacement makes it more likely. While controversial, one can argue that high testosterone levels prevent prostate cancer. The patient’s initial PSA was 0.7. One year later it was 0.8. Mild increases in PSA do occur and reflect modest increases in prostate mass. Because this was a minimal increase, I was not concerned. His hemoglobin at this time was now 15.2, and his free testosterone was increased to 145 pg/mL. His LH was 4.6, but his FSH was 24.2. His body fat fell to 15.5%. His cholesterol/HDL ratio was now 3.0. I wondered if I should increase his topical testosterone. I decided not to do so, because he is doing quite well on an integrated hormonal replacement program, coupled with a diet and exercise. His body fat fell because he lost fat mass and increased muscle mass! His sexual activity had increased to twice monthly at 77 and his energy and vigor had substantially improved.
The Characteristics of Aging and the Axioms of Gerontology
The characteristics of aging seem harsh. They are universal, decremental, progressive, and intrinsic (Appendix 1). But the axioms of gerontology moderate this unpleasantness: (1) sustained exercise inhibits the decline of the physique with age; (2) sustained physical activity inhibits the decline of physical fitness with age; and (3) sustained physical activity inhibits the decline of mental functions with age. The net effect is moderation and slowing of the characteristics of aging, with routine exercise and physical activity, and with vitality and independence sustained!5–9
Hormonal Issues Surrounding Aging and Activity
Most experts agree that the major hormones responsible for physical decline in men are falling levels of testosterone, human growth hormone (HGH), and a principal secondary hormone derived from the HGH-liver interaction, insulin-like growth factor-I (IGF-I) (also called somatomedin-C), and dehydroepiandrosterone (DHEA), which are coupled with rising levels of hydrocortisone (stress-induced) and insulin, secondary to increasing insulin resistance, and increasing levels of the testosterone-derived female hormones estradiol and estrone.1,3 Many other hormones are involved to a lesser extent, so we will focus on the major players, while looking at strategies to optimize hormone levels, but not losing sight of nutrition, which directly affects hormone levels in men.10
Testosterone is responsible for the relatively larger muscle mass in males, their increased leanness, and aggression, as compared with females.11–13 Conversely, lower levels of testosterone result in lower levels of muscle mass, increased body fat, and decreased strength, while increasing depressive symptoms and decreasing aggression to the point of apathy1,2,13,14 Supraphysiological amounts of testosterone administered to nonexercising males, by itself results in increased muscle mass, strength, and leanness compared with exercise alone. Combining both yields greater gains.15 The aging man, however, because of rising levels of endogenous adrenal stress hormones (principally hydrocortisone), increasing obesity,16,17 increasing insulin levels, increasing cyclic aromatase activity secondary to increasing mesenteric adiposity, and lower levels of certain nutrients, such as zinc, is victim to both a falling testosterone synthesis and rising levels of sex hormone–binding globulin (SHBG), the latter further decreasing the level of “free testosterone,” in the milieu of falling total testosterone. The rise in SHBG is quite critical, because testosterone in highly bound (almost 99%) and very little is free and bioactive (Fig. 8–1). Conversely, estradiol and estrone are much more weakly bound, and therefore more “adroit” at shutting down the hypothalamic-pituitary-gonadal axis, resulting in a falling LH level and a further decrement in gonadal synthesis of testosterone. The high levels of estrogens further enhance hepatic synthesis of SHBG, augmenting the downward spiral of free testosterone.
Augmenting Testosterone Levels in the Older Hypogonadal Man
What strategies might be employed to circumvent these changes? Increasing the testosterone level, if low, is paramount. The goal is to bring levels in most patients to average or higher than normal levels of free testosterone. This can be accomplished by weight reduction, best promoted by the combination of increased levels of physical activity, including both strength and aerobic training, with an HPLC diet, which has the added benefit of lowering insulin levels and improving lipid levels, compared with traditional lower fat, low-calorie diets.18–20 Energy efficiency on the HPLC diet is markedly decreased and it has been shown to reduce adipocyte fatty acid synthase (FAS) and FAS messenger RNA (mRNA) levels by over 50%, making energy storage in the fat cell much more difficult.18 Decreasing fat mass directly decreases estradiol levels formed by cyclic aromatization of testosterone by the adipocyte, which in turn increases both LH and FSH levels, enhancing endogenous testosterone synthesis. Furthermore, decreased estrogen levels decrease SHBG levels, which raise free bioactive testosterone.
FIGURE 8-1. Testosterone metabolism (direct pathways). Low levels of zinc (Zn) impede synthesis of testosterone and estradiol from their precursors and increasing fat mass increases conversion (via aromatization) of androstenedione to estrone and testosterone to estradiol, lowering testosterone levels. Low levels of zinc and increased fat mass are commonly found in older men. NADPH, reduced nicotinamide adenine dinucleotide phosphate.
Administering testosterone to the hypogonadal man may be directly beneficial, but it potentiates a leaner body mass and enhances gains in strength through training. Furthermore, a testosterone replacement causes a rise in hematocrit11,12 that enhances aerobic performance directly, by increasing oxygen delivery to peripheral muscles and vital organs, and reducing stress on the heart. Myocardial oxygen consumption is directly proportional to mean arterial pressure (MAP) times the heart rate (HR). Exercise lowers both the HR and the blood pressure for any given level of exertion. But increasing myocardial oxygen delivery by increasing hemoglobin levels augments this training effect.21 One of the most surprising findings in recent studies is the antiatherogenic properties of normal testosterone levels in males, refuting earlier medical views that testosterone was responsible for differential rates of heart disease between males and females.22–24 Testosterone’s effect may even extend to coronary artery vasodilatation, because its effects are immediately seen during stress testing in men with established coronary artery disease.24 This body of research so contradicts the previous paradigm that a prospective, controlled, double-blind study on the replacement of testosterone in hypogonadal men will be needed to convince the unconvinced.
Exogenous Testosterone Administration in Men
The preferred routes of administration are transdermal (gels or patches), followed by intramuscular (injection). Transdermal administration results in more even levels of testosterone and avoids the peaks and valleys seen with injection and is associated with lower rates of benign prostatic hypertrophy and polycythemia.1,3,11 There is no evidence that testosterone administration to hypogondal males potentiates the development of prostate cancer.1,3,11
Nutritional Issues Vital to Normal Hormonal Status
Zinc
It is prudent to check zinc levels in all male patients, because low zinc levels cause hypogonadism. Low zinc levels impair testosterone synthesis by the Leydig cells of the testes and increase aromatase activity. Indeed, low levels of zinc in utero will impair the testes irreparably, diminishing both testosterone synthesis and spermatogenesis.25 A mild deficiency of zinc, affecting growth and development in children and adolescents, has been reported from developed countries as well. Zinc deficiency in humans, aside from hypogonadism in males, may manifest as severe, moderate, or mild. The manifestations of severe zinc deficiency include bullous pustular dermatitis, alopecia, diarrhea, emotional disorders, weight loss, intercurrent infections due to cell-mediated immune dysfunctions, neurosensory disorders, and problems with healing of ulcers. A moderate level of zinc deficiency has been reported in a variety of conditions. Clinical manifestations include growth retardation and male hypogonadism in adolescence, arrested penile development in puberty, rough skin, poor appetite, and mental lethargy. Even a mild level of zinc deficiency may present with decreased serum testosterone levels and/or oligospermia in males, and decreased lean body mass. The recognition of mild levels of zinc deficiency has been difficult.4,25–27
Dietary zinc restriction in normal young men was associated with a significant fall (>75%) in serum testosterone concentrations after 20 weeks of zinc restriction. Conversely, supplementation of marginally zinc-deficient normal elderly men for 6 months resulted in a doubling of serum testosterone.28 Zinc is equally important in the postsynthesis disposition of testosterone. Zinc deficiency significantly decreases 5α-reductase conversion of testosterone to dihydrotestosterone (DHT). DHT is responsible for hair loss and prostatic hypertrophy. Zinc deficiency has been shown in animals to further enhance aromatase activity, increasing estradiol production, but also decreasing androgen receptors, while increasing estrogen receptor binding sites and SHBG. The negative impact on the aging male should be quite clear.29,30 I recommend all older patients take between 50 and 100 mg of chelated zinc daily (zinc gluconate), not only to optimize testicular function, but to obtain the other additional benefits seen with immune function, enhanced healing, and sharpened senses of taste and smell.
Dehydroepiandrosterone
Dehydroepiandrosterone (DHEA) levels fall significantly with age, whereas adrenal cortisol production rises. The supplementation of 100 mg of DHEA to older men and women was studied and resulted in lower SHBG levels, higher free testosterone levels, increased IGF-I levels, and a youthful DHEA/cortisol ratio. No side effects were noted in either sex.31 In another recent study dehydroepiandrosterone sulfate (DHEAS) serum levels were negatively correlated with patient age, sexual function score, total wellness score (an index of general well-being), and PSA.32,33 DHEA is an independent variable apart from testosterone related to erectile dysfunction in diabetics.33,34 DHEA is inversely correlated with bone mineral density in elderly men.35 Because of the positive benefits of DHEA administration on free testosterone and a heightened IGF-I, and the correlations with male wellness and DHEA levels, I favor recommending 100mg daily of DHEA for older men, irrespective of testosterone levels. Because DHEA taken orally affects hydrocortisone levels, taking it in the evening results in a modest lowering of cortisol levels compared with morning ingestion.36
As previously mentioned, decreased carbohydrate intake and increased protein intake, irrespective of fat (HPLC diet), lowers insulin secretion, which promotes higher testosterone levels, improved insulin sensitivity, and weight loss. I strongly recommend this diet for men, particularly for those with metabolic obesity syndrome. Whether or not adherence to this diet is possible, exercising men can optimize training benefits, particularly weight training, by ingesting 1.5 to 2.0 g of protein per kilogram of body weight daily. Chromium picolinate (200 to 400µg/day) improves insulin sensitivity,37 improves diabetic status, negates the adverse effects of glucocorticoids,38 and even increases lean body mass.39 I recommend that older men take 400µg daily, irrespective of diet.
HGH levels can be increased with high-intensity training, although this effect is not seen with testosterone. Scattered reports suggest increases in testosterone levels might occur with exercise, but this is explained by the immediate hemoconcentration, secondary to transmigration of plasma water into exercising muscle, and secondary to increasing osmolality. HGH levels put out by the pituitary during exercise decrease with age, but still are significant. Higher intensity weight training, followed by high-intensity interval aerobic activity, results in substantial increases in HGH production, mirrored by higher IGF-I levels. IGF-I appears to be the principal mediator of the action of growth hormone, whereas IGF-II has more insulin-like activity. The principal anabolic actions of IGFs include stimulation of amino acid transport; stimulation of DNA, RNA, and protein synthesis; and induction of cell proliferation and growth. IGF-I is directly responsible for chondrogenesis, skeletal growth, and the growth of soft tissue. Linear growth is stimulated by affecting cartilaginous growth areas of long bones. Growth is also stimulated by increasing the number and size of skeletal muscle cells, influencing the size of organs, and increasing red cell mass through erythropoietin stimulation. These indirect effects are inhibited by glucocorticoids.
Low-level aerobic training has virtually no effect on HGH secretion.40 Because DHEA levels augment hepatic synthesis of IGF-I, taking DHEA and engaging in high-intensity exercise are the best strategies for increasing the trophic benefits of HGH, short of taking the hormone itself, which has substantial benefits in older men with low levels, even enhancing insulin sensitivity.41
The Benefits of Regular Physical Activity on the Aging Process
The benefits of regular physical activity, consisting of both aerobic and anaerobic exercises, has gained scientific support in the medical literature.42–45 The value of such programs extends beyond measures of disease prevalence and life expectancy. Exercise can markedly increase the quality of life for an individual by removing physical constraints and providing the necessary endurance and strength needed to participate in and enjoy numerous activities, even as one ages (Appendix 2). The aging process, as a naturally occurring phenomenon, does not place limitations on the adaptations to training of cardiovascular endurance and muscular strength that were thought to exist. Rather, the decrease in these parameters may reflect more an inactive lifestyle (Appendix 3). Indeed the changes commonly associated with aging parallel the changes seen with inactivity or even with weightlessness in space (Table 8–1, Appendix 1).
Cardiovascular/respiratory |
Decrease in Vo2 max |
Decrease in cardiac output |
Decrease in stroke volume |
Decrease in maximum heart rate |
Increase in systolic blood pressure |
Decrease in body water |
Decrease in vital capacity and maximal ventilation |
Blood |
Decrease in red blood cell mass and lymphocytes |
Decrease in fibrinolytic capacity |
Increase in total cholesterol and triglycerides |
Body composition/musculoskeletal |
Decrease in lean body weight (muscle loss) |
Increase in fat weight |
Decrease in bone mineral mass |
Decrease in joint flexibility |
Metabolic/regulatory |
Decrease in bowel function |
Disordered glucose tolerance |
Decrease in activity of sympathomedullary axis |
Decrease in serum androgen levels |
Decrease in immune system function |
Nervous system |
EEG abnormalities |
Sleep pattern disorders |
Deterioration of sense organ function |
Deterioration of intellectual capacity (cognition, memory) |
Adapted from Bortz WM. Disuse and aging. JAMA 1992;248:1203–1208, with permission.
Cardiovascular endurance has been established as a measure of fitness that allows one to make assumptions pertaining to the general level of physical activity an individual is able to endure. Higher levels of physical activity, as demonstrated by higher levels of physical fitness, appear to decrease all-cause mortality, primarily due to lower rates of cardiovascular disease and cancer.46 Lower levels of physical fitness are associated with a higher risk of death from coronary heart disease and cardiovascular disease in clinically healthy men, independent of other major coronary risk factors.43
In addition to the positive effects of regular physical activity on morbidity and mortality, improved cardiovascular endurance and muscular strength have numerous practical implications when applied to lifestyle and physical activity. This concept is especially applicable to the aging population, which not only may lose the capability of participating in previously enjoyable activities, but also, more importantly, may lose the ability to maintain functional independence. The decline in strength and endurance with aging may also expose these individuals to significant dangers, such as falls, with their associated morbidity and mortality.47,48
After the age of 25 years, a decrease in the maximal capacity of the cardiovascular system to maintain an adequate oxygen supply to the exercising muscles occurs with advancing age, as evidenced by a progressive decline in maximal oxygen uptake (VO2max).49,50 This regression occurs as a result of several factors found with aging: decreased maximum heart rate, decreased stroke volume, decreased cardiac contractility, increased body mass, and decreased level of physical activity (Table 8–1). Until recently, many people questioned the trainability of older individuals and the extent to which their physical fitness could be modified. The decrease in aerobic power was felt to be a naturally occurring phenomenon that explained the limitations placed upon the elderly and the limited range of activities in which they could comfortably and safely participate.
In a study using sedentary men between 49 and 65 years of age, Pollock et al50 demonstrated a significant improvement in VO2max of 18%, from 31.0 to 36.8 mL/kg/min, following 20 weeks of aerobic training. These men trained for 30 minutes, 3 days a week, at an intensity of 80 to 90% of their maximum heart rate. A nonexercising control group showed no significant changes over the same time period. The investigators concluded not only that these men responded favorably to endurance exercise, but also that the improvement was similar in magnitude to that found in previous investigations of similar design with younger subjects.
The ability to endurance train individuals even older than the subjects used in the aforementioned study was previously not well understood, but felt to be negligible. Early investigations demonstrated nonsignificant to moderate changes in VO2max in response to training in individuals over 60 years of age, depending upon the intensity of training and the length of the program.51,52 Several studies have further examined these earlier findings and have demonstrated a significant improvement in VO2max in subjects not only in the 60- to 69-year age range, but also even in older individuals.53,54 In the study by Seals et al,54 11 subjects, with a mean age of 63 years, were evaluated before training, after 6 months of low-intensity training (∼40% of heart rate reserve for 20 to 30 minutes, 3 days a week), and, finally, after an additional 6 months of higher intensity training (up to 85% of the heart rate reserve for 30 to 45 minutes, 3 days a week). VO2max increased significantly, following low-intensity and high-intensity training (+30%). VO2max was 25.2 mL/kg/min before training, 28.2 mL/kg/min after low-intensity training, and 32.9 mL/kg/min after high-intensity training. These findings demonstrated that individuals older than 60 could adapt to and benefit from prolonged endurance training with a large increase in aerobic power.
In a study of even older individuals, Hagberg et al53 demonstrated improvement in cardiovascular endurance with training. Sixteen subjects, whose ages ranged from 70 to 79 years, participated in an exercising program consisting of three sessions per week for 26 weeks (40 minute per session at 50 to 70% VO2max initially for the first 13 weeks and then at 75 to 85% VO2max for the last 13 weeks of training).
These subjects increased their VO2max by 16%, from 22.5 to 25.8 mL/kg/min, during the first 13 weeks of training and by a total of 22% after 26 weeks of training, with a final VO2max of 27.1 mL/kg/min. Therefore, this study demonstrated that healthy men and women in their 70s can respond to prolonged endurance exercise training with adaptations similar to those in younger individuals.
Though improvements in cardiovascular endurance are clearly noted in elderly individuals following a conditioning program, the effects of a long-term routine of physical activity on the rate of decline in aerobic power requires further delineation. Several earlier studies demonstrated a decline in VO2max despite habitual exercise, though fitness levels in the exercisers remained significantly higher compared with sedentary controls.55,56 In a longitudinal study on 16 men initially between the ages of 32 to 56 years (mean 44.6), Kasch and Wallace57 found essentially no change in VO2max over a 10-year period of aerobic conditioning. These subjects trained 3 days a week, 12 months a year by running, swimming, or a combination of running and swimming. The duration of the activity session ranged from 20 to 105 minutes (mean 59 minutes) and intensity ranged from 60 to 95% (mean 86%) of maximal physical work capacity by heart rate.
In another study, Kasch et al58 longitudinally followed two groups of men: 15 exercisers were followed for 23 years, from a mean age of 44.6 to 68.0, and 15 nonexercising control subjects were followed for 18 years, from a mean age of 51.6 to 69.7. Physical fitness, as measured by VO2max, declined 13% in the exercisers, from 44.4 to 38.6mL/kg/min and, in the nonexercisers, it declined 41%, from 34.2 to 20.3 mL/kg/min (Table 8–2). The combination of a higher level of fitness and a lower rate of decline would allow the active subjects to comfortably and safely participate in a wider range of physical pursuits when these results are compared with the MET (metabolic equivalent rate for any given person) requirements of selected activities (Fig. 8–1). The decline in VO2max of the nonexercisers was similar to that reported by Dehn and Bruce.59 Habitual aerobic exercise retarded the usual loss of VO2max thought to characterize aging (Table 8–3).
A decline in muscle strength appears to be one of the more predictable characteristics of the aging process and creates difficulty performing the activities of daily living.60 Decreased levels of trophic hormones, decreased neuromuscular coordination, decreased muscle mass, and low physical activity are several contributing factors to this decline (Table 8–2). This loss of strength and its associated loss of function was previously felt to be a natural consequence of aging and, with that assumption, the ability of a strength training program to improve strength in the elderly was thought to be minimal. As with endurance training, recent studies have further explored this previously held belief.
Using 12 healthy untrained volunteers (age range 60–72 years) participating in a 12-week strength-training program, Frontera et al61 demonstrated significant increases in strength. The participants performed eight repetitions per set, three times a week at 80% of their one repetition maximum (1 RM) for extensors and flexors of both knee joints. At the end of the program, the subjects improved their strength by both isotonic (1 RM) and isokinetic measurements (Cybex II dynamometer measuring isokinetic peak torque). Upon further analysis, the rate of dynamic strength gain per training session of these elderly men was similar to the 4.4 to 5.6% increases in dynamic strength per session observed in young men (average age 28 years) after a similar training protocol.62 A well-designed strength training program of appropriate intensity, similar in nature to standard rehabilitation techniques, caused a marked gain in strength in older men.
In a study using individuals in an older age group in an institutional setting, 10 frail, institutionalized volunteers aged 90 ± 1 years under took 8 weeks of high-intensity resistance training program (eight repetitions per set, three sets per session, three sessions per week, beginning at 50% 1 RM, then increasing to 80% RM after 1 week).63 After 8 weeks of training, strength gains averaged 174% ± 31% (mean ± standard error of the mean, SEM) in the nine subjects who were able to complete the training program. Therefore, high-resistance weight training led to significant gains in muscle strength, size, and functional mobility among frail residents of nursing homes up to the age of 96 years (Table 8–4).64
Whether the improved muscle strength demonstrated in elderly individuals placed on a regular program of strength-training results in improved quality of life as indicated by a greater degree of independence and reduced rate of falls has not been studied, though one could postulate a positive correlation. Furthermore, the effects of a sustained program of resistive-training on the rate of decline in muscle strength is not yet known and would have significant clinical implications in terms of prescribing a well-rounded program of physical activity, combining aerobic as well as anaerobic exercise training.
Both cardiovascular endurance and muscle strength have been shown to increase in the elderly population following a program of regular exercise. Regardless of the positive effects on improved rates of morbidity and mortality, the improvement in VO2max and strength allows individuals to actively participate in a broader range of physical activities as they age. The effect allows the elderly to maintain an active and independent role in society and decreases the burden of care on the younger generation. Most importantly, the positive effects of a well-designed exercise program remove the limitations placed on the elderly by a sedentary lifestyle and allows participation in some of the more pleasurable and vigorous activities of life. Exercise programs can be designed to minimize the effects of aging, both perceived and real, and also overcome some limitations imposed by preexisting disease, such as arthritis.
Conclusion
The synergy between optimal androgen status, optimal nutrition, and optimal physical activity in the aging male can be seen. An integrated approach has yet to be tried on a large scale. I believe better results for health can be expected in the aging male, when all three are addressed simultaneously rather than each individually. We are at societal edge where a brave few are testing the waters. Hopefully, a prospective study will confirm my belief.
Appendix 1: Physiological Changes During Aging
I. Eyes
A. Presbyopia: less able to focus on near objects
B. Light reduction
1. 33% as much light reaches retina
2. Causes
a. Yellowing of lens, sclera, vitreous humor.
b. Reduction in size of pupils
c. Entropion
II. Ears
A. Presbycusis
1. Lose ability to hear higher frequencies
2. Secondary to retrocochlear and inner ear degeneration
B. Conductive hearing loss: stiffening of connective tissue
III. Cardiovascular
A. Loss of elasticity in arteries as collagen cross-linkages stabilize with maturity
1. Increased peripheral resistance
2. Slight increase in blood pressure
a. Age 20–24: females 116/70, males 122/76
b. Age 60–64: females 142/85, males 140/85
B. Heart
1. Cardiac output
a. Rate of decline
(1) Decreases 40% between 3rd and 8th decades
(2) A little less than 1%/year
b. Components
(1) Decreased responsiveness to β-adrenergic modulation: lower maximum heart rate
(a) Young adult, 195 beats/min
(b) Average 65 year old, max 170/min
(2) Stroke volume decreases
2. A-Vo2 difference decreases
3. Cardiac weight remains constant: left ventricular wall up to 25% thicker at age 80 than at age 30
4. No significant increase in collagen or reticulin
IV. Pulmonary—lungs
A. Reduced expandability
B. Abdominal musculature becomes responsible for much of the respiratory excursion of diaphragm
C. Less efficient gas exchange at alveolar level
1. Loss of alveolar tissue relative to air passages
2. Stickier mucus
D. Changes in lung volumes and capacities
1. Residual volume: increases 100% between 3rd and 9th decades
2. Vital capacity: decreases by 17 to 22mL/yr
3. Forced expired air volume in 1 second (FEV1): falls progressively: males, 32 mL/yr; females, 25mL/yr
4. Maximal breathing capacity: reduced by 40% between 20 and 80 years
V. Renal—kidney
A. Weight: age 60, 250 g; age 70, 230 g; age 80, 190 g
B. Renal function usually decreases
1. Reduced renal blood flow: 2nd decade, 670 mL/min: 8th decade, 350mL/min
2. Decreased number of functioning nephrons: number of glomeruli per kidney
a. Birth–age 40, 500,000–1 million
b. By 7th decade 1/3 to ½ less
3. Glomerular filtration rate: decreases 46% from age 20 to age 90
4. Maximum specific gravity of urine: youth, 1.032; age 80, 1.024.
C. 33% of elderly persons have normal renal function
VI. Vascular compartment
A. Markedly reduced total body water
1. Volume of extracellular fluid remains about same
2. Intracellular fluid volume reduced
3. Red blood cell mass decreased, lower growth hormone, lower testosterone
B. Decreased “tissue” percentage
C. Increased body fat
VII. Central nervous system
A. Difficult to define what constitutes average changes in brain function with increasing age
B. Some loss of short-term memory and some decline in ability to learn new skills: given time, able to acquire new knowledge and skills
C. Nerve conduction in peripheral nervous system slows
D. Brain
1. Weight decrease of 10 to 12% during normal long life
2. Gray/white matter ratio
a. Age 20, 1.28:1
b. Age 50, 1.13:1
c. Age 100, 1.33:1
3. Decline in cerebral blood flow
a. Age 17–18, 79.3 mL/min/100 g brain tissue
b. Age 57–99, 47.7 mL/min/100 g brain tissue
4. Neurofibrillary tangles and senile plaques
a. Up to age 50, uncommon
b. Age 90, found in 90%
VIII. Bone—skeleton
A. Rate of bone loss/decade
1. Men, 3%
2. Women, 8%
B. Average height loss: age 65–75, 1.5%; age 85–94, 3%
C. Osteoporosis
1. Generic term referring to a state of decreased mass per unit volume (density) of normally mineralized bone
2. Risk factors
a. Race and heredity
b. Nutrition
c. Endocrine factors
d. Weight-bearing activity
(1) As much as 30 to 40% of initial total bone mass may be lost following 6 months of complete immobilization
(2) Disuse osteopenia
IX. Musculoligamentous
A. Cells making up voluntary muscle: decrease by 50% at age 80
B. Weight of skeletal muscle
1. Age 21–30, 45% of body weight
2. Age 70+, 27% of body weight
C. No change in muscle fibers or motor units
1. Loss in muscle mass parallel to loss of strength
2. Higher percentage of type I fibers
D. Changes in collagen
1. Increased stability to thermal denaturing and reduced solubility
2. Collagen fibers possess all of its cross-linkages shortly after synthesis
a. With maturation, reducible cross-linkages gradually stabilize
b. Physical activity enhances rate of collagen turnover
E. Tendons and ligaments
1. Increased stiffness with decreased rate of collagen turnover
2. Osseous resorption at sight of ligamentous attachment
3. Decreased cellular component of ligaments and tendons
4. Decreased collagen fiber bundle thickness
5. Reduced capillarization
6. Increased extensibility per unit length
7. Decreased glycosaminoglycans
8. Reduced water content
Note: The final result is less elastic stiffer ligaments/tendons that have more cross-linked collagen.
Appendix 2: Exercise and Aging
I. Benefits reported in elderly who exercise
A. Improved cardiovascular and respiratory function
B. Reduced risk of coronary artery disease
C. Decreased body fat
D. Increased lean body and bone mass
E. Better work capacity
G. Reduced susceptibility to depression H. Increased self-esteem and quality of life
II. Components of physical fitness
A. Cardiovascular endurance
B. Muscle strength
C. Muscle endurance
D. Flexibility
E. Body composition
III. Cardiovascular endurance and aging
A. Progressive decline in maximal oxygen up take (VO2max) noted
1. Decreased maximum heart rate
2. Decreased stroke volume
3. Decreased cardiac contractility
4. Increased body mass
5. Decreased level of physical activity
B. Adaptability to training
1. Increase in VO2max noted in elderly individuals (Table 8–1)
2. Habitual aerobic exercise may retard the usual loss of VO2max characteristic of the aging process (Table 8–2)
IV. Muscle strength and endurance and aging
A. Predictable decline in strength
1. Decreased levels of trophic hormones
2. Decreased neuromuscular coordination
3. Decreased muscle mass
4. Low physical activity
B. Loss of strength with aging creates difficulties performing activities of daily living
C. Significant gains in strength noted in elderly following strength-training program (Table 8–4)
D. Association between improved strength and greater degree of independence/reduced rate of falls is not yet known: positive correlation with weakness and falling has been shown
Appendix 3: Average Biological Functional Changes Between the Ages of 30 and 70
I. Work capacity −25 to 30%
II. Cardiac output, −30%
A. Maximum heart rate, −30 bpm
B. Blood pressure
1. Systolic, +10 to 40 mmHg
2. Diastolic, −5 to 10 mmHg
III. Respiration
A. Vital capacity, −40 to 50%
B. Residual volume, −30 to 50%
IV. Basal metabolic rate, −8 to 12%
V. Musculature
A. Muscle mass, −25 to 30%
B. Hand grip strength, −25 to 30%
C. Nerve conduction velocity, −10 to 15%
VI. Flexibility, −20 to 30%
VII. Bone
A. Women, −25 to 30%
B. Men, −15 to 20%
VIII. Renal function, −30 to 50%
REFERENCES
1. Shippen E, Fryer W, Evans S. Testosterone Syndrome. The Critical Factor for Energy, Health, and Sexuality—Reversing the Male Menopause. New York, NY: M. Evans and Co., Inc.; 1998
2. Barrett-Connor E, von Mhlen DG, Kritz-Silverstein D. Bioavailable testosterone and depressed mood on older men: the Rancho-Bernardo Study. J Clin Endocrinol Metab 1999;84:573–577
3. Lamberts SW, van den Beld AW, van der Lely A. The endocrinology of aging. Science 1997;278:419–424
4. Prasad AS, Mantzaros CS, Beck FW, Hess JW, Brewer CJ. Zinc status and serum testosterone levels of healthy males. Nutrition 1996;12:344–348
5. Fiatarone MA, O’Neill EF, Ryan ND, et al. Exercise training and nutritional supplementation for physical frailty in very elderly people. N Engl J Med 1994;330:1769–1775
6. Penninx BWJH, Messier SP, Rejeski WJ, et al. Physical exercise and the prevention of disability in activities of daily living in older persons with osteoarthritis. Arch Intern Med 2001;161:2309–2316
7. Singh MAF. Exercise comes of age: rationale and recommendations for a geriatric exercise prescription. J Gerontol A Biol Sci Med Sci 2002;57:M262–M282
8. Bortz WM 2nd. Disuse and aging. JAMA 1992;248:1203–1208
9. Aniansson A, Zetterberg C, Hedberg M, Henriksson KG. Impaired muscle function with aging. Clin Orthop 1984;191: 193–201
10. Singh MAF. Combined exercise and dietary intervention to optimize body composition in aging. Ann NY Acad Sci 1998; 854:378–393
11. Bagatell CJ, Bremner WJ. Androgens in men—uses and abuses. N Engl J Med 1996;334:707–714
12. Bardin CW. The anabolic action of testosterone. N Engl J Med 1996;335:52–53
13. Bhasin S, Storer TW, Berman N, et al. Testosterone replacement increases fat-free mass and muscle size in hypogonadal men. J Clin Endocrinol Metab 1997;82:407–413
14. Segal RJ, Reid RD, Courneya KS, et al. Resistance exercise in men receiving androgen deprivation therapy for prostate cancer. J Clin Oncol 2003;21:1653–1659
15. Bhasin S, Storer TW, Berman N, et al. The effects of supraphysiologic doses of testosterone on muscle size and strength in normal men. N Engl J Med 1996;335:1–7
16. Abate N, Haffner SM, Garg A, Peshock RM, Grundy SM. Sex steroid hormones, upper body obesity, and insulin resistance. J Clin Endocrinol Metab 2002;87(10):4522–4527
17. Tchernof A, Labrie F, Belanger A, et al. Relationships between endogenous steroid hormone,sex hormone-binding globulin and lipoprotein levels in men: contribution of visceral obesity, insulin levels and other metabolic variables. Atherosclerosis 1997;133(2):235–244
18. Morris KL, Namey TC, Zemel MB. Effects of dietary carbohydrate on the development of obesity in heterozygous Zucker rats. J Nutr Biochem 2003;14(1):32–39
19. Foster GD, Wyatt HR, Hill JO, et al. A randomized trial of a low-carbohydrate diet for obesity. N Engl J Med 2003; 348:2082–2090
20. Samaha FF, Iqbal N, Seshadri P, et al. A low-carbohydrate as compared with a low-fat diet in severe obesity. N Engl J Med 2003;348:2074–2081
21. Thompson WG, Namey TC. Cardiovascular complications of inactivity. Rheum Dis Clin North Am 1990;16(4):803–813
22. Alexandersen P, Haarbo J, Christiansen C. The relationship of natural androgens to coronary heart disease in males: a review. Atherosclerosis 1996;125(1):1–13
23. De Pergola G, Pannacciulli Niccone M, Tartagni M, Rizzon P, Giorgino R. Free testosterone plasma levels are negatively associated with the intima-media thickness of the common carotid artery in overweight and obese glucose-tolerant young adult men. Int J Obes Relat Metab Disord 2003;27(7): 803–807
24. English KM, Mandour O, Steeds RP, Diver MJ, Jones TH, Channer KS. Men with coronary artery disease have lower levels of androgens than men with normal coronary angiograms. Eur Heart J 2000;21(11):890–894
25. Rosano GM, Leonardo F, Pagnotta P, et al. Acute anti-ischemic effect of testosterone in men with coronary artery disease. Circulation 1999;99(13):1666–1670
26. Hamdi SA, Nassif OI, Ardawi MS. Effect of marginal or severe dietary zinc deficiency on testicular development and functions of the rat. Arch Androl 1997;38(3):243–253
27. Li Y. A study on the relationships among plasma testosterone, sex development and serum zinc in normal boys aged 10–15. Zhonghua Yu Fang Yi Xue Za Zh. 1992;3:148–150
28. Prasad AS. Zinc in growth and development and spectrum of human zinc deficiency. J Am Coll Nutr 1988;7(5):377–384
29. Prasad AS, Mantzoros CS, Beck FW, Hess JW, Brewer GJ. Zinc status and serum testosterone levels of healthy adults. Nutrition 1996;12(5):344–348
30. Om AS, Chung KW. Dietary zinc deficiency alters 5 alpha-reduction and aromatization of testosterone and androgen and estrogen receptors in rat liver. J Nutr 1996;126(4):842–848
31. Okubo T, Truong TK, Yu B, et al. Down-regulation of promoter 1.3 activity of the human aromatase gene in breast-zinc finger protein (SnaH). Cancer Res 2001;61(4):1338–1346
32. Morales AJ, Haubrich RH, Hwang JY, Asakura H. The effect of six months treatment with a 100 mg daily dose of dehydroepiandrosterone (DHEA) on circulating sex steroids, body composition and muscle strength in age-advanced men and women. Clin Endocrinol (Oxf) 1998;49(4):421–432
33. Ponholzer A, Plas E, Schatzl G, Jungwirth A, Madersbacher S. Association of DHEA-S and estradiol serum levels to symptoms of aging men. Aging Male 2002;5(4):233–238
34. Alexopoulou O, Jamart J, Maiter D, et al. Erectile dysfunction and lower androgenicity in type 1 diabetic patients. Diabetes Metab 2001;27:329–336
35. Clarke BL, Ebeling PR, Jones JD, et al. Predictors of bone mineral density in aging healthy men varies by skeletal site. Calcif Tissue Int 2002;70:137–145
36. Kroboth PD, Slalek FS, Pittenger AL, et al. DHEA and DHEAS: a review. J Clin Pharmacol 1999;39:327–348
37. Anderson RA, Cheng N, Bryden NA, et al. Elevated intakes of supplemental chromium improve glucose and insulin variables in individuals with type 2 diabetes. Diabetes 1997;46: 1786–1791
38. Ravina A, Slezak L, Mirsky N, et al. Reversal of corticosteroid induced diabetes mellitus with supplemental chromium. Diabet Med 1999;16:164–167
39. Pasman WJ, Westerterp-Plantenga MS, Saris WH. The effectiveness of long-term supplementation of carbohydrate, chromium, fibre, and caffeine on weight maintenance. Int J Obes Relat Metab Disord 1997;21:1143–1151
40. Stuerenburg HJ, Jung R, Kunze K. Age effects on growth hormone and testosterone responses to endurance exercise in patients with neuromuscular diseases. Arch Gerontol Geriatr 1999;1:45–51
41. Svensson J, Fowelin J, Landin K, Bengtsson BA, Johansson JO. Effects of seven years of GH-replacement therapy on insulin sensitivity in GH-deficient adults. J Clin Endocrinol Metab 2002;87:2121–2129
42. Blair SN, Kohl HW, Paffenbarger RS, Clark DG, Cooper KH, Gibbons LW. Physical fitness and all-cause mortality: a prospective study of healthy men and women. JAMA 1989;262:2395–2401
43. Ekelund LG, Haskell WL, Johnson JL, Whaley FS, Criqui MH, Sheps DS. Physical fitness as a predictor of cardiovascular mortality in asymptomatic North American Men. N Engl J Med 1988;319:1379–1384
44. Paffenbarger RS, Hyde RT, Wing AL, Steinmetz CH. A natural history of athleticism and cardiovascular health. JAMA 1984;252:491–495
45. Paffenbarger RS, Hyde RT, Wing AL, Hsieh CC. Physical activity, all-cause mortality, and longevity of collge alumni. N Engl J Med 1986;314:605–613
46. Blair SN, Kohl HW, Paffenbarger RS, Clark DG, Cooper KH, Gibbons LW. Physical fitness and all-cause mortality: a prospective study of healthy men and women. JAMA 1989;262:2395–2401
47. Aniansson A, Zetterberg C, Hedberg M, Henriksson KG. Impaired muscle function with aging. Clin Orthop 1984;191: 193–201
48. Baker SP, Harvey AH. Fall injuries in the elderly. Clin Geriatr Med 1985;1:501–512
49. Heath GW, Hagberg JM, Ehsani AA, Holloszy JO. A physiological comparison of young and older endurance athletes. J Appl Physiol 1981;51:634–640
50. Pollock ML, Dawson GA, Miller HS, et al. Physiologic responses of men 49 to 65 years of age to endurance training. J Am Geriatr Soc 1976;24:97–104
51. DeVries H. Physicological effects of an exercise training regimen upon men aged 52 to 88. J Gerontol 1970;25:325–336
52. Souminen H, Heikkinen E, Parkatti T. Effect of eight weeks’ physical training on muscle and connective tissue of the M. Vastus lateralis in 69-year-old men and women. J Gerontol 1977;32:33–37
53. Hagberg JM, Graves JE, Limacher M, et al. Cardiovascular responses of 70- to 79-yr-old men and women to exercise training. J Appl Physiol 1989;66:2589–2594
54. Seals DR, Hagberg JM, Hurley BF, Ehsani AA, Holloszy JO. Endurance training in older men and women, I: cardiovascualr responses to exercise. J Appl Physiol 1984;57:1024–1029
55. Pollock ML, Miller HS, Wilmore J. Physiological characteristics of champion American track athletes 40 to 75 years of age. J Gerontol 1974;29:645–649
56. Robinson S, Dill DB, Robinson RD, Tzankoff SP, Wagner JA. Physiological aging of champion runners. J Appl Physiol 1976;41:46–51
57. Kasch FW, Wallace JP. Physiological variables during 10 years of endurance exercise. Med Sci Sports 1976;8:5–8
58. Kasch FW, Boyer JL, Schmidt PK, et al. Ageing of the cardiovascular system during 33 years of aerobic exercise. Age Ageing (England) 1999;28:531–536.
59. Dehn MM, Bruce RA. Longitudinal variations in maximal oxygen intake with age and activity. J Appl Physiol 1972;3s3: 805–807
60. Larsson L, Grimby G, Karlsson J. Muscle strength and speed of movement in relation to age and muscle morphology. J Appl Physiol 1979;46:451–456
61. Frontera WR, Meredith CN, O’Reilly KP, Knuttgen HG, Evans WJ. Strength conditioning in older men: skeletal muscle hypertrophy and improved function. J Appl Physiol 1988;64:1038–1044
62. Rutherford OM, Greig CA, Sargeant AJ, Jones DA. Strength training and power output: transference effects in the human quadriceps muscle. J Sports Sci 1986;4:101–107
63. Fiatarone MA, Marks EC, Ryan ND, Meredith CN, Lipsitz LA, Evans WJ. High-intensity strength training in nonagenarians. JAMA 1990;263:3029–3034
64. Namey TC. Exercise and arthritis. Adaptive bicycling. Rheum Dis Clin North Am 1990;16:871–886
< div class='tao-gold-member'>
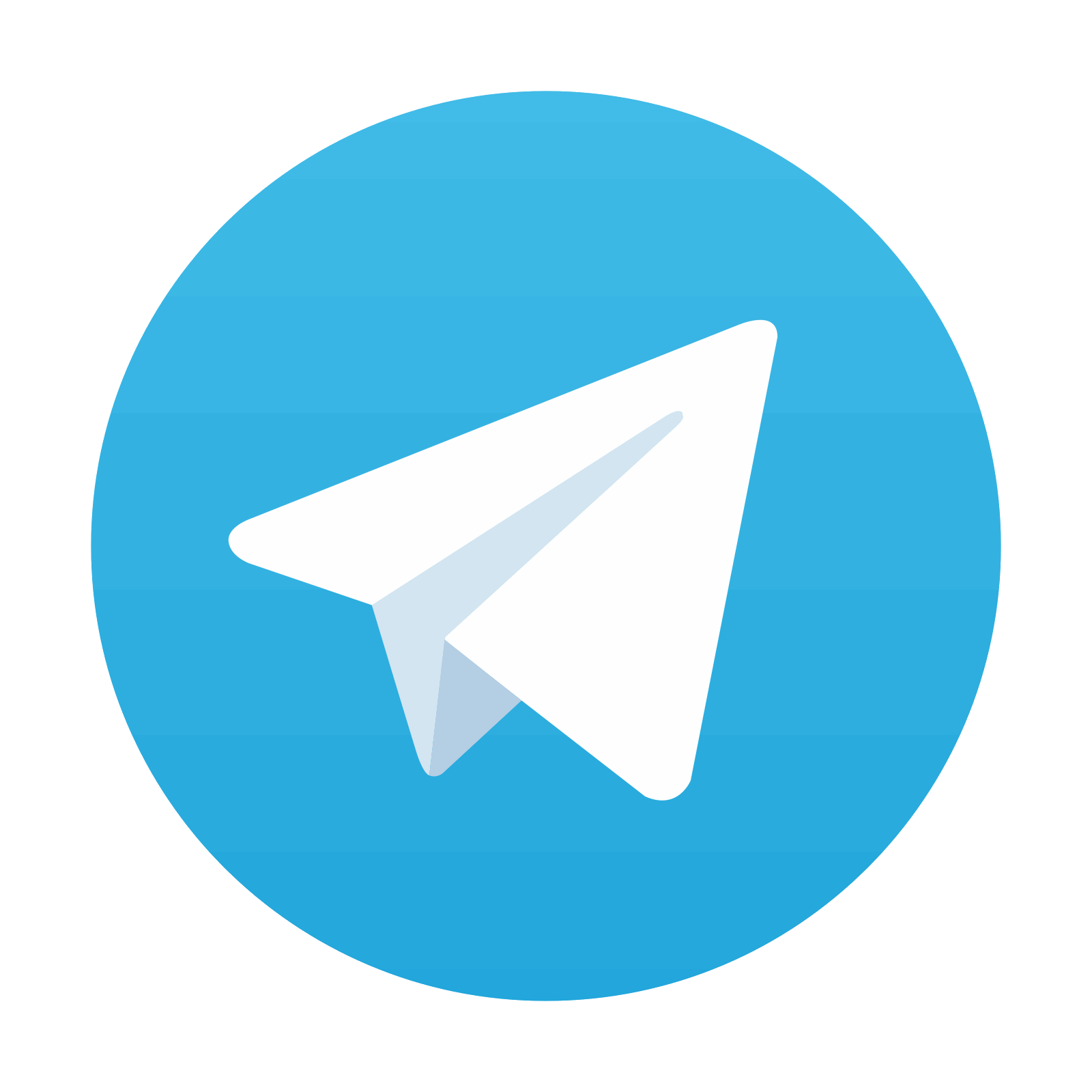