Wilhelm Kriz, Marlies Elger
Renal Anatomy
The complex structure of the mammalian kidney is best understood in the unipapillary form that is common to all small species. Figure 1-1 is a schematic coronal section through a unipapillary kidney, with a cortex enclosing a pyramid-shaped medulla, the tip of which protrudes into the renal pelvis. The medulla is divided into an outer and an inner medulla; the outer medulla is further subdivided into an outer and an inner stripe.
Structure of the Kidney
The specific components of the kidney are the nephrons, the collecting ducts, and a unique microvasculature.1 The multipapillary kidney of humans contains about 1 million nephrons, although this number varies considerably. The number of nephrons is already established during prenatal development; after birth, new nephrons cannot be developed, and a lost nephron cannot be replaced.
Nephrons
A nephron consists of a renal corpuscle (glomerulus) connected to a complicated and twisted tubule that finally drains into a collecting duct (Fig. 1-2 and Table 1-1). Three types of nephron can be distinguished by the location of renal corpuscles within the cortex: superficial, midcortical, and juxtamedullary nephrons. The tubular part of the nephron consists of a proximal tubule and a distal tubule connected by a loop of Henle2 (see later discussion). There are two types of nephron, those with long loops of Henle and those with short loops. Short loops turn back in the outer medulla or even in the cortex (cortical loops). Long loops turn back at successive levels of the inner medulla.
Table 1-1
Subdivisions of the nephron and collecting duct system.
Subdivisions of the Nephron and Collecting Duct System | |
Section | Subsections |
Nephron | |
Renal corpuscle | Glomerulus: term used most frequently to refer to entire renal corpuscle Bowman capsule |
Proximal tubule | Convoluted part Straight part (pars recta), or thick descending limb of Henle loop |
Intermediate tubule | Descending part, or thin descending limb of Henle loop Ascending part, or thin ascending limb of Henle loop |
Distal tubule | Straight part, or thick ascending limb of Henle loop: subdivided into medullary and cortical parts; the cortical part contains the macula densa in its terminal portion Convoluted part |
Collecting Duct System | |
Connecting tubule | Includes the arcades in most species |
Collecting duct | Cortical collecting duct Outer medullary collecting duct: subdivided into an outer stripe and an inner stripe portion Inner medullary collecting duct: subdivided into basal, middle, and papillary portions |
Collecting Ducts
A collecting duct is formed in the renal cortex when several nephrons join. A connecting tubule is interposed between a nephron and a cortical collecting duct. Cortical collecting ducts descend within the medullary rays of the cortex. These ducts traverse the outer medulla as unbranched tubes. On entering the inner medulla, the cortical collecting ducts fuse successively and open finally as papillary ducts into the renal pelvis (see Fig. 1-2 and Table 1-1).
Microvasculature
The microvascular pattern of the kidney is similarly organized in mammalian species1,3 (Fig. 1-3; see also Fig. 1-1). The renal artery, after entering the renal sinus, finally divides into the interlobar arteries, which extend toward the cortex in the space between the wall of the pelvis (or calyx) and the adjacent cortical tissue. At the junction between cortex and medulla, the interlobar arteries divide and pass over into the arcuate arteries, which also branch. The arcuate arteries give rise to the cortical radial arteries (interlobular arteries), which ascend radially through the cortex. No arteries penetrate the medulla.
Afferent arterioles supply the glomerular tufts and generally arise from cortical radial arteries. Aglomerular tributaries to the capillary plexus are rarely found. As a result, the blood supply of the peritubular capillaries of the cortex and the medulla is exclusively postglomerular.
Glomeruli are drained by efferent arterioles. Two basic types of efferent arterioles can be distinguished, cortical and juxtamedullary. Cortical efferent arterioles, which derive from superficial and midcortical glomeruli, supply the capillary plexus of the cortex. The efferent arterioles of juxtamedullary glomeruli represent the supplying vessels of the renal medulla. Within the outer stripe of the medulla, these vessels divide into the descending vasa recta and then penetrate the inner stripe in cone-shaped vascular bundles. At intervals, individual vessels leave the bundles to supply the capillary plexus at the adjacent medullary level.
Ascending vasa recta drain the renal medulla. In the inner medulla, the vasa recta arise at every level, ascending as unbranched vessels, and traverse the inner stripe within the vascular bundles. The ascending vasa recta that drain the inner stripe may join the vascular bundles or may ascend directly to the outer stripe between the bundles. All the ascending vasa recta traverse the outer stripe as individual wavy vessels with wide lumina interspersed among the tubules. Because true capillaries derived from direct branches of efferent arterioles are relatively scarce, the ascending vasa recta form the capillary plexus of the outer stripe. The ascending vasa recta empty into arcuate veins.
The vascular bundles represent a countercurrent exchanger between the blood entering and that leaving the medulla. In addition, the organization of the vascular bundles results in a separation of the blood flow to the inner stripe from that to the inner medulla. Descending vasa recta supplying the inner medulla traverse the inner stripe within the vascular bundles. Therefore blood flowing to the inner medulla has not been exposed previously to tubules of the inner or outer stripe. All ascending vasa recta originating from the inner medulla traverse the inner stripe within the vascular bundles. Thus blood that has perfused tubules of the inner medulla does not subsequently perfuse tubules of the inner stripe. However, the blood returning from either the inner medulla or the inner stripe afterward does perfuse the tubules of the outer stripe. This arrangement in the outer stripe may function as the ultimate trap to prevent solute loss from the medulla.
The intrarenal veins accompany the arteries. Central to the renal drainage of the kidney are the arcuate veins, which, in contrast to arcuate arteries, do form real anastomosing arches at the corticomedullary border. The arcuate veins accept the veins from the cortex and the renal medulla. The arcuate veins join to form interlobar veins, which run alongside the corresponding arteries.
The intrarenal arteries and the afferent and efferent glomerular arterioles are accompanied by sympathetic nerve fibers and terminal axons representing the efferent nerves of the kidney.1 Tubules have direct contact to terminal axons only when the tubules are located around the arteries or the arterioles. Tubular innervation consists of “occasional fibers adjacent to perivascular tubules.”4 The density of nerve contacts to convoluted proximal tubules is low; contacts to straight proximal tubules, thick ascending limbs of Henle loops, and collecting ducts (located in medullary rays and outer medulla) have never been encountered. The vast majority of tubular portions have no direct relationships to nerve terminals. Afferent nerves of the kidney are believed to be sparse.5
Nephron
Renal Glomerulus (Renal Corpuscle)
The glomerulus comprises a tuft of specialized capillaries attached to the mesangium, both of which are enclosed in a pouch-like extension of the tubule, the glomerular capsule, or Bowman capsule (Figs. 1-4 and 1-5). The capillaries and mesangium are covered by epithelial cells (podocytes), forming the visceral epithelium of Bowman capsule. At the vascular pole, this structure is reflected to become the parietal epithelium of Bowman capsule. At the interface between the glomerular capillaries and the mesangium on one side and the podocyte layer on the other side, the glomerular basement membrane (GBM) develops. The space between both layers of Bowman capsule represents the urinary space, which at the urinary pole continues as the tubule lumen.
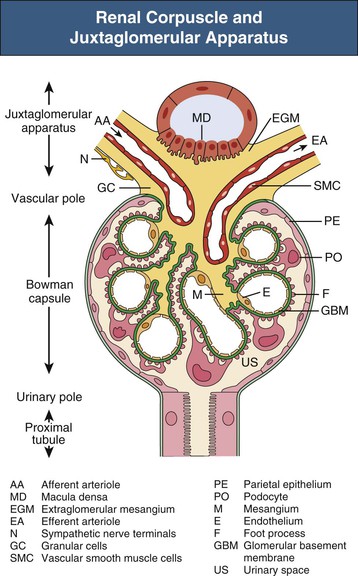
On entering the tuft, the afferent glomerular arteriole immediately divides into up to five primary capillary branches, each of which gives rise to an anastomosing capillary network representing a glomerular lobule. In contrast to the afferent arteriole, the efferent glomerular arteriole is already established inside the tuft by confluence of capillaries from each lobule.6 Thus the efferent arteriole has a significant intraglomerular segment located within the glomerular stalk.
Glomerular capillaries are a unique type of blood vessel consisting of only an endothelial tube (Figs. 1-6 and 1-7). A small stripe of the outer aspect of this tube directly abuts the mesangium; a major part bulges toward the urinary space and is covered by the GBM and the podocyte layer. This peripheral portion of the capillary wall is the filtration area. The glomerular mesangium represents the axis of a glomerular lobule to which the glomerular capillaries are attached.
Glomerular Basement Membrane
The GBM serves as the skeleton of the glomerular tuft. This membrane is a complexly folded sack with an opening at the glomerular hilum (see Fig. 1-4). The outer aspect of this GBM sack is completely covered with podocytes. The interior of the sack is filled with the capillaries and the mesangium. As a result, on its inner aspect, the GBM is in contact with either capillaries or the mesangium. At any transition between these two locations, the GBM changes from a convex pericapillary to a concave perimesangial course; the turning points are called mesangial angles.
In electron micrographs of traditionally fixed tissue, the GBM appears as a trilaminar structure, with a lamina densa bounded by two less dense layers, the lamina rara interna and lamina rara externa (see Fig. 1-7). Studies with freeze techniques reveal only one thick dense layer directly attached to the bases of the epithelium and endothelium.7
The major components of the GBM include type IV collagen, laminin, and heparan sulfate proteoglycans, as in basement membranes at other sites. Types V and VI collagen and nidogen (entactin) have also been demonstrated. However, the GBM has several unique properties, notably a distinct spectrum of type IV collagen and laminin isoforms. The mature GBM consists of type IV collagen made of α3, α4, and α5 chains (instead of α1 and α2 chains of most other basement membranes) and laminin 11, made of α5, β2, and γ1 chains.8 Type IV collagen is the antigenic target in Goodpasture disease (see Chapter 24), and mutations in the genes of the α3, α4, and α5 chains of type IV collagen are responsible for Alport syndrome (see Chapter 48).
Current models depict the basic structure of the GBM as a three-dimensional network of type IV collagen.7 The type IV collagen monomer consists of a triple helix that is 400 nm in length, with a large, noncollagenous globular domain at its C-terminal end called NC1. At the N terminus, the helix possesses a triple helical rod 60 nm long: the 7S domain. Interactions between the 7S domains of two triple helices or the NC1 domains of four triple helices allow type IV collagen monomers to form dimers and tetramers. In addition, triple helical strands interconnect by lateral associations through binding of NC1 domains to sites along the collagenous region. This network is complemented by an interconnected network of laminin 11, resulting in a flexible, nonfibrillar polygonal assembly that provides mechanical strength to the basement membrane and serves as a scaffold for alignment of other matrix components.
The electronegative charge of the GBM mainly results from the presence of polyanionic proteoglycans. The major proteoglycans of the GBM are heparan sulfate proteoglycans, including perlecan and agrin. Proteoglycan molecules aggregate to form a meshwork that is kept well hydrated by water molecules trapped in the interstices of the matrix. Abrahamson9 provides an up-to-date summary of the molecular organization of the GBM.
Mesangium
Three major cell types occur within the glomerular tuft, all of which are in close contact with the GBM: mesangial cells, endothelial cells, and podocytes. The mesangial/endothelial/podocyte cell ratio is 2 : 3 : 1 in the rat. The mesangial cells and mesangial matrix establish the glomerular mesangium. In addition, some studies suggest that macrophages bearing HLA-DR/Ia-like antigens may also rarely be found in the normal mesangium.
Mesangial Cells
Mesangial cells are irregular in shape, with many processes extending from the cell body toward the GBM (see Figs. 1-6 and 1-7). In these processes, dense assemblies of microfilaments are found, containing actin, myosin, and α-actinin.10 The processes are attached to the GBM directly or through the interposition of microfibrils (see later discussion). The GBM represents the effector structure of mesangial contractility. Mesangial cell–GBM connections are especially prominent alongside the capillaries, interconnecting the two opposing mesangial angles of the GBM.
Mesangial cells possess a great variety of receptors, including those for angiotensin II (Ang II), vasopressin, atrial natriuretic factor, prostaglandins, transforming growth factor β (TGF-β), and other growth factors (PDGFs, EGF, CTGF)11.
Mesangial Matrix
The mesangial matrix fills the highly irregular spaces between the mesangial cells and the perimesangial GBM, anchoring the mesangial cells to the GBM.6 The ultrastructural organization of this matrix is incompletely understood. In specimens prepared by a technique that avoids osmium tetroxide and uses tannic acid for staining, a dense network of elastic microfibrils is seen. Many common extracellular matrix proteins have been demonstrated within the mesangial matrix, including collagen types IV, V, and VI and microfibrillar protein components such as fibrillin and the 31-kilodalton microfibril-associated glycoprotein. The matrix also contains several glycoproteins, most abundantly fibronectin, as well as several types of proteoglycans.
Endothelium
Glomerular endothelial cells consist of cell bodies and peripherally located, attenuated, and highly fenestrated cytoplasmic sheets (see Figs. 1-6 and 1-7). Glomerular endothelial pores lack diaphragms, which are encountered only in the endothelium of the final tributaries to the efferent arteriole.6 The round to oval pores have a diameter of 50 to 100 nm. The luminal membrane of endothelial cells is negatively charged because of the cell coat of several polyanionic glycoproteins, including podocalyxin. In addition, the endothelial pores are filled with sieve plugs mainly made of sialoglycoproteins.12
Visceral Epithelium (Podocytes)
The visceral epithelium of Bowman capsule comprises highly differentiated cells, the podocytes (Fig. 1-8; see also Fig. 1-6
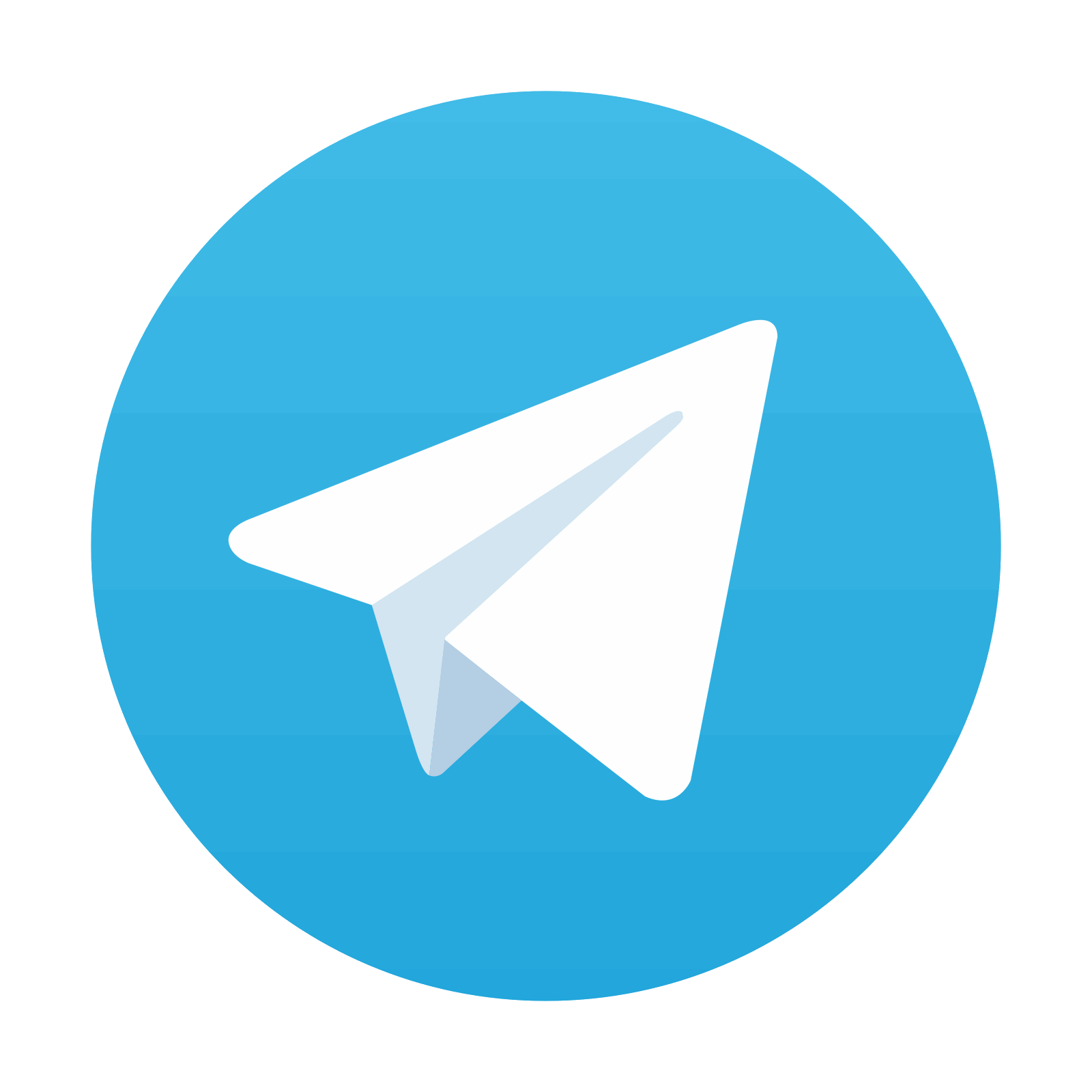
Stay updated, free articles. Join our Telegram channel

Full access? Get Clinical Tree
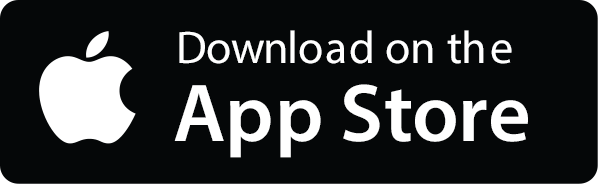
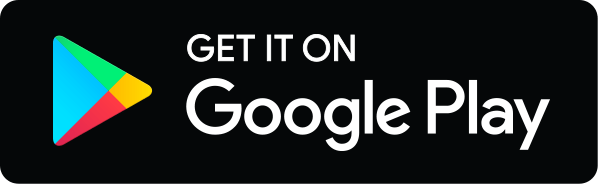