10
Effect of Androgens on Muscle, Bone, and Hair in Men
Considerable scientific effort has been directed toward the study of the aging man, with particular attention to the role of androgen replacement for the maintenance of muscle, bone, and hair. This chapter examines how the normal progression of aging affects these processes, and provides a clinical perspective for the use of androgen therapy to treat age-associated muscle, bone, and hair loss.
Case History
A 66-year-old man presents for his yearly examination. He has a medical history of hypertension and hypothyroidism, both diagnosed 5 years ago. He states that he is in his usual state of health but does notice worsening fatigue. On further questioning, he complains of not being able to play tennis as often because of exhaustion by mid-afternoon. He admits to depressive symptoms, decreased sexual desire, and inability to maintain erections during the last 9 months. His hypertension has been well controlled with a thiazide diuretic and his hypothyroidism has been managed by a stable dose of levothyroxine. On review of systems, the patient also complains of a 10-pound weight gain over 9 months, and he has noticed decreased beard growth and decreased muscle strength. He is happy that his frontal hair loss has slowed. Pertinent exam findings include a well-nourished Caucasian male that appears his stated age. He has frontal and vertex scalp hair thinning with occipital sparing, no thyroid tissue palpable, and appears noticeably more frail as compared with last year’s exam. On testicular exam, no masses are palpable but his testicles are decreased in size and softer than previous exams. Laboratory data are listed in Table 10–1.
Initially, the patient’s physical findings indicate hypothyroidism; however, laboratory data reveal his total and free testosterone levels are below normal.
The patient is concerned that his recent loss of muscle mass and strength may impair his ability to perform his activities of daily living, and he is right to be concerned.
Androgens and Muscle
Muscle Loss and Aging
Aging is associated with a progressive deterioration of many physical processes. Changes include the loss of skeletal muscle mass and a decrease in functional capacity.1 In the aging man, the mechanisms responsible for these physical changes, collectively referred to as sarcopenia, are multifactorial but are likely facilitated by the adoption of a more sedentary lifestyle, a less than optimal diet, and a gradual decline in gonadal function.2,3
The relationship between hypogonadism or andropause and the sarcopenic process poses a major health concern for aging males.4–6 Although multifaceted, sarcopenia is ultimately the result of a chronic imbalance between muscle protein synthesis and breakdown.7 The resultant loss of contractile protein mass and quality contributes to muscle weakness and is a key contributing factor in fall-related injury and disability. Consequently, the ability to counteract progressive losses in skeletal muscle mass and strength in older men has considerable health and economic implications. By attenuating or even preventing these losses, older individuals may have an improved quality of life, prolonged independent living, and a significantly reduced dependence on structured health care. There are also metabolic consequences of sarcopenia to be considered. These include, but are not limited to, thermoregulatory disruption,8 glucose intolerance,9 and altered lipid metabolism.10,11 Thus, as the population of older men increases, the need to develop therapies to counteract losses in skeletal muscle mass and strength with aging takes on added significance.
A large number of strategies designed to ameliorate the effects of sarcopenia have been studied. Appropriately, many of these have focused on voluntary lifestyle changes. Older individuals have been encouraged to become more active, exercise regularly, modify their diets, and take nutritional supplements. When applied and monitored carefully, these lifestyle interventions often show promising results.12–15 However, there is evidence that the effectiveness of strength training in older individuals is positively correlated with serum testosterone concentration,16 and in many instances exercise and dietary interventions fail due to poor adherence and insufficient supervision.17 Further, compromised joint integrity or balance, or frailty may restrict the intensity and type of physical activity that elderly individuals can perform.1
It is estimated that 50% of men over the age of 50 are hypogonadal.18,19 Although a downregulation in gonadal function does not necessarily result in a symptomatic state, many individuals are symptomatic and may benefit from androgen replacement therapy. Positive outcomes may include a decrease in muscle protein breakdown,20 an increase in muscle protein synthesis,10,21 enhanced skeletal muscle mass,20,22 and increased muscle strength.23,24
Unlike menopause in women, andropause does not occur in all older men and its presentation and pathophysiology may vary considerably.5 Decreased potency and libido, increased fatigability, and decreased muscle strength are clinical features associated with andropause. However, a significant reduction in serum testosterone concentration represents the core physiological event and is a key diagnostic element in those affected by andropause.
Although age is undoubtedly a factor, advanced age per se is not the sole prerequisite for andropause. For example, when a gonadotropin-releasing hormone analog is administered to eugonadal young men, serum testosterone concentrations fall and lean body mass and muscle strength are also lost.25 Further, many chronically institutionalized older men have lower serum testosterone concentrations26 compared with their healthy age-matched counterparts. Thus, although there is no definitive age of onset of andropause, a significant endogenous reduction in testosterone can occur as early as the fifth decade with the lowest androgen levels typically seen in men 70 years and older.3,27,28 Although there is an abundance of evidence indicating a link between lower serum testosterone concentrations and reduced skeletal muscle function in older men, sarcopenia also occurs in elderly women. Clearly, testosterone alone is not the only factor implicated in muscle loss.
Androgens and Muscle Protein Synthesis
When combined with exercise, the ability of exogenous androgens to promote skeletal muscle hypertrophy in eugonadal men is clearly evident. Although much of the early evidence could be considered anecdotal, the ability of androgens to increase lean muscle mass and strength in an athletic context has been apparent for more than four decades. Although the successful use of androgens in athletic populations is predicated on a rigorous concomitant diet and exercise regimen, it has been demonstrated that both testosterone and one of its synthetic analogs (oxandrolone) are capable of reducing muscle protein breakdown and inducing myotrophic effects in skeletal muscle of postabsorptive young and older men.20,23,29,30 Androgens such as testosterone clearly result in net skeletal muscle protein synthesis, and protein deposition also occurs in other tissues as a result of the androgen-induced positive nitrogen balance. However, as a cautionary note, androgens also possess well-documented androgenic and virilizing effects including stimulation of male secondary sexual characteristics, prostate hyperplasia, hirsutism, and virilization in females. This may limit their clinical use to specific patient populations such as hypogonadal men. Ultimately, if the optimal dose and timing of androgen that gives a maximal anabolic response with minimal side effects can be determined, the expectation is that androgens could be beneficial in modulating age-related sarcopenia in a broader range of patients.
Androgens and Muscle Strength
Physical strength is often compromised with increasing age.4,24 This functional decrement is accompanied by the loss of lean body mass and increase in body fat and often facilitated by lower circulating levels of serum testosterone. Evidence from a 12-year longitudinal study indicated that a reduction in muscle cross-sectional area was a major contributor to the reduction in muscle strength seen with aging.31 As noted, the adoption of a more sedentary lifestyle contributes to this loss of muscle mass; however, serum testosterone concentrations also play a regulatory role. In fact, there is a positive correlation between circulating levels of testosterone and the capacity to improve strength in older adults involved in a resistance training program.16 Further, it is generally accepted that, irrespective of age, muscle strength correlates with muscle mass. However, as an individual ages, strength often decreases to a greater extent than muscle mass. Thus, the possibility of increasing muscle strength and lean body mass via the administration of therapeutic doses of androgen alone would be of considerable benefit.
The primary goal of androgen therapy in the older man is to improve or maintain muscle strength to an extent where functional capability is also maintained or improved.1,32 However, results thus far have been equivocal. Several studies have demonstrated improved functional capacity following the administration of therapeutic doses of androgens to hypogonadal men.20,23,33,34 Others have been unable to demonstrate increases in muscle strength with testosterone administration alone.22,32 In the absence of definitive findings, it is reasonable to consider that a combination of hormone replacement therapy, physical activity, and nutritional intervention would provide the greatest opportunity for increases in muscle strength and function to be realized.
Following an exercise or androgen intervention, a desired outcome is an increase in muscle strength. However, when designing an intervention program (androgen, exercise, and/or diet) other components of fitness such as power, muscular endurance, flexibility, and balance must also be considered and targeted if the primary functional goal is to improve the ability to perform practical tasks and activities of daily living.
Summary
Life expectancy continues to increase as advances in medicine, nutrition, and technology are made. However, the importance of prolonged life expectancy per se is perhaps diminished if the quality of life is less than optimal. Many aging men experience significant functional impairment and a decreased quality of life due in part to alterations in body composition associated with the aging process. Although there is evidence to support the efficacy of androgen therapy in older men afflicted with symptoms of sarcopenia, we are far from understanding many of the underlying mechanisms. This chapter demonstrates the need for additional cross-sectional and longitudinal studies examining the timing, dosing, and mechanisms responsible for slowing progressive sarcopenia in the aging man.
Androgens and Bone
Age-Associated Osteoporosis in Men
Osteoporosis is a condition characterized by low bone mass and microarchitectural deterioration of bone tissue, with a consequent increase in bone fragility and susceptibility to fracture.35 Due to the prevalence of osteoporosis in postmenopausal women, very little attention is given to bone health in men. Nevertheless, the risk of fracture in men increases with age and ∼1.5 million men >65 years have osteoporosis, with another 3.5 million estimated to be osteopenic.36 The incidence of hip fractures in both sexes rises exponentially with age, with the majority of fractures occurring in men over the age of 80 years.37 Although lifetime bone mineral density (BMD) losses are estimated to be 30 to 40% in women, men lose an estimated 20 to 30% in their lifetime. Various estimates of the lifetime risk of osteoporotic-related fractures in men have been suggested, with some reporting as high as 25%21 and others stating the apparent lifetime risk nationwide to be ∼13%.38 Regardless, this represents a considerable portion of the male population and as such certainly represents a significant public health issue. Compounding the problem, when men do experience hip fractures, they have higher rates of morbidity and mortality than women.39 Specifically, ∼20% of male patients die of thromboembolus or infection-related to hip fractures,40 and hip fractures are not the only age-related fractures that increase with age in men. Spine, proximal humerus, pelvis, ankle, and distal forearm fractures occur more often in men as they age, although incidence rates remain lower than in women.37 These data clearly demonstrate the need to evaluate BMD status in men as part of all general health screenings.
To address the growing concern of diagnosing osteoporosis in men, the International Society for Clinical Densitometry met in 2001 for a position conference. Of particular interest was the society’s position on the application and use of T-scores in men for the assessment of BMD as currently applied to women. In women, the World Health Organization (WHO) has established a threshold for osteoporosis with bone densities of either the lumbar spine or the proximal femur of at least 2.5 standard deviations (SD) below the young adult mean. However, this threshold has not been validated for men, and recent data indicate that use of a single scale factor for T-scores in men may not be appropriate, and doing so may erroneously identify men as experiencing fractures at a higher relative BMD as compared with age-matched women.41–43 Thus it appears that a gender-specific scale must be developed to accurately assess BMD in men and women.41,43,44
Hypogonadism and Secondary Osteoporosis in Men
The maintenance of bone mass in the aging man is complicated by the decline in androgens associated with andropause. Significant reductions in serum testosterone concentration is a key physiological event in those affected by andropause, with men aged 70 years and older experiencing the lowest androgen levels.45 As noted, although significant endogenous reductions in testosterone can occur as early as the fifth decade,3 there is no definitive age of onset of andropause.
The andropause phenomena and the resulting hypogonadism has multiple consequences on BMD and is a primary factor in the development of osteoporosis in men.21 Hypogonadism, whether it be primary or secondary, is reported in 15 to 20% of men with spinal osteoporosis.37 Table 10–2 summarizes the common causes of secondary osteoporosis. Further, men with hypogonadism are at 6.5 times the risk of developing an osteoporotic fracture than eugonadal men.46 The loss of androgens with age is clinically significant and has multiple direct effects on bone. Testosterone has been shown to increase the activity and number of both osteoblasts and osteoclasts.47 This increased cellular activity in bone translates into increased bone turnover (i.e., an upregulation of both bone synthesis and bone breakdown), which has been shown to be essential for the maintenance of good bone health. In similar studies involving 3 months of testosterone or placebo replacement, those men receiving testosterone were found to have a reduction in markers of bone resorption32 and an increased level of osteocalcin, a marker of bone formation.48 It has been suggested that in aging men, estradiol is the dominant sex steroid regulating bone resorption, with bone formation being regulated by both estradiol and testosterone.49 Although reductions in estradiol levels have been shown to correlate with BMD in both aging women and men,50 the full impact of estradiol on bone health in men has not been realized.
Perhaps more significant with respect to bone health is that testosterone works to prevent the release of sex hormone-binding globulin (SHBG).47 Although serum total testosterone concentrations are influenced by SHBG, serum free testosterone concentrations also decrease in healthy aging men,27,28 indicating that bioavailable testosterone also decreases. Thus the amount of free testosterone available to the tissues is further decreased, ultimately affecting tissue growth and repair. In addition to binding testosterone, SHBG also binds estrogen. This may be one of the most important downstream effects of decreased testosterone on bone health. Estrogen acts on estrogen receptors in osteoblasts and increases both the activity and numbers of these bone matrix secreting cells. Although the role of estrogen on osteoclasts is controversial, it is thought to increase osteoclast cell death.47 The combination of these effects is thought to cause the well-recognized phenomenon of estrogen-delayed bone loss in postmenopausal women.
The relationship between male hypogonadism and reduced bone density has been well documented.46,50,51 However, the importance of estrogen on the male skeleton becomes apparent upon review of two particular case reports.52,53 In both instances, the men, in their mid-20s, did not experience a pubertal growth spurt, though they had normal secondary sexual characteristics. Further, both men had low BMD with several unfused epiphyses. One of the men was found to have a defective estrogen receptor, so despite high testosterone and estrogen levels, his tissue-level response was impaired.53 The other case was determined to be an aromatase deficiency resulting in low levels of estrogen,52 and treatment with estrogen led to an increase in BMD and fusion of the growth plates. These case reports support the premise that a portion of the androgen action on the male skeleton is mediated by estrogens.
Disease States and Osteoporosis in Aging Men
Although 50% of cases of osteoporosis in men appear to be idiopathic, the remaining cases are generally attributed to various diseases and treatment medications (Table 10–2).21 Chronic glucocorticoid therapy is a common cause of osteoporosis in both men and women.21 Further, some diseases of the kidney can decrease renal tubule reabsorption of calcium, also affecting BMD. In addition, loop diuretics such as furosemide also decrease the reabsorption of calcium. Furthermore, a study of men at a VA hospital found chronic obstructive pulmonary disease (COPD) and antipsychotic medications were associated with lower BMD, independent of smoking status and corticosteroid use.54 Antipsychotics, which work by decreasing dopamine, can cause an increase in prolactin, which in turn inhibits the release of estrogen and testosterone.54 Finally, a phenomenon well known to urologists is the negative effect of prostate cancer therapy on BMD.40 Following orchiectomy, men have an increased incidence of osteoporotic fractures. One study reported that 38% of men 5 years postorchiectomy had at least one osteoporotic fracture.55 These results clearly demonstrate the need for intervention strategies to prevent osteoporosis in men.
Medical Interventions | Secondary Diseases |
Medications: | Endocrine disorders: |
Glucocorticoids | Hyperparathyroidism |
Antipsychotics | Hypogonadism |
Loop diuretics (furosemide) | Pituitary tumors |
Gonadotrophin-releasing | Type 1 diabetes |
hormone agonists | Addison’s disease |
Chemotherapy (methotrexate, cyclosporine) | Cushing’s syndrome |
Anticoagulants (heparin) | Other: |
Anticonvulsants | Malnutrition |
Antacids with aluminum | Malabsorption |
Hepatic insufficiency | |
Surgeries: | Vitamin C or D deficiency |
Orchiectomy | Renal failure |
Gastrectomy | Anemia |
Alcohol |
Treatments for Osteoporosis
In patients with no clear cause of osteoporosis, many of the same treatment strategies that apply to women are also applied to men. Current recommendations include the ingestion of 1200 to 1500 mg of calcium per day, along with up to 600 IU of vitamin D.56,57 However, because some studies have shown a link between overconsumption of calcium and prostate cancer, calcium intake should be monitored in men,58 and they should consider regular evaluations by their physicians. Though more studies are needed, testosterone supplementation is not recommended at this time as supplementation appears to have only a minimal effect on BMD.59 Studies with estrogen supplementation have not been conducted in men, but in light of recent discoveries of increased risk of heart disease in estrogen-supplemented postmenopausal women, this is not a likely future direction of research.
Finally, several medications developed for osteoporotic postmenopausal women have also been used experimentally in men. One study in particular has shown that calcitonin can improve BMD in castrated men.60 However, although calcitonin is moderately effective, bisphosphonates are more widely used, have proven to be an effective treatment strategy in women with osteoporosis, and have shown positive effects on BMD and a decreased rate of fractures in men.61
Summary
Although the focus of this topic has been osteoporosis in aging men, there is some evidence that treatment strategies used for women may also prove appropriate for men. Unlike women, however, men more commonly have an underlying pathology contributing to their osteoporosis. Future studies should evaluate the extent to which the normal aging process and the resulting endocrine dysfunction contribute to alterations in bone metabolism, bone loss, and skeletal function.
Androgens and Hair
Hair is a prerequisite to be classified as a mammal, yet has no physiological function in humans.62 Through evolution and scientific advances, humans no longer requires hair for maintenance of temperature, protection, or camouflage. Although the physiological function of hair is lost, the psychological importance remains.63 Hair for males and females is a powerful social and sexual communication mechanism, through both visual and olfactory senses. A man’s beard has been compared with a lion’s mane as a visual sexual attractant for the opposite sex.62,64 Fullness of scalp, beard, and chest hair has classically been a visual correlate to a man’s masculinity. The psychological ramification of thinning hair in these areas, especially the scalp, has been the topic of much research and the target of pharmaceutical investment.
Physiology of Hair Growth
Approximately 5 million hair follicles cover the entire human body, sparing only the palms and soles. A hair follicle is defined as an invagination of the skin with continuously proliferating specialized matrix cells.65 The hair follicle is responsible for producing the keratinaceous proteins that compose a strand of hair. After birth, hair follicles can produce two different types of hair. Vellus hair is short, soft, nonpigmented, and lacks an arrector pili muscle. At birth, vellus hair covers the entire body except the palms and soles. Terminal hair is large, pigmented, and coarse, and at birth, comprises scalp hair, eyelashes, and eyebrows.62,64,65
Each hair follicle undergoes three phases of activity: a growth phase (anagen), a transition phase (catagen), and a resting phase (telogen). Anagen, which varies at different body sites and between individuals, produces hair continuously and lasts for several months to years. The average duration of this phase is 2 to 4 years.62,64–66 In normal human scalp, the total number of hairs is ∼100,000. At any given time, 90% of scalp hair follicles are in the anagen phase. Anagen determines the length of the hair at different body sites and the growth rate of anagen hairs is ∼0.35mm per day.67 Less than 1% of scalp hair follicles are in the catagen phase, a transition phase characterized by regressive changes of the hair follicle. The average duration for catagen is 2 weeks. Telogen follicles comprise ∼8 to 10% of scalp hair. Similar to anagen, the duration of telogen varies by different sites and between individuals. During the telogen phase, the hair remains secured in the base of the follicle, known as the club. The typical length of the telogen phase is approximately 2 to 3 months during which the follicle begins a new growth cycle and the hair is shed with the beginning of another anagen.68 The average ratio of anagen to telogen hairs on the scalp at any given time is 9:1, with ∼30 to 100 hairs shed on a daily basis with moderate seasonal variation.69,70
Androgen Effects on Hair
Androgens are the most potent regulator of hair growth.71,72 Although other hormones including thyroid hormone, growth hormone, and estrogen affect hair growth, androgens appear to control hair growth and distribution to the greatest extent. At the onset of puberty in males, the large increase in androgens cause vellus hair, located in the pubic and axillary regions, to transform to intermediate hair.73 These changes are graded by the Tanner scale in children and adolescents. This hair eventually becomes long, thick, pigmented terminal hair, which is found in adults. Similar changes occur on the chest, arms, legs, beard, and scalp as puberty continues and adulthood begins. These hairs and follicles cycle through the three phases described previously for the lifetime of the adult male (Fig. 10–1).
In genetically predisposed males, androgens lead to male pattern baldness or androgenetic alopecia (AGA) by inhibiting terminal hair follicles and transforming them into vellus hair.74,75 The importance of androgens on hair growth, male pattern baldness, and an inheritance component was described over 60 years ago by Hamilton.76 Prepubertal castration led to the retention of juvenile hairline, whereas castration after puberty led to normal distribution of hair seen in the general population. Despite the age of the patients or the degree of baldness at the time of castration, none of the patients had progression of hair loss over a 1-year observation period. Testosterone administration to these castrated patients, even at subphysiological doses, produced progressive hair loss and follicular miniaturization in men with a significant family history for balding. Men without a significant family history displayed no further loss of hair.
The effect of androgens on hair growth varies by body site.77–79 Hair follicles are classified into one of three categories: androgen-insensitive (growth without the influence of androgens), androgen-sensitive (anagen phase shortening and miniaturization of hair follicle leading to transformation of terminal to vellus hair upon exposure to androgens), or androgen-dependent (growth of hair requires presence of androgens). A list of body sites for each category is documented in Table 10–3. Currently, it is unknown how androgens produce these paradoxical growth effects on various sites and the genes that are involved.
Androgens derived from both the testes and the adrenal glands play a crucial role in follicle stimulation and suppression. Hair follicles not only respond to androgens but are also actively involved in androgen metabolism. Hair follicles at different body sites contain various enzymes.77 Both steroid sulfatase and 5α-reductase have been localized in the dermal papilla of scalp follicles.72,77,80 Both of these enzymes are responsible for converting weaker androgens into more potent metabolites. Hair follicles metabolize testosterone to dihydrotestosterone (DHT) through the 5α-reductase enzyme. DHT is considered more potent as it has five times the affinity toward androgen receptors. Androgen-dependent sites (e.g., beard) showed a higher 5α-reductase activity compared with androgen-insensitive body sites (e.g., occipital scalp).81
FIGURE 10-1. Different phases of hair growth. A major difference between pigmented terminal and vellus phases of hair growth is the activation of a hexose monophosphate shoot that produces NADPH, which is required by the 5α-reductase pathway to produce PHT. The pigmented terminal phase follicles produce more PHT. The effects of androgens on hair growth varies by body site. Follicles are either androgen insensitive or androgen sensitive.
A major biochemical difference between the anagen and telogen phases of hair growth is the activation of a hexose monophosphate shunt that produces reduced nicotinamide adenine dinucleotide phosphate (NADPH), which is required by the 5α-reductase pathway to produce DHT.78 This results in the anagen hair follicles producing more DHT than the resting ones. Scalp follicles have also been found to contain steroid sulfatase within their dermal papilla.82,83 Dehydroepiandrosterone sulfate (DHEAS) is converted to dehydroepiandrosterone (DHEA) via the enzyme steroid sulfatase found in scalp hair follicles. The DHEA is metabolized to androstenedione and testosterone systemically and to DHT in the hair follicles, which is another mechanism that increases androgen concentration at the hair follicle.
Androgen-insensitive Eyebrows Eyelids Occipital scalp |
Androgen-sensitive Scalp: frontal Scalp: vertex |
Androgen-dependent Pubic Axilla Chest Extremities Beard |
Androgenetic alopecia occurs in genetically predisposed men and women and is thought to be related to the concentration of potent androgens, specifically DHT, at the site of the frontal and vertex scalp follicles84 (Fig. 10–2). The dermal papilla of balding hair follicles was found to have higher concentrations of androgen receptors compared with nonbalding scalp follicles.85 Dermal papilla of hair follicles from balding (AGA) subjects were also smaller and grew less well under normal growth conditions compared with nonbalding papilla.86 In 1974, the description of patients with incomplete male pseudohermaphroditism established that DHT rather than testosterone was the androgen involved in AGA.87,88 Male patients were homozygous for mutation of the gene that encodes for steroid 5α-reductase. They were born with ambiguous genitalia and raised initially as girls. At puberty, the boys virilized, and identity was changed from female to male. These patients through puberty and adulthood developed normal male muscle mass and libido, but never experienced prostate enlargement or male pattern hair loss. These patients had markedly decreased levels of DHT with high-normal to elevated levels of testosterone. Confirmation of the importance of DHT over testosterone in the development of AGA occurred with the use of finasteride, a 5α-reductase inhibitor, in the treatment of this disorder.89–91 Finasteride was found to increase hair weight when given in low doses to patients with early AGA.92 In patients with late AGA, patients were found to decrease the amount of hair loss, with some increase in new growth.
FIGURE 10-2. Patient with androgenetic alopecia, in which androgen-sensitive terminal hair follicles are transformed to vellus hair. See Table 10–3 for effects of androgens on hair by body site.
Andropause Effects on Hair Growth
After detailing the effects that androgens have on hair follicles, it is simple to elucidate the effects of andropause on hair growth. Andropause, a syndrome of androgen deficiency with aging, leads to a decrease in all androgen production including testosterone, DHEA, and DHT.93 With the loss of these potent androgens, men experience loss of hair at androgen-dependent body sites. Men complain of decreased hair on their chest, extremities, and beard. Androgen-insensitive sites experience no change in volume or rate of growth, and androgen-sensitive sites experience decreased hair loss in genetically predisposed patients. These findings are similar to the patients described by Hamilton76 during the castration studies. Androgen replacement by injection, patch or transdermal gel reverses these symptoms and signs causing increased total body hair,94–96 but will resume the male pattern hair loss in genetically susceptible individuals.
Case Discussion
The 66-year-old man described previously is clearly having symptoms and clinical physical findings consistent with age-associated androgen deficiency. His laboratory data are consistent with an andropause state and do not appear to result from other hypogonadal etiologies. The complaints of muscle weakness, loss of beard growth, and decreased scalp hair loss in a man with the physical exam finding of male pattern baldness are also consistent with andropause. This patient, because of his symptoms, exam findings, and laboratory data, was diagnosed with androgen deficiency most likely secondary to andropause. He began testosterone replacement with Androgel 5 g applied topically daily. Within 4 weeks his testosterone level increased to midnormal ranges and his complaints of depressive symptoms, decreased energy, and erectile dysfunction resolved. After 6 months of treatment he noticed increased muscular strength and increased thickness of his beard, but complained that his scalp hair has begun to thin again. He is currently contemplating starting finasteride and is scheduled for a follow-up BMD scan in 6 months to evaluate his bone health.
REFERENCES
1. Casiano ER, Paddon-Jones D, Ostir GV, Sheffield-Moore M. Assessing functional status measures in older adults: a guide for health care professionals. Phys Ther Rev 2002;7:89–101
2. Deslypere JP, Vermeulen A. Leydig cell function in normal men: effect of age, life-style, residence, diet, and activity. J Clin Endocrinol Metab 1984;59:955–962
3. Moroz EV, Verkhratsky NS. Hypophyseal-gonadal system during male aging. Arch Gerontol Geriatr 1985;4:13–19
4. Dutta C, Hadley EC. The significance of sarcopenia in old age. J Gerontol A Biol Sci Med Sci 1995;50(Spec No):1–4
5. Mastrogiacomo I, Feghali G, Foresta C, Ruzza G. Andropause: incidence and pathogenesis. Arch Androl 1982;9:293–296
6. Simon D, Charles MA, Nahoul K, et al. Association between plasma total testosterone and cardiovascular risk factors in healthy adult men: The Telecom Study. J Clin Endocrinol Metab 1997;82:682–685
7. Short KR, Nair KS. Mechanisms of sarcopenia of aging. J Endocrinol Invest 1999;22:95–105
8. Kenney WL, Buskirk ER. Functional consequences of sarcopenia: effects on thermoregulation. J Gerontol A Biol Sci Med Sci 1995;50(Spec No):78–85
9. Kohrt WM, Holloszy JO. Loss of skeletal muscle mass with aging: effect on glucose tolerance. J Gerontol A Biol Sci Med Sci 1995;50(Spec No):68–72
10. Beaufrere B, Morio B. Fat and protein redistribution with aging: metabolic considerations. Eur J Clin Nutr 2000;54(suppl 3):S48–S53
11. Morio B, Hocquette JF, Montaurier C, et al. Muscle fatty acid oxidative capacity is a determinant of whole body fat oxidation in elderly people. Am J Physiol Endocrinol Metab 2001;280:E143–E149
12. Fiatarone MA, Marks EC, Ryan ND, Meredith CN, Lipsitz LA, Evans WJ. High-intensity strength training in nonagenarians: effects on skeletal muscle. JAMA 1990;263:3029–3034
13. Frontera WR, Meredith CN, O’Reilly KP, Evans WJ. Strength training and determinants of VO2max in older men. J Appl Physiol 1990;68:329–333
14. Frontera WR, Meredith CN, O’Reilly KP, Knuttgen HG, Evans WJ. Strength conditioning in older men: skeletal muscle hypertrophy and improved function. J Appl Physiol 1988;64:1038–1044
15. Parise G, Yarasheski KE. The utility of resistance exercise training and amino acid supplementation for reversing age-associated decrements in muscle protein mass and function. Curr Opin Clin Nutr Metab Care 2000;3:489–495
16. Hakkinen K, Pakarinen A. Serum hormones and strength development during strength training in middle-aged and elderly males and females. Acta Physiol Scand 1994;150:211–219
17. Mazzeo RS, Tanaka H. Exercise prescription for the elderly: current recommendations. Sports Med 2001;31:809–818
18. Morley JE, Kaiser FE, Sih R, Hajjar R, Perry HM III. Testosterone and frailty. Clin Geriatr Med 1997;13:685–695
19. Sih R, Morley JE, Kaiser FE, Perry HM III, Patrick P, Ross C. Testosterone replacement in older hypogonadal men: a 12-month randomized controlled trial. J Clin Endocrinol Metab 1997;82:1661–1667
20. Ferrando AA, Sheffield-Moore M, Paddon-Jones D, Wolfe RR, Urban RJ. Differential anabolic effects of testosterone and amino acid feeding in older men. J Clin Endocrinol Metab 2003;88:358–362
21. Bilezikian P. Osteoporosis in men. J Clin Endo Metab 1999; 84:3431–3434
22. Snyder PJ, Peachey H, Hannoush P, et al. Effect of testosterone treatment on body composition and muscle strength in men over 65 years of age. J Clin Endocrinol Metab 1999;84:2647–2653
23. Ferrando AA, Sheffield-Moore M, Yeckel CW, et al. Testosterone administration to older men improves muscle function: molecular and physiological mechanisms. Am J Physiol Endocrinol Metab 2002;282:E601–E607
24. Reed RL, Pearlmutter L, Yochum K, Meredith KE, Mooradian AD. The relationship between muscle mass and muscle strength in the elderly. J Am Geriatr Soc 1991;39:555–561
25. Mauras N, Hayes V, Welch S, et al. Testosterone deficiency in young men: marked alterations in whole body protein kinetics, strength, and adiposity. J Clin Endocrinol Metab 1998;83:1886–1892
26. Abbasi AA, Drinka PJ, Mattson DE, Rudman D. Low circulating levels of insulin-like growth factors and testosterone in chronically institutionalized elderly men. J Am Geriatr Soc 1993;41:975–982
27. Nankin HR, Caulkins JH. Decreased bioavailable testosterone in aging normal and impotent men. J Clin Endocrinol Metab 1986;63:1418–1420
28. Tenover JS, Matsumoto AM, Plymate SR, Bremner WJ. The effects of aging in normal men on bioavailable testosterone and luteinizing hormone secretion: response of clomiphene citrate. J Clin Endocrinol Metab 1987;65:1118–1125
29. Sheffield-Moore M. Androgens and the control of skeletal muscle protein synthesis. Ann Med 2000;32:181–186
30. Sheffield-Moore M, Urban RJ, Wolf SE, et al. Short-term oxandrolone administration stimulates net muscle protein synthesis in young men. J Clin Endocrinol Metab 1999;84:2705–2711
31. Frontera WR, Hughes VA, Fielding RA, Fiatarone MA, Evans WJ, Roubenoff R. Aging of skeletal muscle: a 12-yr longitudinal study. J Appl Physiol 2000;88:1321–1326
32. Tenover JS. Effects of testosterone supplementation in the aging male. J Clin Endocrinol Metab 1992;75:1092–1098
33. Bhasin S, Storer TW, Berman N, et al. Testosterone replacement increases fat-free mass and muscle size in hypogonadal men. J Clin Endocrinol Metab 1997;82:407–413
34. Urban RJ, Bodenburg YH, Gilkison C, et al. Testosterone administration to elderly men increases skeletal muscle strength and protein synthesis. Am J Physiol 1995;269:E820–E826
35. Sherman S. Preventing and treating osteoporosis: strategies at the millennium. Ann N Y Acad Sci 2001;949:188–197
36. Siddiqui NA, Shetty KR, Duthie EH Jr. Osteoporosis in older men—discovering when and how to treat it. Geriatrics 1999; 54:20–30
37. Boonen S, Vanderschueren D. Bone loss and osteoporotic fracture occurrence in aging men. In: Lundenfeld B, Gooren L, eds. Textbook of Men’s Health. New York: Parthenon; 2002:455–475
38. Melton LJ 3rd, Atkinson EJ, O’Connor MK, O’Fallon WM, Riggs BL. Bone density and fracture risk in men. J Bone Miner Res 1998; 13:1915–1923
39. Seeman E. The structural basis of bone fragility in men. Bone 1999;25:143–147
40. Smith M. Osteoporosis during androgen deprivation therapy for prostate cancer. Urology 2002;3(suppl 1):79–86
41. de Laet CE, van der Klift M, Hofman A, Pols HA. Osteoporosis in men and women: a story about bone mineral density thresholds and hip fracture risk. J Bone Miner Res 2002;17:2231–2236
42. Leib ES, Lenchik L, Bilezikian JP, Maricic MJ, Watts NB. Position statements of the International Society for Clinical Densitometry: methodology. J Clin Densitom 2002;5(suppl):S5–S10
43. Selby PL, Adams JE. Do men and women fracture bones at similar bone densities? Osteoporosis 2000;11:153–157
44. Binkley NC, Schmeer P, Wasnich RD, Lenchik L. What are the criteria by which a densitometric diagnosis of osteoporosis can be made in males and non-Caucasians? J Clin Densitom 2002; 5(suppl):S19–S27
45. Sheffield-Moore M, Urban RJ. Androgens and lean body mass in the aging male. In: Lundenfeld B, Gooren L, eds. Textbook of Men’s Health. New York: Parthenon; 2002:241–246
46. Stanley HL, Schmitt BP, Poses RM, Deiss WP. Does hypogonadism contribute to the occurrence of a minimal trauma hip fracture in elderly men? J Am Geriatr Soc 1991;39:766–771
47. Riggs B, Khosla S, Melton LJ 3rd. Sex steroids and the construction and conservation of the adult skeleton. Endocr Rev 2002;23:279–302
48. Morley JE, Perry HM III, Kaiser FE, et al. Effects of testosterone replacement therapy in old hypogonadal males: a preliminary study. J Am Geriatr Soc 1993;41:149–152
49. Falahati-Nini A, Riggs BL, Atkinson EJ, O’Fallon WM, Eastell R, Khosla S. Relative contributions of testosterone and estrogen in regulating bone resorption and formation in normal elderly men. J Clin Invest 2000;106:1553–1560
50. Greendale GA, Edelstein S, Barret-Connor E. Endogenous sex steroids and bone mineral density in older women and men: the Rancho Bernardo Study. J Bone Miner Res 1997;12:1833–1843
51. Murphy S, Khaw KT, Cassidy A, Compston JE. Sex hormones and bone mineral density in elderly men. Bone Miner 1993; 20:133–140
52. Morishima A, Simpson ER, Fischer C, Qin K. Aromatase deficiency in male and female siblings caused by a novel mutation and the physiological role of estrogens. J Clin Endoclinol Met 1995;80:3689–3698
53. Smith EP, Boyd J, Frank GR, et al. Estrogen resistance caused by a mutation in the estrogen receptor gene in a man. N Engl J Med 1994;331:1056–1061
54. Yeh SS, Phanumas D, Hafner A, Schuster MW. Risk factors for osteoporosis in a subgroup of elderly men in a Veterans Administration nursing home. J Invest Med 2002;50:452–457
55. Daniell HW. Osteoporosis after orchiectomy for prostate cancer. J Urol 1997;157:439–444
56. Consensus development conference: diagnosis, prophylaxis, and treatment of osteoporosis. Am J Med 1993;94:646–650
57. NIH Consensus Conference. Optimal calcium intake. NIH Consensus Development Panel on Optimal Calcium Intake. JAMA 1994;272:1942–1948
58. Giovannucci E, Rimm EB, Wolk A, et al. Calcium and fructose intake in relation to risk of prostate cancer. Cancer Res 1998; 58:442–447
59. Shetty KR, Maas DL. Testosterone replacement for hypogonadal elderly men. Clin Geriatr 1998;6:60–68
60. Stepan JJ, Lachman M, Zverina J, et al. Castrated men exhibit bone loss: effect of calcitonin treatment on biochemical indices of bone remodeling. J Clin Endocrinol Metab 1989;69:523–527
61. Orwall E, Weiss S, Miller P, et al. Alendronate for the treatment of osteoporosis in men. N Engl J Med 2000;343:604–610
62. Ebling FJ. Hair. J Invest Dermatol 1976;67:98–105
63. Sternbach H. Age-associated testosterone decline in men: clinical issues for psychiatry. Am J Psychiatry 1998;155:1310–1318
64. Ebling FJ. The biology of hair. Dermatol Clin 1987;5:467–481
65. Mercurio MG, Gogstetter DS. Androgen physiology and the cutaneous pilosebaceous unit. J Gend Specif Med 2000;3:59–64
66. Tosi A, Misciali C, Piraccini BM, Peluso AM, Bardazzi F. Drug-induced hair loss and hair growth: incidence, management and avoidance. Drug Saf 1994;10:310–317
67. Myers R, Hamilton J. Regeneration and rate of growth of hair in man. Ann N Y Acad Sci 1951;53:562–568
68. Orentreich N, Durr NP. Biology of scalp hair growth. Clin Plast Surg 1982;9:197–205
69. Courtois M, Loussouarn G, Hourseau S, Grollier JF. Periodicity in the growth and shedding of hair. Br J Dermatol 1996; 134:47–54
70. Randall VA, Ebling FJ. Seasonal changes in human hair growth. Br J Dermatol 1991;124:146–151
71. Ebling FJ. Hair follicles and associated glands as androgen targets. Clin Endocrinol Metab 1986;15:319–339
72. Randall VA, Thornton MJ, Hamada K, et al. Androgens and the hair follicle. Cultured human dermal papilla cells as a model system. Ann N Y Acad Sci 1991;642:355–375
73. Hiort O, Holterhus PM, Nitsche EM. Physiology and pathophysiology of androgen action. Baillieres Clin Endocrinol Metab 1998;12:115–132
74. Sawaya ME. Biochemical mechanisms regulating human hair growth. Skin Pharmacol 1994;7:5–7
75. Sawaya ME. Clinical updates in hair. Dermatol Clin 1997;15: 37–43
76. Hamilton JB. Male hormone stimulation is prerequisite and an incitement in common baldness. Am J Anat 1942;71:451
77. Hoffmann R. Enzymology of the hair follicle. Eur J Dermatol 2001;11:296–300
78. Mooradian AD, Morley JE, Korenman SG. Biological actions of androgens. Endocr Rev 1987;8:1–28
79. Randall VA, Hibbetrs NA, Thornton MJ, et al. The hair follicle: a paradoxical androgen target organ. Horm Res 2000;54:243–250
80. Drake L, Hordinsky M, Fiedler V, et al. The effects of finasteride on scalp skin and serum androgen levels in men with androgenetic alopecia. J Am Acad Dermatol 1999;41:550–554
81. Itami S, Kurata S, Sonoda T, Takayasu S. Mechanism of action of androgen in dermal papilla cells. Ann N Y Acad Sci 1991; 642:385–395
82. Hoffmann R, Rot A, Niiyama S, Billich A. Steroid sulfatase in the human hair follicle concentrates in the dermal papilla. J Invest Dermatol 2001;117:1342–1348
83. Sawaya ME. Steroid chemistry and hormone controls during the hair follicle cycle. Ann N Y Acad Sci 1991;642:376–383; discussion 383–374.
84. Hoffmann R, Happle R. Current understanding of androgenetic alopecia. Part I: etiopathogenesis. Eur J Dermatol 2000; 10:319–327
85. Hibberts NA, Howell AE, Randall VA. Balding hair follicle dermal papilla cells contain higher levels of androgen receptors than those from non-balding scalp. J Endocrinol 1998; 156:59–65
86. Randall VA, Hibberts NA, Hamada K. A comparison of the culture and growth of dermal papilla cells from hair follicles from non-balding and balding (androgenetic alopecia) scalp. Br J Dermatol 1996;134:437–444
87. Imperato-McGinley J, Guerrero L, Gautier T, Peterson RE. Steroid 5alpha-reductase deficiency in man: an inherited form of male pseudohermaphroditism. Science 1974;186: 1213–1215
88. Walsh PC, Madden JD, Harrod MJ, Goldstein JL, MacDonald PC, Wilson JD. Familial incomplete male pseudohermaphroditism, type 2. Decreased dihydrotestosterone formation in pseudo-vaginal perineoscrotal hypospadias. N Engl J Med 1974; 291:944–949
89. Hoffmann R, Happle R. Current understanding of androgenetic alopecia. Part II: clinical aspects and treatment. Eur J Dermatol 2000;10:410–417
90. Whiting DA. Advances in the treatment of male androgenetic alopecia: a brief review of finasteride studies. Eur J Dermatol 2001;11:332–334
91. Wolff H, Kunte C. Current management of androgenetic alopecia in men. Eur J Dermatol 1999;9:606–609
92. Price VH, Menefee E, Sanchez M, Ruane P, Kaufman KD. Changes in hair weight and hair count in men with androgenetic alopecia after treatment with finasteride, 1 mg, daily. J Am Acad Dermatol 2002;46:517–523
93. Heaton JP. Andropause: coming of age for an old concept? Curr Opin Urol 2001;11:597–601
94. Bhasin S, Bremner WJ. Clinical review 85: emerging issues in androgen replacement therapy. J Clin Endocrinol Metab 1997; 82:3–8
95. Rolf C, Nieschlag E. Potential adverse effects of long-term testosterone therapy. Baillieres Clin Endocrinol Metab 1998; 12:521–534
96. Winters SJ. Current status of testosterone replacement therapy in men. Arch Fam Med 1999;8:257–26
< div class='tao-gold-member'>
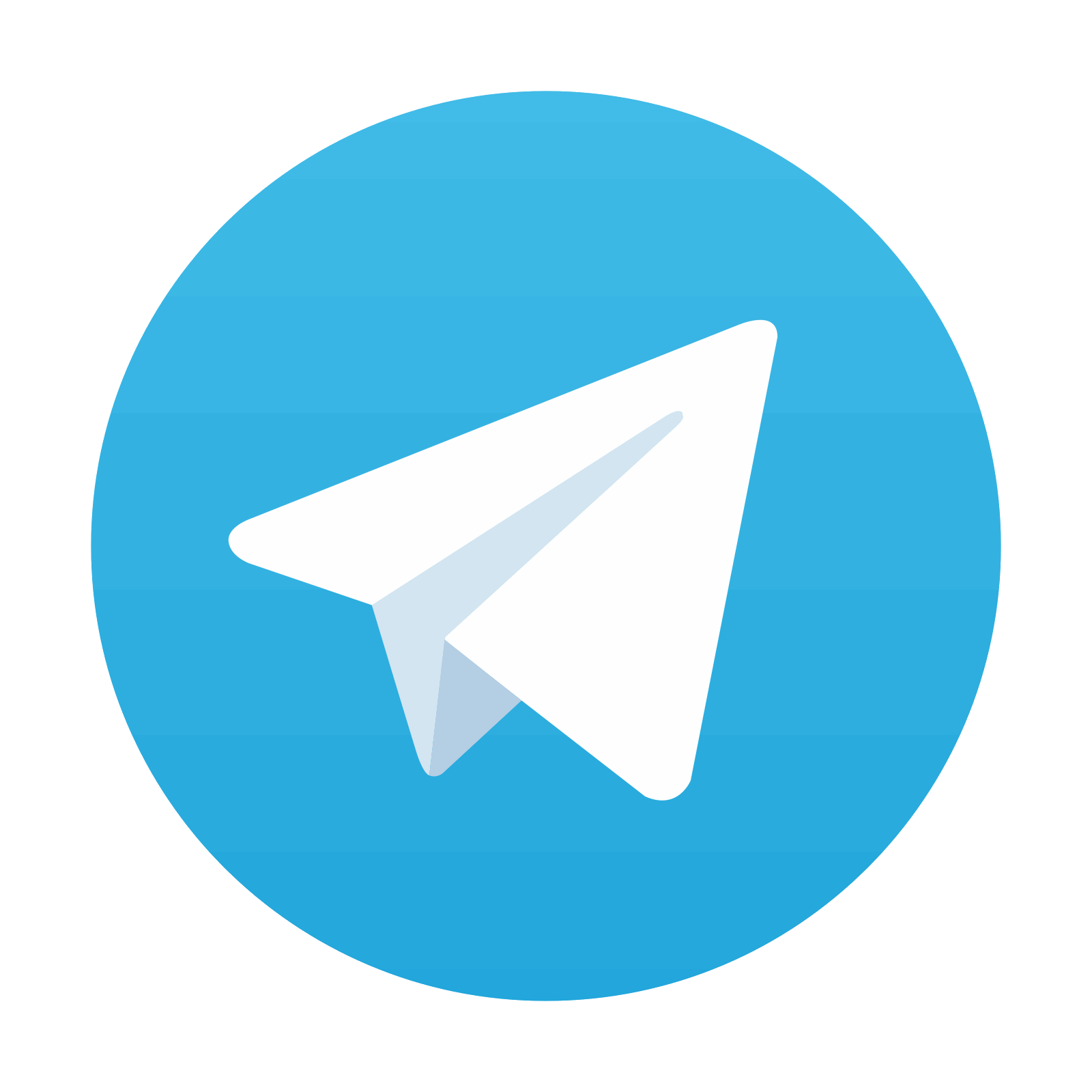