Lee Richstone, MD, Douglas S. Scherr, MD No sooner were laparoscopic techniques established that the field witnessed the rise of a new minimally invasive technique: robotic-assisted laparoscopic surgery. Robotic surgical approaches have been employed for nearly all types of bladder surgery, becoming an accepted alternative for the operative management of lower tract pathology of benign and malignant etiology. Technology continues to drive the field forward, and natural orifice transendoluminal surgery (NOTES) and laparoendoscopic single-site (LESS) surgical techniques have been employed to further reduce the incisions and morbidity associated with surgery of the urinary bladder. In this chapter we describe conventional laparoscopic, LESS, and robotic approaches to surgery of the distal ureter and the bladder, focusing on indications, technique, complications, and outcomes (Table 84–1). Table 84–1 Minimally Invasive Surgery of the Bladder and Distal
Bladder Diverticulectomy
Diverticula of the urinary bladder represent a herniation of the urinary mucosa through a weakness of absence of the detrusor muscle. Bladder diverticula have scattered and/or nonfunctional residual muscle fibers, impairing emptying and contributing to urinary stasis. Diverticula can be congenital, acquired, or, rarely, iatrogenic in nature. Congenital bladder diverticula include periureteral diverticula, also referred to as Hutch diverticula, which can be associated with vesicoureteral reflux (VUR). Posterior urethral valves and neurogenic bladder are also commonly associated with diverticula. Various syndromes including Williams, Menkes, and prune belly can include multiple diverticula in the absence of outlet obstruction. Acquired diverticula are most commonly associated with bladder outlet obstruction (BOO), with resultant high-pressure voiding and subsequent diverticula formation. Traditionally, diverticulectomy has been approached through a low midline incision. However, with the evolution of minimally invasive technology and skill, even complex and multiple bladder diverticula can be managed with minimally invasive surgical techniques (Fig. 84–1). In combination with endoscopic management of bladder outlet obstruction and bladder calculi, these techniques often result in reduced morbidity to the patient.
Surgical Approach
Since Czerny’s first description of diverticulectomy in 1897 (Knappenberger et al, 1960), the surgical treatment of bladder diverticula has evolved from open surgery, to endoscopic procedures, to laparoscopic and robotic techniques. Transperitoneal laparoscopic bladder diverticulectomy (LBD) was first introduced in 1992 (Das et al, 1992; Parra et al, 1992) and later in the pediatric setting (Kok et al, 2000). Numerous subsequent reports demonstrate the reproducibility of the technique via transvesical and extravesical approaches (Nadler et al, 1995). Extraperitoneal laparoscopy may limit the risk of visceral injury and intra-abdominal urine leak. The transperitoneal approach provides a wide and generous working space, as well as superior access for posterior diverticula. Laparoscopic bladder diverticulectomy has also been described for tumor within a diverticulum (Tai et al, 2007; Wang et al, 2007; Thwaini et al, 2008) and is described in more detail in a subsequent section on laparoscopic and robotic partial cystectomy.
Similarly, robotic bladder diverticulectomy (RBD) has been described in adults and children as an effective minimally invasive alternative providing similar benefits (Myer et al, 2007; Rao et al, 2007; Macejko et al, 2008; Tareen et al, 2008; Meeks et al, 2009). The robotic approach mimics the laparoscopic technique, while aiding the surgeon without extensive laparoscopic experience, especially for complex diverticula and the need for concomitant ureteral reimplantation. Neither approach is likely to be superior to one another in efficacy. Choosing a surgical approach to bladder diverticulectomy is dependent on numerous factors including the number and location of diverticula, proximity of the diverticulum to the ureter(s), and the need for concomitant ureteral or bladder outlet surgery. Even complex and multiple diverticula requiring concomitant ureteral reimplantation can be handled via a laparoscopic or robotic approach provided sufficient surgeon experience and skill.
Technique
Before port placement, flexible cystoscopy can be performed for ureteral catheterization if necessary. Selective catheterization of the diverticulum can be performed by placing a Councill tip catheter over a guidewire into the diverticulum and a separate Foley catheter into the bladder proper, for selective filling and intraoperative identification of bladder diverticula (Nadler et al, 1995; Porpiglia et al, 2002; Khonsari et al, 2004), as seen in Figure 84–2.
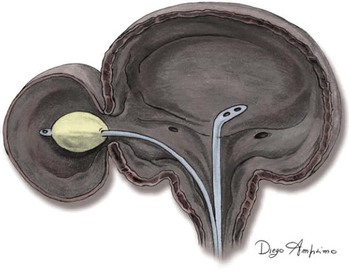
(From Porpiglia F, Tarabuzzi R, Cossu M, et al: Sequential transurethral resection of the prostate and laparoscopic bladder diverticulectomy: comparison with open surgery. Urology 2002;60:1045.)
Alternatively, real-time illumination of the diverticulum with the flexible cystoscope is a useful aid in identifying the diverticulum (Parra et al, 1992; Jarrett et al, 1995; Nadler et al, 1995). In the authors’ experience, this is particularly valuable in patients with multiple diverticula, in which separate catheterization of each diverticulum is not practical (Cinman et al, in press). After pneumoperitoneum is established by closed or open technique, trocars for robotic and laparoscopic bladder diverticulectomy are positioned as depicted in Figure 84–3.
If approached transperitoneally, LBD or RBD begins with incision of the peritoneum overlying the diverticulum. For complex, large, or multiple diverticula, the bladder can be mobilized: The peritoneum is incised medial to the obliterated umbilical ligament bilaterally, the urachus is divided, and the bladder is “dropped” posteriorly, allowing for entry into the space of Retzius. The 10-mm LigaSure (Valleylab, Boulder, CO) can be used during LBD, and a combination of monopolar and bipolar cautery instrumentation is employed during RBD. With the aid of either selective catheterization and filling or cystoscopic transillumination, the diverticulum is identified. Peridiverticular adhesions are transected, and the mouth of the diverticulum is then circumscribed and excised. Constant vigilance is required to prevent injury to the ureter or ureteral orifice. If necessary, a transvesical approach can be employed. The bladder is opened and the diverticulum pulled into the bladder, circumscribed, and excised. In either approach, the bladder is then closed anatomically in two layers with 2-0 polyglactin suture. During LBD, free-hand intracorporeal suturing can be employed. Alternatively, an Endo Stitch device (Covidien, Mansfield, MA) can be used as a suturing aid. An Endo GIA (Covidien, Mansfield, MA) stapling device can also be used for excision, yet this carries with it the theoretical risk of bladder stone formation (Kerbl et al, 1993; Wang et al, 2007). The bladder is then filled to ensure water-tight closure. A Jackson-Pratt drain is placed via one of the trocar sites to avoid an unnecessary additional incision. If needed, ureteral reimplantation secondary to reflux, obstruction, or iatrogenic injury is performed as described in the following section.
Surgical management for bladder outlet obstruction can be performed concomitantly, either immediately before or after bladder diverticulectomy (BD). This includes TURP, KTP laser vaporization, or holmium laser enucleation (Iselin et al, 1996; Grønlund et al, 1998; Porpiglia et al, 2002; Faramarzi-Roques et al, 2004; Shah et al, 2006; Cinman et al, in press). If performed after diverticulectomy, a suprapubic catheter should be placed to avoid perforation at the bladder-closure suture lines. Similarly, laparoscopic or robotic simple prostatectomy can be performed at the time of LBD or RBD (Magera et al, 2008).
Outcomes and Complications
Minimally invasive techniques have been demonstrated to be effective in treating BD. For example, in 2009 Cinman and colleagues (in press) demonstrated that LBD was effective in reducing PVR (preoperative mean 475 mL) to normal values less than 50 mL (Fig. 84–4). LBD has been demonstrated effective even for patients with complex pathology including giant diverticula up to 30 cm, multiple diverticula (Khonsari et al, 2004; Cinman et al, in press), and those needing concomitant ureteral reimplantation or laparoscopic pyeloplasty (Cinman et al, in press).
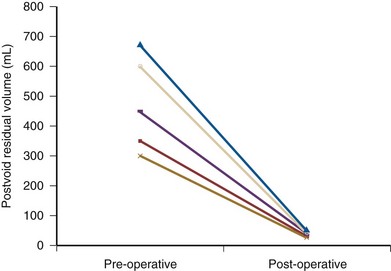
(Richstone and Kavoussi, unpublished data.)
Minimally invasive management of a bladder diverticulum and BOO with concomitant LBD and TURP has been demonstrated to be superior when compared with open BD and transvesical prostatectomy. LBD and TURP is associated with reduced blood loss, analgesic requirements, and shorter hospital stay but longer operating room times (Porpiglia et al, 2002; Porpiglia et al, 2004). No complications were noted with either approach, and postoperative urinary flow rates were equivalent. In a series of 13 patients undergoing extravesical LBD without concurrent management of BOO, Abdel-Hakim and colleagues (2007) experienced only one complication in the form of extravasation from the suture line that resolved with conservative management.
Nonrefluxing Ureteral Reimplantation
Several investigators demonstrated the feasibility of laparoscopic correction of VUR in animal studies (Atala et al, 1993; Schimberg et al, 1994; McDougall et al, 1995). Laparoscopic extravesical Lich-Gregoir ureteral reimplantation in humans followed shortly thereafter (Ehrlich et al, 1994; Reddy et al, 1994; Janetschek et al, 1995). Typical port-site placement is similar to that employed for bladder diverticulectomy (see Fig. 84–3).
The extravesical Lich-Gregoir technique can be performed via a conventional laparoscopic or robotic-assisted approach. The ureter is identified medial to the obliterated umbilical ligament, and the overlying peritoneum is incised. The ureter is mobilized to the ureterovesical junction, preserving periureteric tissue to ensure adequate vascular supply. The location of the proposed tunnel is then determined with the bladder filled with saline, and care is taken to avoid creating a “kinking” tunnel. A 5 : 1 tunnel-to-ureteral diameter is maintained. Taking care to avoid perforation of the bladder mucosa, the detrusor muscle is incised to create a sufficient trough. The ureter is placed within the trough and the detrusor closed over it, using a series of interrupted 3-0 absorbable sutures (Fig. 84–5) (Reddy et al, 1994; Lakshmanan et al, 2000).
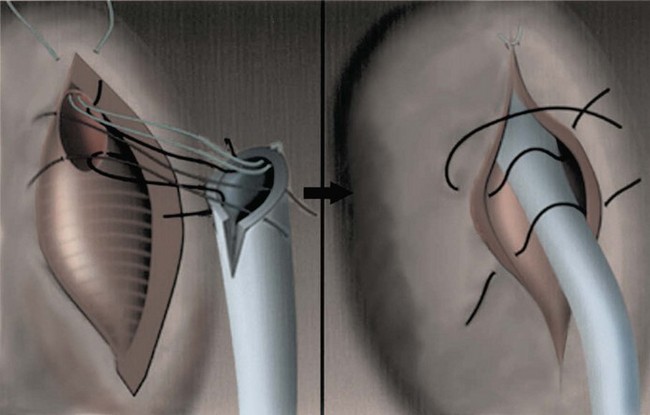
(From Andou M, Yoshioka T, Ikuma K. Laparoscopic ureteroneocystostomy. Obstet Gynecol 2003;102:1183.)
The transvesical Cohen cross-trigonal approach has been employed by several authors, by both the pure laparoscopic and robotic technique (Gill et al, 2001; Peters et al, 2005; Yeung et al, 2005; Kutikov et al, 2006). By avoiding the peritoneal cavity, this transvesical approach has the potential to limit complications associated with the transabdominal approach. A three-trocar configuration accommodates either the pure laparoscopic or robotic technique.
As originally described by Gill and colleagues, the procedure is performed with two 5-mm transvesical trocars, glycine irrigation within the bladder, and visualization throughout the procedure with a transurethral 24-Fr resectoscope (Gill et al, 2001). An electrosurgical Collins knife is used to incise the bladder mucosa and create the cross-trigonal trough. Then the detrusor is sewn over the ureter laparoscopically. This procedure was modified by Yeung and colleagues using carbon dioxide insufflation of the bladder and the use of a midline trocar for laparoscopic, rather than cystoscopic visualization of the procedure. In pediatric patients, two 3-mm trocars can be used for working ports, a 5-mm midline trocar can be used for a camera, and an 8-Fr pediatric feeding tube can be placed via urethra for intermittent suctioning (Kutikov et al, 2006). Similarly, a three-trocar robotic transvesical approach to cross-trigonal reimplantation has been described (Peters et al, 2005). After circumferential incision around the ureteral orifice, the ureter is mobilized by incising periureteral attachments. The native hiatus is reduced with 4-0 absorbable suture, and a cross-trigonal submucosal tunnel and new mucosa hiatus are created. The ureter is advanced and anchored with several interrupted 4-0 absorbable sutures, and the periureteral mucosa is sutured to the bladder mucosa with 5-0 absorbable stitches (Fig. 84–6).
Refluxing Ureteral Reimplantation
Laparoscopic or robotic ureteral reimplantation for benign ureteral stricture disease or select cases of ureteral malignancy is commonly performed in a refluxing manner (Seideman et al, 2009). The authors routinely perform laparoscopic ureteral reimplantation using a three-trocar approach (Fig. 84–7).
The distal ureter is dissected, and the diseased segment is excised. In cases of transitional cell carcinoma, a flexible cystoscope can be placed in the bladder to ensure adequate bladder cuff resection and protection of the contralateral ureteral orifice. The ureteral hiatus is closed in two layers with absorbable sutures. A new ureteral hiatus is created at the dome of the bladder, and the spatulated ureter is reimplanted with 4-0 absorbable sutures in a running or interrupted fashion (Reddy et al, 1994; Fugita et al, 2001). Minimally invasive management of longer ureteral defects is discussed in the next section on psoas hitch and bladder advancement flaps.
Outcomes and Complications
Lakshmanan and colleagues (2000) describe 71 extravesical ureteral reimplantations in 47 patients for management of VUR. No patients experienced subsequent obstruction or recurrent reflux. Complications were minimal and included three ureteral injuries, two of which required open reimplantation. Yeung and colleagues (2005) performed 30 laparoscopic transvesical cross-trigonal reimplantations in 16 patients. The mean operative team was 112 minutes for unilateral and 178 minutes for bilateral cases. Two patients had subcutaneous and scrotal emphysema that resolved spontaneously. The radiographic success rate was 96%.
In the largest reported series in the adult population, Seideman and colleagues (2009) reported on 45 patients undergoing laparoscopic ureteral reimplantation for benign and malignant pathology. The median hospital stay was 3 days and estimated blood loss (EBL) of 150 mL. Minimal complications were experienced including three patients with urinary extravasation at the anastomotic site, conservatively managed in all cases. With a mean follow-up of 2 years, the authors reported a 96% success rate.
Rassweiler (2007) compared 10 patients undergoing laparoscopic ureteral reimplantation to 10 patients treated by open techniques. In this small series, the laparoscopic approach was associated with less blood loss, analgesic requirements, time to oral intake, hospital stay, and convalescence time. Similarly, Simmons and colleagues (2007) retrospectively compared 12 laparoscopic versus 34 open ureteral reimplantation procedures, demonstrating a reduced blood loss and shorter hospitalization associated with laparoscopy. Complication rates and ureteral patency rates were equivalent at a mean follow-up of nearly 2 years. Several authors have documented the feasibility of robotic-assisted ureteral reimplantation (Yohannes et al, 2003; Uberoi et al, 2007; Patil et al, 2008). The robotic technique recapitulates the laparoscopic technique and may facilitate the learning curve for reconstructive procedures requiring intracorporeal suturing.
More recently, LESS surgery has emerged as a technique with the potential for improved cosmetic outcomes and perhaps reduced pain and convalescence. LESS ureteroneocystostomy has been reported, but larger series are necessary to validate the efficacy and safety of the approach (Desai et al, 2009).
Key Points: Ureteral Reimplantation
Psoas Hitch and Bladder Advancement Flaps
When faced with minimal distal ureteral loss, simple ureteroneocystostomy is sufficient to re-establish urinary continuity. However, more extensive gaps require more complex reconstructive techniques. The Boari bladder flap was introduced for bridging larger gaps between the ureter and bladder in 1894 in the canine model and in humans in 1947 (Fugita et al, 2001). Laparoscopic Boari flap was first performed in the porcine model and in humans in 2001 (Fergany et al, 2001; Fugita et al, 2001). This can be performed with or without a psoas hitch, to anchor the bladder in order to gain additional length and avoid anastomotic tension. Defects of the distal third ureter are routinely bridged with this technique, and in select cases the mid or proximal ureter can be reached. When this approach is not sufficient, transureteroureterostomy, autotransplantation, or ileal ureteral substitution can be considered.
Technique
Laparoscopic or Robotic Boari Flap
Laparoscopic Boari flap was first described in humans in by Fugita and colleagues (2001) in a series of three patients with distal ureteral obstruction. The robotic-assisted laparoscopic approach was first reported several years later (Schimpf et al, 2008). Trocar placement for robotic and laparoscopic reimplantation and Boari flap is depicted in Figures 84-3 and 84-7, respectively. The procedure begins by incising the ipsilateral white line of Toldt and the ureter identified as it crosses the iliac vessels. The ureter is mobilized distally, and the diseased segment excised, ensuring that the distal margin is well vascularized and healthy. If indicated, a distal margin is sent for frozen section, and the ureter is spatulated. The bladder is filled with 200 mL of normal saline and mobilized by incising the peritoneum medial to the obliterated umbilical ligaments bilaterally and transecting the urachus. Blunt dissection allows the bladder to “drop” posteriorly, and the space of Retzius is entered. Ligation of the contralateral bladder pedicle with an Endo GIA stapling device (Covidien, Mansfield, MA) and psoas hitch using 2-0 absorbable suture can often provide enough mobilization to perform a ureteroneocystostomy (Modi et al, 2005; Schimpf et al, 2008). If this maneuver does not suffice, a Boari flap or bladder advancement flap is performed. Using electrosurgical scissors or a 10-mm LigaSure (Valleylab, Boulder, CO) device, an anterior bladder flap is created beginning approximately 2 cm from the bladder neck, extending to the ipsilateral bladder dome; the apex and base of the flap are approximately 2 cm and 4 cm, respectively (Fugita et al, 2001) (Fig. 84–8). Care should be taken to ensure that the base of the flap is wide enough to ensure adequate vascularity. The spatulated ureter is anastomosed to the apex of the flap with interrupted 4-0 absorbable suture. After placement of a 7-Fr double pigtail catheter over a guidewire, the flap is then closed in a running fashion in two layers with 4-0 and 2-0 absorbable suture or with the assistance of an EndoStitch device (Covidien, Mansfield, MA). The bladder is filled to 300 mL to identify any sites of anastomotic leakage, and a Jackson-Pratt drain is placed through the 5-mm trocar site. The Jackson-Pratt drain is typically removed in 48 hours, and the Foley catheter is removed in 1 week following a cystogram to confirm no urinary leakage. The ureteral stent is removed in 4 weeks.
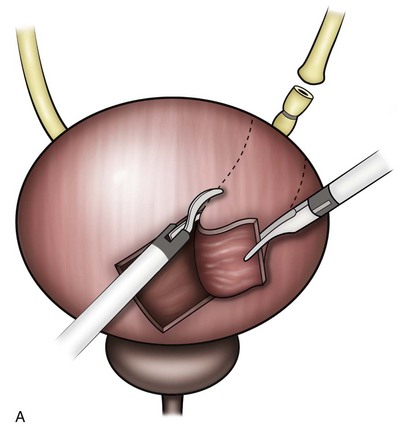
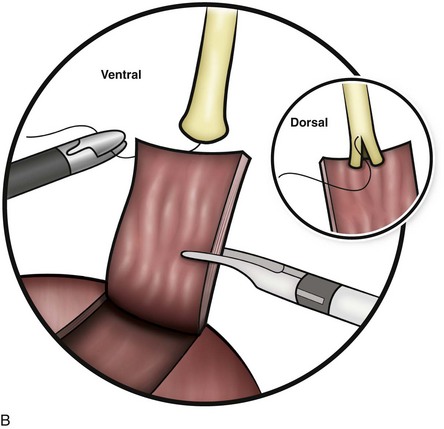
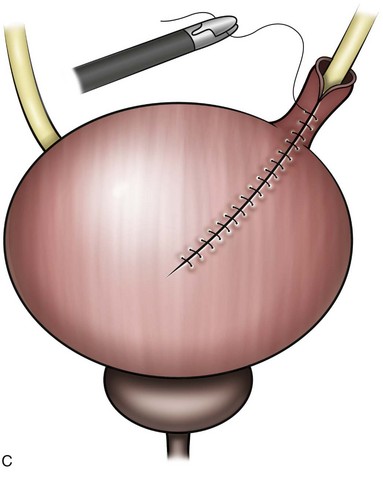
(Modified from Fugita OE, Kavoussi L. Laparoscopic ureteral reimplantation for ureteral lesion secondary to transvaginal ultrasonography for oocyte retrieval. Urology 2001;58:281.)
Laparoscopic Bladder Advancement Flap
Laparoscopic bladder advancement flap was first described by Lima and colleagues (2005) as a simplified alternative to a Boari flap. The bladder is opened with a transverse incision, placed one third of the distance from the dome to the bladder neck (Fig. 84–9). The spatulated ureter is anastomosed to the bladder flap in a fashion similar to a Boari flap, described previously.
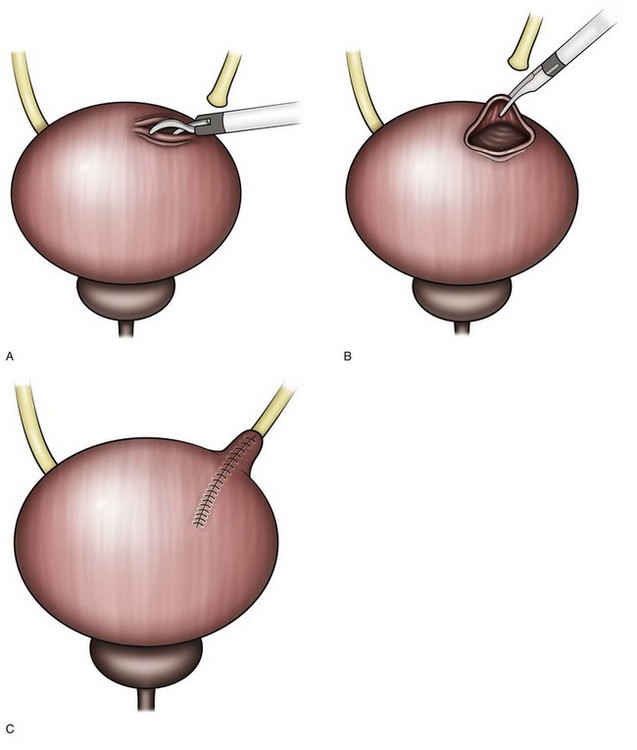
(Adapted from Lima GC, Rais-Bahrami S, Link RE, Kavoussi LR. Laparoscopic ureteral reimplantation: a simplified dome advancement technique. Urology 2005;66:1307.)
Outcomes and Complications
The first clinical series of laparoscopic ureteral reimplantation with Boari flap was reported in 2001, involving three patients (Fugita et al, 2001). The approach was demonstrated to be feasible with a mean operative time of 220 minutes and EBL ranging from 400 to 600 mL. With a mean follow-up of 11 months, all patients had resolution of obstruction. Castillo and colleagues (2005) reported a slightly larger series of laparoscopic Boari flap in eight patients. Operative times (mean 157 minutes), blood loss (mean 124 mL), and hospital stay (mean 3 days) further validated the feasibility of the technique. Two complications occurred including one pulmonary embolism and one patient with urinary leakage at the anastomosis requiring laparoscopic repair. With a mean follow-up of 18 months, all patients had resolution of obstruction. Seideman and colleagues (2009) reported laparoscopic Boari flap in 21 out of 45 patients undergoing laparoscopic ureteral reimplantation, representing the largest published series to date, with the longest reported follow-up (mean 24 months). Two patients underwent distal ureterectomy for transitional cell carcinoma. Median length of stay and EBL was 3 days and 150 mL, respectively. Complications included one patient with small bowel obstruction, one patient with Clostridium difficile colitis and respiratory distress, and two patients with urinary leakage that was managed conservatively in both cases.
Enterocystoplasty
Augmentation cystoplasty is a well-established surgical option for the patient with a small capacity, poorly compliant bladder who has failed conservative management. Docimo and colleagues (1995) were the first to perform a purely laparoscopic gastrocystoplasty in a single patient. Although the procedure took almost 11 hours and involved a 13-day hospital course, the feasibility of the technique was demonstrated. Subsequently, Gill and colleagues (2000)
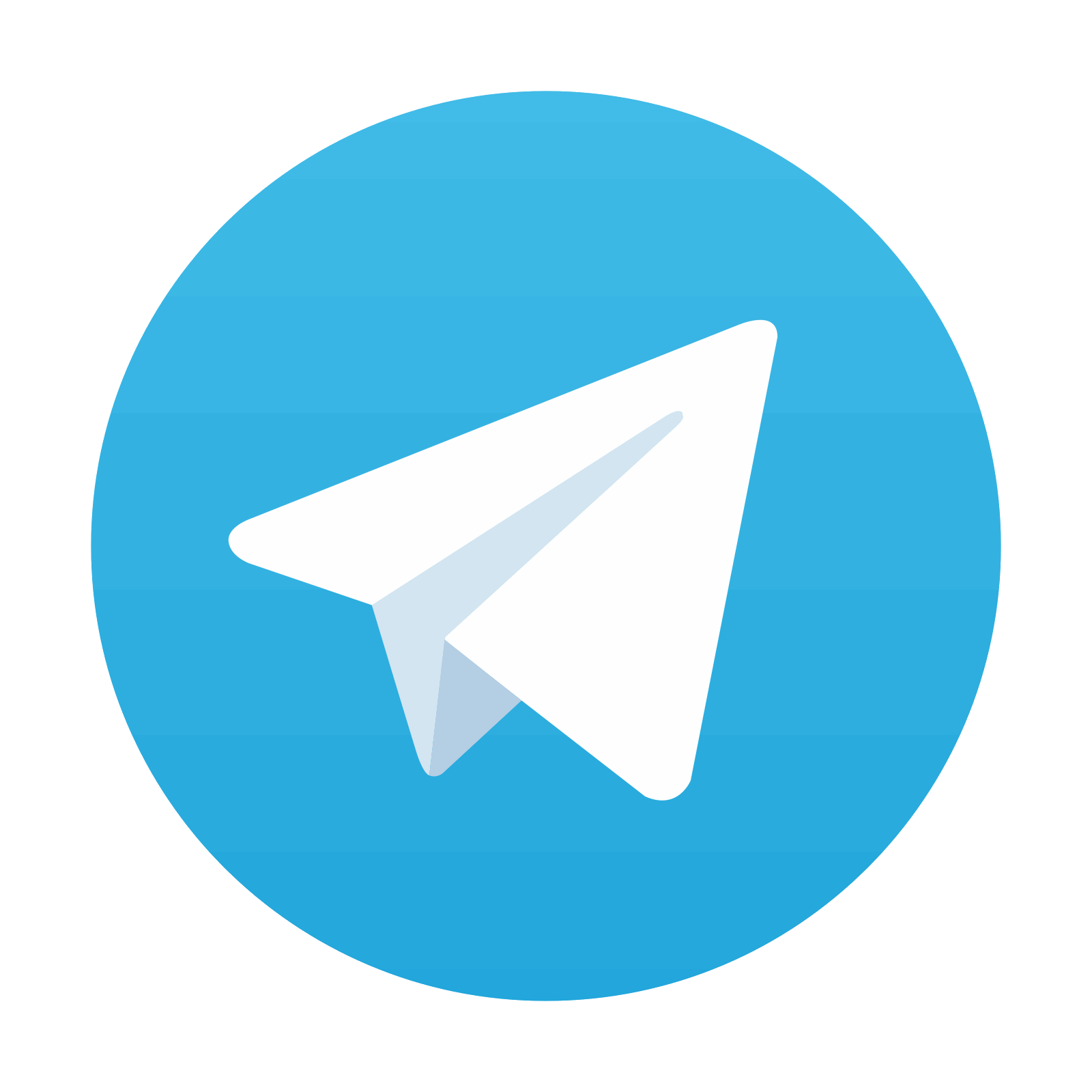
Stay updated, free articles. Join our Telegram channel

Full access? Get Clinical Tree
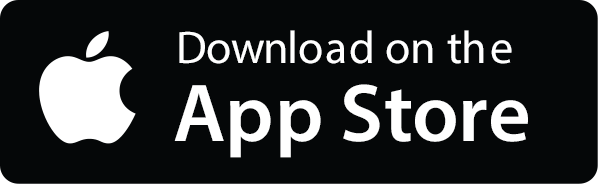
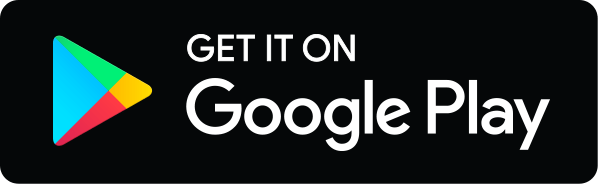