PERITONEAL DIALYSIS PERITONITIS—PATHOGENESIS
Organisms that colonize the skin, S. aureus and coagulase-negative staphylococci (CNS), are the most frequent pathogens isolated in PD peritonitis, accounting for 50% or more of cases in most series (2,3). The most frequent cause of PD peritonitis is “touch contamination,” which occurs when organisms are transferred from the hands of the patient or helper during connection or disconnection. Less common modes of infection are periluminal introduction of organisms from an exit-site or tunnel infection, transvisceral introduction of organisms from an intra-abdominal process, or rarely from hematogenous seeding. Once introduced into the peritoneum, the organisms encounter an environment that favors growth, with a high glucose content and few inflammatory cells initially. In the early stages of infection, the host immune response is inadequate to control the infection, with small numbers of peritoneal macrophages, very few neutrophils, and negligible quantities of host defense proteins such as immunoglobulins and complement. As the organisms proliferate, they produce toxins and other products that stimulate the resident macrophages to generate proinflammatory cytokines such as tumor necrosis factor (TNF), interleukin 1 (IL-1), interleukin 6 (IL-6), interleukin 8 (IL-8), which recruits inflammatory cells such as neutrophils, monocytes, and lymphocytes into the peritoneum. These cells and their products are responsible for the predominant symptoms of peritonitis—pain, cloudy effluent, and fever.
Factors that make the treatment of PD peritonitis more difficult are the characteristics of PD fluid and the presence of a foreign body, the catheter itself, in the peritoneum. Standard PD fluids are hyperosmolar and have a low pH, which may impair the function of neutrophils and macrophages. Although necessary to cure peritonitis, the constant exchange of PD fluid removes host phagocytic cells and host defense compounds from the peritoneum. Another factor that impairs host defense in PD peritonitis is the formation of biofilms. Biofilms are aggregates of organisms, adherent to a surface, usually embedded in an extracellular matrix composed of host compounds and products of the organism (4). Biofilms can form on infected PD catheters, particularly with infection by CNS species. Bacteria within the deeper portions of the biofilm do not divide as rapidly and therefore are far less susceptible to antibiotics and may persist despite clinical resolution of infection, providing a nidus for relapsing infection. Relapsing infection is often the result of biofilm formation and is an indication for catheter exchange.
Less common mechanisms of PD peritonitis include extraluminal spread from an exit-site or more often a tunnel infection producing a route for organisms to enter the peritoneum. These are usually clinically evident at the time of diagnosis of peritonitis. Peritonitis may arise from an intra-abdominal site, either a perforated viscus, focal intra-abdominal infection, transmural migration from the gut into the peritoneal cavity, or ascending infection from the female genital tract. The isolation of multiple enteric bacteria or the presence of particulate matter in the effluent suggests a perforated viscus and should prompt immediate imaging and surgical evaluation. Bacteremia or fungemia from an extraperitoneal site may also result in PD peritonitis.
EXIT-SITE INFECTION
Purulent drainage at the PD exit site, with or without erythema or induration, usually indicates an exit-site infection. Exit-site infection is a superficial infection that involves only the distal 2 cm or less of the catheter tunnel, which can spread proximally, resulting in a tunnel infection or frank peritonitis. The organisms that cause most exit-site infections are those that colonize the skin and exit site itself; S. aureus, CNS, Candida, and gram-negative enterics. Topical mupirocin or gentamicin cream applied to the exit site have been shown to reduce the incidence of exit-site infection overall (5,6) but increase the incidence of infection caused by antibiotic-resistant pathogens such as diphtheroids, Candida, and nontuberculous mycobacteria (NTM) (7).
Exit-site infection should be suspected if the patient develops purulent drainage around the catheter. Swelling, pain, and crust formation may also be present. Erythema, by itself is neither a sensitive nor a specific indicator of infection without other findings. Pericatheter drainage should be sent for Gram stain and culture. Do not culture the exit site in the absence of purulent drainage or other signs of infection because exit sites are rarely sterile and may be colonized by the same types of organisms that cause infection.
The goals of therapy are to prevent progression to a tunnel infection or peritonitis. Mild exit-site infections may be treated topically with chlorhexidine, dilute hydrogen peroxide, or gentamicin ophthalmic solution and close monitoring. In more severe infections, systemic therapy is indicated which can usually be administered orally. See TABLE 14.1 for dosing of oral antibiotics in PD patients. Gram staining of the exudate may be helpful in directing initial antimicrobial therapy, and culture and sensitivity will direct definitive therapy. If gram-positive cocci are seen, therapy should be directed against staphylococcal species and empiric choices would be dependent on the incidence of methicillin-resistant staphylococci in your program. With a low incidence of methicillin resistance, oral therapy with dicloxacillin 500 mg po qid or cephalexin 500 mg bid to tid would be adequate. If methicillin resistance is a concern, oral therapy with trimethoprim/sulfamethoxazole (TMP/SMX) 80/400 mg po qd or clindamycin 300 mg po tid would be a better choice. Linezolid (400 to 600 mg po bid) is also an option but is much more expensive and may produce adverse effects with courses longer than 14 days (8,9). If gram-negative bacteria are seen on Gram stain, oral ciprofloxacin 250 mg po bid should be used pending culture and sensitivity results. If the Gram stain is unavailable or unrevealing, empiric therapy should cover both staphylococcal species and gram-negative bacteria including Pseudomonas. Use of topical antimicrobials for prevention can affect the types of organisms responsible for exit-site infection. In patients receiving topical mupirocin, gram-negative organisms are more likely, whereas topical gentamicin results in more frequent staphylococcal and fungal infection (7).
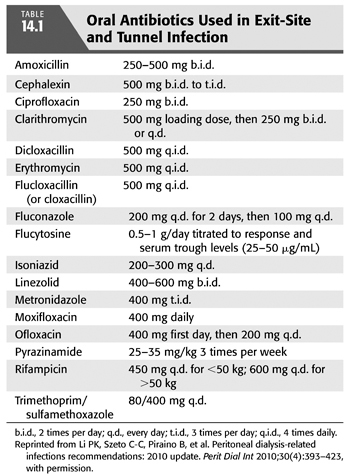
Upon receipt of culture and sensitivity results, therapy should be focused. For methicillin-susceptible staphylococcal species, oral therapy with cephalexin or dicloxacillin should be used. Streptococcal species should be treated with amoxicillin 250 to 500 mg po bid or cephalexin and with enterococci oral amoxicillin. “Community-acquired” strains of methicillin-resistant Staphylococcus aureus (MRSA) are often susceptible to TMP/SMX, clindamycin, doxycycline 100 mg po bid or minocycline 100 mg po bid and can be treated orally with these. Hospital-acquired strains maybe susceptible only to vancomycin and can be treated with vancomycin 15 to 30 mg/kg every 5 to 7 days administered intraperitoneally or oral linezolid if susceptible. For more difficult MRSA infections, rifampin 600 mg po daily may be added (1). Infection with gram-negative enterics should be treated with oral ciprofloxacin or possibly TMP/SMX depending on drug susceptibilities. Exit-site infections caused by Pseudomonas aeruginosa can be more difficult to treat and may relapse (10). If the isolate is susceptible, initial therapy with oral ciprofloxacin is appropriate. In the case of ciprofloxacin resistance, a slow response to oral ciprofloxacin or a relapsing infection, treatment with intraperitoneal tobramycin 0.6 mg/kg IP daily, cefepime 1 g IP qd, or meropenem 1 g IP qd should be added. Treatment for pseudomonal exit-site infection should be continued for at least 3 weeks or until the infection has resolved clinically. Nonpseudomonal exit-site infections should be treated for at least 2 weeks or until resolution.
The goal of treating exit-site infections is to prevent progression to a tunnel infection or peritonitis. An exit-site infection that is refractory to treatment or progresses to a tunnel infection or peritonitis requires catheter removal and replacement. The one exception is CNS infection, which in some cases can be successfully treated without catheter replacement. In the absence of peritonitis, catheter removal and replacement can be performed in a one-stage procedure, with catheter placement at a different site.
TUNNEL INFECTION
Erythema, swelling, or pain along the catheter extending more than 2 cm proximal to the catheter–skin interface is indicative of a tunnel infection. These infections usually result from the spread of bacteria from the exit site along the subcutaneous portion of the catheter and pose a higher risk of peritonitis. Ultrasound of the subcutaneous track of the catheter may reveal pericatheter fluid or abscess formation. The most common pathogens causing tunnel infection are S. aureus and Pseudomonas. Gram stain and culture of material milked from the exit site should be obtained, and appropriate antibiotic therapy should be started. In most cases, antibiotic therapy alone is inadequate and catheter removal is necessary—particularly with infections caused by S. aureus or Pseudomonas.
PERITONEAL DIALYSIS PERITONITIS
Peritonitis–Diagnosis
Prompt recognition, diagnosis, and treatment of PD peritonitis is essential for patient safety and preservation of the peritoneal membrane. Peritonitis may follow an exit-site or tunnel infection or an intra-abdominal source but most often results from contamination of the external catheter connection by breaks in sterile technique, touch contamination. The earliest signs of peritonitis are cloudy dialysate effluent and/or abdominal pain, which may be accompanied by fever or nausea and vomiting. Patients using automated PD may be less likely to notice cloudy dialysate. The first drain is most likely to be cloudy. The degree of inflammation and severity of abdominal pain is dependent on the virulence of the infecting organism. S. aureus, gram-negative rods, and β-hemolytic streptococci generally produce more severe abdominal pain and are more likely to be associated with fever than CNS. Patients should be educated to be alert for these signs and symptoms and to notify their providers for prompt evaluation.
Diagnosis of peritonitis is made by examining the dialysis effluent. In patients with prominent systemic symptoms, blood cultures should be obtained as well (11). Effluent should be sent for cell counts, white blood cell (WBC) differential, Gram stain, and culture prior to administering antibiotics whenever possible. Dialysate WBC >100/μL and >50% polymorphonuclear cells (PMNs) from a sample of fluid that has had a dwell of >2 hours is very suggestive of peritonitis. In patients receiving APD, fluid samples with <100 WBC but >50% PMNs usually have peritonitis. In a patient with a dry abdomen, 1 L of dialysate is infused, allowed to dwell for 1 to 2 hours, and then removed for cell counts and microbiologic studies.
Fluid specimens must be obtained for microbiologic studies before administering antimicrobials whenever possible to identify the infecting organisms and their drug susceptibilities to guide effective antimicrobial therapy. Five to 10 mL of fluid should be inoculated directly into aerobic and anaerobic blood culture bottles at the bedside. Use of BACTEC/Alert culture bottles may improve yield and reduce culture-negative cases (12). In addition, 50 mL of fluid should be centrifuged at 3,000 g for 15 minutes and the sediment resuspended in 3 to 5 mL of saline. The sediment should be examined with a Gram stain and inoculated into liquid blood culture bottles and solid bacterial media plates. Cultures are usually positive in 24 to 48 hours, but if liquid media cultures are negative at 3 days, they should be subcultured on solid media and examined for an additional 3 to 5 days. ISPD guidelines suggest a benchmark for culture negative peritonitis at <20% of cases. In the patient with negative cultures who fails to respond clinically to empiric antibiotic therapy by 3 days, repeat PD effluent sampling for cell counts and microbiologic studies should be performed. In addition to repeating routine bacteriologic studies, consideration of fastidious bacterial, mycobacterial, and fungal causes should be explored with additional microbiologic studies.
Initial Empiric Therapy for Peritonitis
Prompt initial empiric antimicrobial therapy is essential for optimal short- and long-term outcomes (FIGURE 14.1). A positive peritoneal fluid Gram stain can guide initial empiric therapy, focusing on gram-positive or gram-negative bacteria. If the Gram stain is negative, empiric antibiotic therapy, including coverage for both gram-positive and gram-negative bacteria should be started promptly. Antibiotics should be administered intraperitoneally, added to the dialysate. The choice of empiric therapy should be determined by the local prevalence of antimicrobial resistance in gram-positive and gram-negative bacterial causes of peritonitis. In most programs, gram-positive bacteria account for 50% or more of cases and gram-negative enterics 10% to 20%. Empiric coverage for gram positives with cefazolin 15 to 20 mg/kg IP qd or vancomycin 15 to 30 mg/kg IP every 5 to 7 days is recommended, with the choice dependent on the local incidence of methicillin-resistant staphylococcal species. Empiric coverage for gram-negative bacteria should include coverage for P. aeruginosa. Choices include ceftazidime 1 to 1.5 g IP qd, cefepime 1 g IP qd, gentamicin 0.6 mg/kg IP qd, or tobramycin 0.6 mg/kg IP qd, again depending on local antimicrobial resistance patterns. A recent episode of peritonitis or exit-site infection or recent antibiotic therapy may modify initial empiric antibiotic choices. Results of peritoneal fluid cultures and drug susceptibilities will direct definitive therapy for peritonitis.
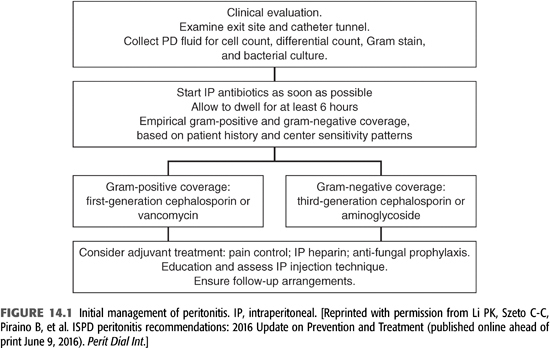
Intraperitoneal Antibiotics for Peritonitis
Intraperitoneal administration of antibiotics is the recommended route of administration for the treatment of PD peritonitis for a number of reasons (13) (TABLE 14.2). IV access and continued IV administration is not necessary. Antibiotics added to the dialysate are delivered in high concentrations to the site of infection. The peritoneal membrane is permeable to most intraperitoneally administered antibiotics, resulting in therapeutic serum levels, which allows diffusion back into the peritoneum when the patient is not undergoing dialysis. If antibiotics are administered intermittently, the antibiotic containing fluid should dwell for at least 6 hours, usually with a daytime dwell in patients receiving APD. There is less data on the dosing and efficacy of IP antibiotics in patients receiving APD, particularly with β-lactam compounds, and some recommend converting to continuous ambulatory peritoneal dialysis (CAPD), if possible, during therapy for peritonitis. (14)
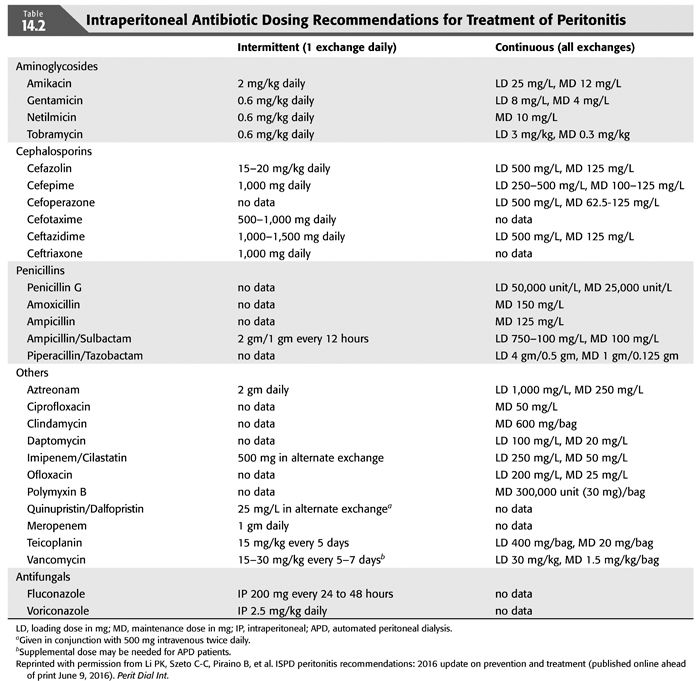
Peritonitis Treatment Course and Outcomes
Upon receiving the results of peritoneal fluid cultures and drug susceptibilities, antibiotic therapy should be focused on the infecting pathogen using the narrowest spectrum, least toxic agent. Duration of treatment is usually 14 to 21 days depending on the pathogen. Patient symptoms should be improving, and peritoneal effluent should be clear after 2 to 4 days of definitive treatment. In one study, effluent WBC of >1,000 on day 3 of treatment predicted failure of therapy (15). If the effluent does not clear after 5 days of culture-directed therapy, ISPD guidelines define this as refractory peritonitis and recommend consideration of catheter removal. Catheter removal should also be considered for culture-negative cases if the effluent does not clear in 5 days. Failure of the effluent to clear after 5 days of appropriate empiric therapy should also raise suspicion for infections caused by fastidious bacterial pathogens, mycobacterial, or filamentous fungal causes. This should prompt repeat cultures for bacteria, fungal, and acid-fast bacteria.
Relapsing peritonitis is defined as a second episode of peritonitis caused by the same pathogen or as a culture negative case within 4 weeks after the end of treatment for the first episode. Recurrent peritonitis is a second episode occurring within 4 weeks of completion of therapy for a prior one, caused by a different organism (16,17). Repeat peritonitis is a second episode caused by the same organism as the first, more than 4 weeks after the completion of therapy. These three forms of peritonitis are associated with subsequent cases of peritonitis. ISPD guidelines suggest that removal and replacement of the PD catheter be considered to prevent damage to the peritoneal membrane and technique failure (1).
Catheter Management in Peritoneal Dialysis Peritonitis
Appropriate management of peritonitis, tunnel, and exit-site infections is focused on resolving the current infection, preventing subsequent infection, and preserving the peritoneal membrane. Patients with refractory exit-site and tunnel infections should have their catheters removed and replaced. Catheter removal and replacement simultaneously in a one-stage procedure is feasible in patients with refractory exit-site infections and in patients with relapsing CNS infection if the effluent has cleared on appropriate therapy. Patients with refractory infections caused by S. aureus, Pseudomonas, fungi, or mycobacteria should have their catheters removed in a timely fashion and suspend PD until their infections have resolved before catheter replacement.
ORGANISM-SPECIFIC TREATMENT AND MANAGEMENT OF PERITONEAL DIALYSIS PERITONITIS
Two to 3 days after obtaining peritoneal fluid, cultures are usually growing a pathogen (FIGURES 14.2 and 14.3). If the peritoneal Gram stain was positive on the initial sample or when cultures become positive, your microbiology laboratory will be able to identify the organism as gram-positive, gram-negative, or yeast and whether it is cocci or bacilli. Where available, polymerase chain reaction (PCR) (BioFire) or matrix-assisted laser desorption/ionization time-of-flight (MALDI-TOF) can rapidly identify the species of the infecting organism and also determine whether it contains resistance genes such as mecA for methicillin resistance, vancomycin resistance genes in enterococci, and carbapenem resistance in gram-negative bacilli. This information would allow focusing or changing antibiotic therapy.
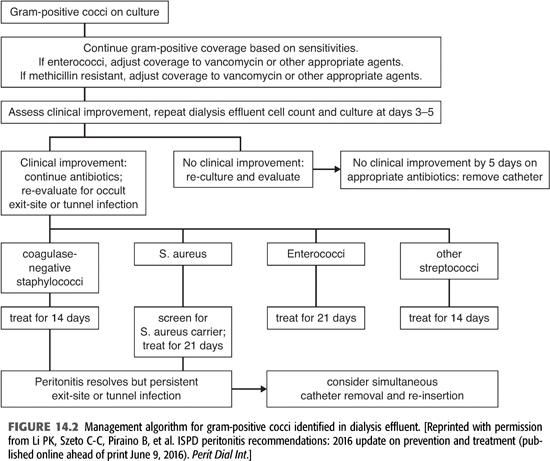
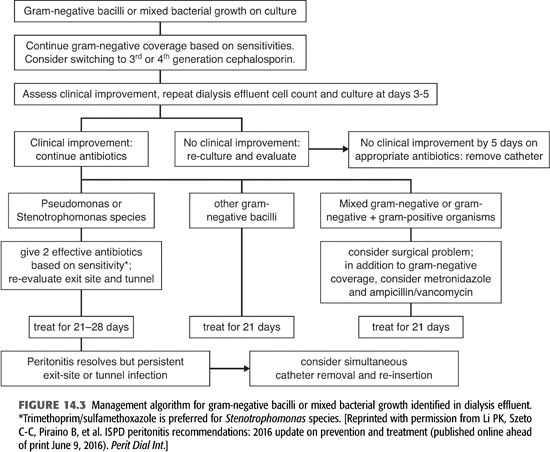
Coagulase-Negative Staphylococcal Peritonitis
CNS are the most common cause of PD peritonitis in most series. These organisms are ubiquitous colonizers of human skin and are a group of species, one of which is Staphylococcus epidermidis, the commonest pathogen in PD peritonitis (18–21). Peritonitis caused by these organisms is almost always the result of touch contamination in the course of manipulating the catheter or connectors during dialysis. CNS species are not very virulent organisms and usually produce a milder form of peritonitis with less pain, peritoneal inflammation, and fewer systemic symptoms. However, these organisms have a higher propensity to produce biofilms on catheters due to their production of “slime,” an extracellular matrix of bacterial and host compounds (22). Organisms in biofilms are much more resistant to antimicrobials, being embedded in slime, impairing antibiotic access, and having reduced replication in the deeper levels of the complex. Probably because of biofilm production, CNS are frequent causes of relapsing and repeat cases of peritonitis (17). Relapsing or repeat peritonitis by these organisms requires catheter removal and replacement in most cases.
Initial empiric antibiotic therapy should always include antibiotic coverage for these organisms. If methicillin-resistant organisms are uncommon in your program, cefazolin can be used empirically; otherwise, vancomycin should be used for empiric therapy. In a series of 115 cases of CNS PD peritonitis cases from Brazil, 70% of their isolates were methicillin-resistant and initial therapy including vancomycin empirically improved outcomes (21). Upon receipt of culture and sensitivity results, methicillin-susceptible isolates can be treated with cefazolin, and methicillin-resistant strains with vancomycin. Intraperitoneal cefazolin is administered 15 to 20 mg/kg to one exchange a day, with a long dwell (>6 hours). There are some concerns about using cefazolin in patients receiving APD in that peritoneal drug levels may be inadequate during APD due to more rapid exchanges, with some authorities recommending conversion to CAPD during cefazolin therapy (1). However, the published experience suggests that these patients may receive therapy on APD, with one study showing equivalent cure rates in patients treated on APD or CAPD (22) and another study showing higher failure rates in those switched to CAPD during therapy (23). This is not a concern with vancomycin therapy, which is administered every third to fifth day intraperitoneally.
Clinical response is usually prompt, with resolution of symptoms and clearing of the effluent, with reduction in peritoneal WBC by 3 to 5 days. Patients with a good clinical response can be treated for 14 days. Failure to respond by 3 to 5 days should prompt reevaluation with repeat cultures and consideration of catheter removal, especially in the presence of an exit-site or tunnel infection. Relapsing or repeat episodes should also prompt considering catheter removal and replacement, which can be done as a one-stage procedure after treatment of peritonitis.
Staphylococcus aureus Peritonitis
In contrast to CNS species, S. aureus is a much more virulent organism, producing clinically more severe peritonitis with a significant risk of failure, morbidity, and mortality (24,25). The cause is often touch contamination but also may result from concurrent exit-site or tunnel infection. Initial empiric therapy should include cefazolin or vancomycin 15 to 30 mg/kg/d IP every 5 to 7 days, depending on the local prevalence of methicillin-resistant strains, followed by focused therapy based on the drug susceptibility of the infecting strain. ISPD guidelines recommend the addition of rifampin, 600 mg po qd for 7 days in addition to the primary antibiotic (1). Rifampin has synergistic activity on S. aureus and is active on bacteria adherent to the catheter and in biofilms. If rifampin is used, be aware of its induction of multiple drug metabolizing enzymes and its effect on concomitant medications.
S. aureus usually produces more clinically severe peritonitis, with pain, higher peritoneal effluent WBC, and systemic signs of infection such as fever and signs of sepsis. For methicillin-susceptible strains, cefazolin 15 to 20 mg/kg IP qd or continuous dosing with cefazolin, a 500 mg/L IP loading dose followed by 125 mg/L IP with oral rifampin. Methicillin-resistant strains should be treated with an initial IP loading dose of vancomycin, 15 to 30 mg/kg up to 2 g, followed by 1 g IP every 3 to 7 days, based on serum vancomycin levels. Trough serum vancomycin levels of <15 μg/mL should prompt redosing with vancomycin. Patients who are intolerant of vancomycin or have vancomycin-resistant strains can be treated with daptomycin 100 mg/L IP loading dose and then 20 mg/L IP continuously, linezolid 300 to 600 mg po bid, or perhaps ceftaroline. Ceftaroline is a third-generation cephalosporin with good MRSA activity but has not been used in PD patients at this point (26). Oral rifampin 600 mg should be given as well. Patients with a good clinical response, with decreasing pain and clearing of the effluent should be treated with 21 days of therapy. Failure to respond after 5 days of appropriate antibiotic therapy, or the presence of an exit-site or tunnel infection, are indications for prompt catheter removal and converting to hemodialysis for 2 to 3 weeks before catheter replacement (1). MRSA peritonitis is associated with an increased risk of technique failure and permanent switching to hemodialysis (25).
Streptococcal Peritonitis
Streptococcal species are less common causes of PD peritonitis than staphylococcal species. Like staph species, the clinical presentation depends on virulence of the species, with β-hemolytic streptococci, Groups A, B, etc., resembling S. aureus and viridans streptococci more resembling the clinical features of CNS (27). The mode of infection is probably touch contamination in most cases, but viridans streptococci are inhabitants of the alimentary tract so a gastrointestinal (GI) source is possible. Initial empiric therapy with cefazolin 15 to 20 mg/kg/d IP or vancomycin 15 to 30 mg/kg IP every 3 to 7 days IP will cover all the β-hemolytic streptococci and most viridans streptococci, which have been shown to have increasing resistance to penicillins and cephalosporins (28). Results of cultures and sensitivities will allow focused therapy with a single agent. Continuous dosing with ampicillin 125 mg/L IP has been recommended for penicillin susceptible strains (1). Some studies have shown that ampicillin in solution begins to lose antimicrobial activity after of mixing, creating problems with patients on home therapy. A better choice may be cefazolin, which has good activity against these strains and is stable for 8 days at room temperature and 14 days refrigerated in solution (29).
β-Hemolytic streptococcal peritonitis presents like S. aureus, with significant abdominal pain, high peritoneal WBCs, and frequent fever and signs of sepsis. These organisms remain very susceptible to most β-lactam antibiotics and peritonitis usually responds promptly to appropriate antibiotic therapy, including cefazolin. Therapy should be continued for 14 days. Failure to respond promptly should raise questions about an exit-site or tunnel infection, or a concomitant skin and soft tissue infection or other focus.
Viridans streptococci, like CNS, include a large number of species and, like CNS, are not very virulent organisms. They are normal inhabitants of the alimentary tract, including the mouth and upper and lower GI tract and probably produce peritonitis through touch contamination, occasionally via the GI tract or hematogenously following invasive procedures involving mucosal surfaces without prophylactic antibiotics (30). Peritonitis caused by these organisms resembles CNS, with less severe abdominal pain, milder inflammatory changes on peritoneal fluid examination, and fewer systemic symptoms like fever (31). One study, however, identified an increased risk of recurrent and refractory peritonitis following an episode of viridans streptococci peritonitis (32). For penicillin-susceptible strains, continuous IP ampicillin has been recommended, but because of poor stability in solution, IP cefazolin might be a better choice. Penicillin-resistant strains with minimum inhibitory concentration (MIC) >4 μg/mL or relatively resistant strains with penicillin MICs >0.5 μg/mL should be treated with IP vancomycin. Therapy should be continued for 14 days.
The organism formerly known as Streptococcus bovis has been reclassified as Streptococcus gallolyticus. In a series of 20 patients with 23 episodes of peritonitis caused by S. gallolyticus reported by Yap et al. (33), some degree of penicillin resistance was common. Patients were successfully treated with cefazolin or vancomycin and an aminoglycoside for 14 days. S. bovis/S. gallolyticus bacteremia is classically associated with GI malignancies, particularly colon cancer. Ten of their 20 patients underwent diagnostic studies—colonoscopy or barium enema—and none were found to have colon cancer.
Enterococcal Peritonitis
Enterococcal species are normal inhabitants of the GI tract and are more common causes of peritonitis than streptococcal species in most series. The source of infection is usually touch contamination, but GI tract contamination also occurs and should be suspected if other enteric organisms are present. In fact, in some series, nearly half of enterococcal peritonitis cases were polymicrobial, and polymicrobial infections were associated with high rates of catheter loss and permanent transfer to hemodialysis (34,35). The clinical presentation of enterococcal peritonitis, without other organisms, is moderately severe in terms of pain and systemic symptoms. Cephalosporins have no activity against enterococci and aminoglycosides alone have very little so that empiric regimens containing cephalosporins or a cephalosporin and aminoglycoside have virtually no effect on enterococcal peritonitis. Vancomycin covers most enterococci, the exception being vancomycin-resistant enterococci (VRE).
Therapy of enterococcal peritonitis can be problematic, given that the organism is not susceptible to most commonly used antibiotics. Enterococcus faecalis is usually susceptible to ampicillin, penicillin, and piperacillin as well as vancomycin. Occasional strains of E. faecalis are resistant to vancomycin (VRE) but remain susceptible to penicillins. Ampicillin IP has been recommended as the drug of choice, but problems with stability in solution requires that it be reconstituted right before use. Piperacillin is stable in solution up to 8 days refrigerated, but there is little published experience in PD peritonitis. Vancomycin is commonly used and is recommended as the first choice in the 2016 ISPD guidelines, which also recommend the addition of IP aminoglycoside in “severe” cases of enterococcal peritonitis (1). Enterococcus faecium is inherently resistant to ampicillin and penicillin, making vancomycin the drug of choice. Most strains of VRE are E. faecium, which are resistant to all commonly used classes of antibiotics. Case reports and a few small series have reported successful treatment of VRE peritonitis with daptomycin. Daptomycin has most often been used IP with a loading dose of 100 mg/L for at least a 6-hour dwell and then 20 mg/L for APD or CAPD (36,37). Daptomycin has also been given at 7 mg/kg every 48 hours IP (38) and 5 mg/kg IV every 48 hours (39). Linezolid has also been used to treat VRE peritonitis. Because of presumed instability in dialysis fluids, linezolid has been given systemically, 600 mg IV every 12 hours (40,41). The bioavailability of linezolid given orally is 100%, so if the patient is taking po meds, po administration is certainly reasonable (42). Also be aware of the potential for hematologic and neurologic toxicities of linezolid with doses of 600 mg bid for courses exceeding 10 days (8,9). Quinupristin/dalfopristin has been used successfully treat E. faecium VRE peritonitis (43), but this compound has no activity on E. faecalis. The recommended duration of therapy for VRE peritonitis is 21 days. A suboptimal response to therapy should prompt consideration for catheter removal.
Corynebacterium Peritonitis
The genus Corynebacterium includes a number of species of small, gram-positive rods, often referred to as diphtheroids. These organisms, like CNS, are part of normal skin flora, of low pathogenicity, and usually produce infections related to implanted devices. In most case series, Corynebacterium account for less than 3% of peritonitis cases. This group of organisms is of low pathogenicity, and peritonitis resembles CNS clinically. Infection most likely occurs through touch contamination. Most Corynebacterium strains are susceptible to cefazolin. The notable exception is Corynebacterium jeikeium, which is resistant to β lactams and many other classes but is susceptible to vancomycin.
The optimal treatment of Corynebacterium peritonitis is not clear based on the two largest case series. A series of 27 patients reported from Hong Kong (44) found a better overall response and fewer recurrences after treatment with vancomycin for 2 to 3 weeks than with cephalosporins. In contrast, another series of 82 patients with Corynebacterium peritonitis found that 2 weeks of therapy with cefazolin was comparable to vancomycin or longer treatment courses (45). In both studies, recurrent peritonitis was frequent. One study also reported that in cases of refractory peritonitis, delaying catheter removal beyond 7 days resulted in 90% technique failure with permanent transfer to hemodialysis (45).
Gram-Negative (Enterobacteriaceae) Peritonitis
Gram-negative enteric bacteria, the family Enterobacteriaceae, are normal inhabitants of the gastrointestinal tract. The most common species causing PD peritonitis are Escherichia coli, Klebsiella, and Enterobacter species (46–48). The incidence of these organisms varies in different centers but usually accounts for 10% to 20% of PD peritonitis cases. Their relative percentage has increased over the past two decades, probably due to decreases in staphylococcus cases as a result of improved connective technologies and the use of mupirocin for exit-site prophylaxis. The mechanisms of infection are probably touch contamination but also exit-site and tunnel infections. Some infections may arise from an intra-abdominal source, constipation, or transmural migration of bacteria. Multiple species on Gram stains and culture suggest an intra-abdominal infection or perforated viscus. The clinical presentation is moderately severe, with fever in about one-third of cases.
Treatment of gram-negative enteric peritonitis is more complex than gram-positive bacteria, given the variety of species and differing antibiotic susceptibilities. Initial empiric therapy with a broad-spectrum cephalosporin IP, like cefepime or ceftazidime or an aminoglycoside, will cover most of these organisms. Results of culture and sensitivity will allow focused therapy. In a series of 210 patients with gram-negative enteric peritonitis, therapy with a single effective antibiotic was associated with a 39% failure rate and a high relapse and recurrence rate (46). Treatment with two effective agents improved response rates and decreased relapse. Depending on drug susceptibilities, a broad-spectrum β-lactam IP like cefepime or ceftazidime combined with either an aminoglycoside or a fluoroquinolone, ciprofloxacin or levofloxacin, would be reasonable choices.
Gram-negative enterics have a variety of potential resistance mechanisms (49). Enterobacter, Serratia, and Citrobacter species have a chromosomal β-lactamase enzyme, AmpC, which is derepressed when exposed to β-lactam antibiotics. Expression of AmpC results in resistance to cephalosporins and is not inhibited by β-lactamase inhibitors. The last decade has seen the emergence and spread of two novel resistance mechanisms. Extended spectrum β-lactamases (ESBLs) are plasmid mediated enzymes, first described in Klebsiella pneumoniae, but are present in many other gram-negative bacteria as well. Strains expressing ESBLs are resistant to all cephalosporins but remain susceptible to carbapenems and most β-lactam/β-lactamase inhibitors (50–52). Even more problematic is the emergence of carbapenem-resistant Enterobacteriaceae (CRE), also known as KPC—Klebsiella pneumoniae carbapenemase (53). These β-lactamase enzymes are carried on mobile genetic elements and induce resistance to all β-lactam antibiotics, including carbapenems. In addition, CRE strains are usually resistant to fluoroquinolones, variably resistant to aminoglycosides, and often only susceptible to polymyxin B and colistin. Colistin has been given systemically to treat PD peritonitis, 300 mg IV on day 1 and then 150 to 200 mg IV qd. There is little experience in the occurrence or treatment of CRE infections in PD peritonitis or the use of colistin or polymyxin B intraperitoneally (54).
Nonfermenting (Aerobic) Gram-Negative Rods—Pseudomonas, Acinetobacter, and Stenotrophomonas
Pseudomonas
Pseudomonas species are aerobic, nonfermenting gram-negative rods that are inherently resistant to many antibiotics. Pseudomonas, most often P. aeruginosa, usually accounts for less than 5% of peritonitis cases in most programs but was the causative agent in 13% of cases in a series from Hong Kong (55). Recent antibiotic therapy appears to predispose for Pseudomonas peritonitis. Catheter infection, either an exit-site or a tunnel infection, is often present and is an indication for catheter removal. Clinically, compared to other bacterial pathogens, pseudomonas peritonitis results in a twofold increased risk of catheter removal and permanent transfer to hemodialysis (56).
Treatment with two effective anti-pseudomonal antibiotics, based on drug susceptibility studies, appears to improve outcomes. Oral ciprofloxacin combined with IP cefepime, ceftazidime, piperacillin, aminoglycoside, meropenem, or imipenem appear to be the most efficacious combinations. In Szeto’s (55) series, outcomes were better with cephalosporin versus aminoglycoside combinations. In patients with a good primary response, antibiotics should be continued for 3 weeks. In refractory cases or in the presence of a catheter infection, prompt catheter removal improves outcomes. Following catheter removal, transfer to hemodialysis with 2 weeks of parenteral anti-pseudomonal therapy is recommended prior to catheter replacement.
Acinetobacter
Acinetobacter species are nonfermenting aerobic, gram-negative rods that are frequent agents of health care–associated infection (57). Most human infections are caused by Acinetobacter baumannii, which is often multidrug-resistant. Acinetobacter, like Pseudomonas, is inherently resistant to many antibiotics, frequently develops resistance during therapy, and not infrequently requires treatment with colistin or polymyxin B because of resistance to all other classes of drugs. Interestingly, most Acinetobacter strains are susceptible to ampicillin/sulbactam due to susceptibility to the sulbactam component. In a series of seven patients with Acinetobacter peritonitis reported by Zhang (58), three patients with multidrug-resistant strains failed antibiotic therapy and transferred to hemodialysis. Peritonitis caused by Acinetobacter should probably treated like Pseudomonas with two effective drugs based on drug susceptibilities, for 3 weeks, with early catheter removal for refractory cases.
Stenotrophomonas maltophilia
Stenotrophomonas maltophilia is another aerobic gram-negative bacillus that causes health care–associated infections and is multidrug-resistant. This organism is inherently resistant to all carbapenems, possessing a chromosomal carbapenemase, most fluoroquinolones, aminoglycosides, and tetracyclines (59). It is most susceptible to TMP/SMX, ticarcillin/clavulanate (no longer available in the United States), tigecycline, and colistin and polymyxin. Stenotrophomonas is not a common cause of PD peritonitis, but in three small case series, failure with catheter loss occurred in about half of treated patients (60,61). The most effective therapy seems to be TMP/SMX combined with an additional effective drug for 3 to 4 weeks of therapy.
Polymicrobial Peritoneal Dialysis Peritonitis
Polymicrobial peritonitis is a case of peritonitis in which more than one organism is isolated in a single episode of peritonitis. This occurs in 10% or less of peritonitis episodes. The isolation of multiple species of organisms, particularly gram-negative enterics and anaerobic bacteria, in a patient with more severe abdominal pain and systemic signs of sepsis should raise concerns for a perforated viscus or other serious intra-abdominal infection and should prompt immediate surgical consultation. In most case series of polymicrobial peritonitis, these intra-abdominal catastrophes occur in fewer than 10% of patients with polymicrobial peritonitis (62–64).
In the majority of cases of polymicrobial peritonitis, the pathogenesis is probably similar to monomicrobial cases, the result of touch contamination or catheter-related infection. Outcomes seem to be best with mixed infection with gram-positive cocci. Isolation of gram-negative bacteria and particularly fungi is associated with higher rates of failure, catheter removal, and permanent transfer to hemodialysis.
Culture-Negative Peritonitis
Culture negative peritonitis is defined as clinical signs of peritonitis, cloudy dialysate with more than 100 WBC/μL, >50% PMNs, and with negative peritoneal fluid cultures after 3 days. PD guidelines suggest a benchmark of fewer than 20% culture-negative cases of peritonitis. A recent history of antibiotic use or initiating antibiotic therapy for peritonitis before obtaining peritoneal fluid cultures is common in these cases. It is thought that most of these cases are due to gram-positive bacteria through touch contamination based on the observation that most patients respond to standard empiric antibiotic regimens (65). If peritoneal fluid cultures remain negative after 3 days of therapy, peritoneal WBC and differential should be repeated. If the patient responds to empiric antibiotic therapy with clearing of the effluent, and persistent negative cultures, gram-negative coverage can be discontinued and cefazolin or vancomycin should be continued for 14 days.
If there is a lack of clinical response after 3 days of antibiotic therapy, peritoneal fluid WBC, differential, and cultures should be repeated. Possible causes in these patients include resistant bacteria, fastidious bacteria, mycobacterial infection, fungal infection (other than candida), or a noninfectious cause. In addition to routine bacterial cultures, peritoneal fluid should be sent for fungal and mycobacterial cultures and bacterial cultures should be held for a longer period of time and subcultured on enriched and specialized media. If there is no clinical improvement after 5 days of therapy, catheter removal should be considered.
Fungal Peritonitis
Fungal peritonitis usually accounts for less than 5% of cases in most centers, but some have higher rates (66). The clinical presentation resembles bacterial peritonitis with cloudy dialysate and abdominal pain. More than half of patients with fungal peritonitis will have a history of antibiotic therapy in the prior month, often for an episode of bacterial peritonitis (67). The presumed mechanism is that antibiotic therapy reduces the normal bacterial flora of the skin and GI tract, facilitating overgrowth or colonization by candida or other fungi. PD fluid studies are similar to bacterial infection with elevated WBC and neutrophilic predominance. Occasionally, eosinophils will be seen in PD effluent with infection by some filamentous fungi (molds). Candida species are responsible for 90% or more of fungal peritonitis cases; infection with molds is less common. On Gram stain, Candida species are seen as gram-positive, ovoid cells, about 5 μm in diameter. Mold species do not stain with Gram stain. Calcofluor white or Gomori methenamine silver stains will detect molds and yeasts if enough organisms are present in the specimen. β-D-Glucan is an important component of most fungal cell walls and is usually measured in the serum in patients with suspected fungal infections (68). It might be a useful adjunct in the diagnosis of suspected fungal peritonitis to measure serum and peritoneal fluid β-D-glucan levels—but to my knowledge, this has not been done. Candida species grow well on standard bacterial culture media, usually taking a day or two longer than bacteria. In contrast, molds require specialized fungal culture media and usually take longer to grow.
In the 20th century, Candida albicans was the predominant species in PD peritonitis and most other sites of candida infection. In the last 15 years, C. albicans now accounts for fewer than 50% of cases (69–71). Of the non-C. albicans species that have been appearing, Candida krusei is always resistant to fluconazole and Candida glabrata is often resistant. Upon identification of Candida on Gram stain or culture of PD fluid, initial therapy is usually with fluconazole 200 mg po on day 1 and then 50 to 100 mg po qd or 200 mg IP every 24 to 48 hours (TABLE 14.3) (1). Upon receipt of the species ID and drug susceptibility testing results, if fluconazole resistance is present, therapy may need to be changed to a broader spectrum agent. Itraconazole, voriconazole, posaconazole, and isavuconazole are broader spectrum azole drugs that have better activity on many non-C. albicans species and some molds as well. Of the broader spectrum azoles, voriconazole 2.5 mg/kg IP qd (72) or 200 mg po BID (73) has the best penetration into aqueous compartments and is a potential option for fluconazole-resistant strains. Echinocandin antifungal drugs have excellent activity against Candida species, but there is little experience in treating PD peritonitis. A possible concern is that echinocandins are large molecules that are highly protein-bound and do not penetrate other aqueous sites such as cerebrospinal fluid (CSF) or urine well. However, caspofungin 50 mg IV qd has been used successfully in combination with amphotericin B to treat a multiresistant C. albicans (74) and alone IV to treat trichosporon PD peritonitis cases (75). IP administration has not been studied to my knowledge. Amphotericin B is active on most fungal species, including molds. Early attempts at using amphotericin IP failed because of severe irritant peritonitis, even at low doses. Amphotericin B given IV penetrates the peritoneal fluid poorly but has been used successfully to treat Candida peritonitis IV at a dose of 0.75 to 1 mg/kg/d along with po flucytosine (76). Flucytosine, 1 g po qd, is recommended to be used in combination with an azole or amphotericin B by the ISPD for the treatment of Candida peritonitis (1). If used, flucytosine serum levels should be measured, maintaining trough levels less than 25 to 50 μg/mL to avoid excessive levels and hematologic and GI toxicity. Most published series of Candida peritonitis (77) and ISPD guidelines (1) emphasize the importance of prompt catheter removal. Culture-directed antifungal therapy should be given for at least 2 weeks, followed by catheter replacement after 4 to 6 weeks (71).
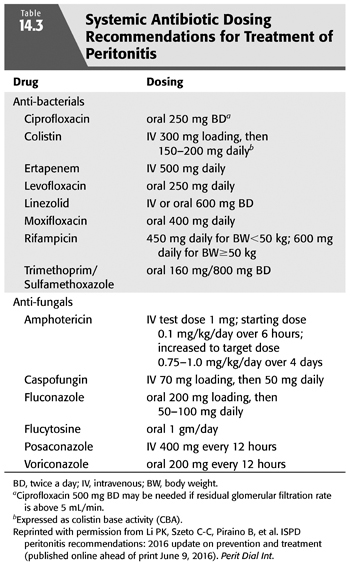
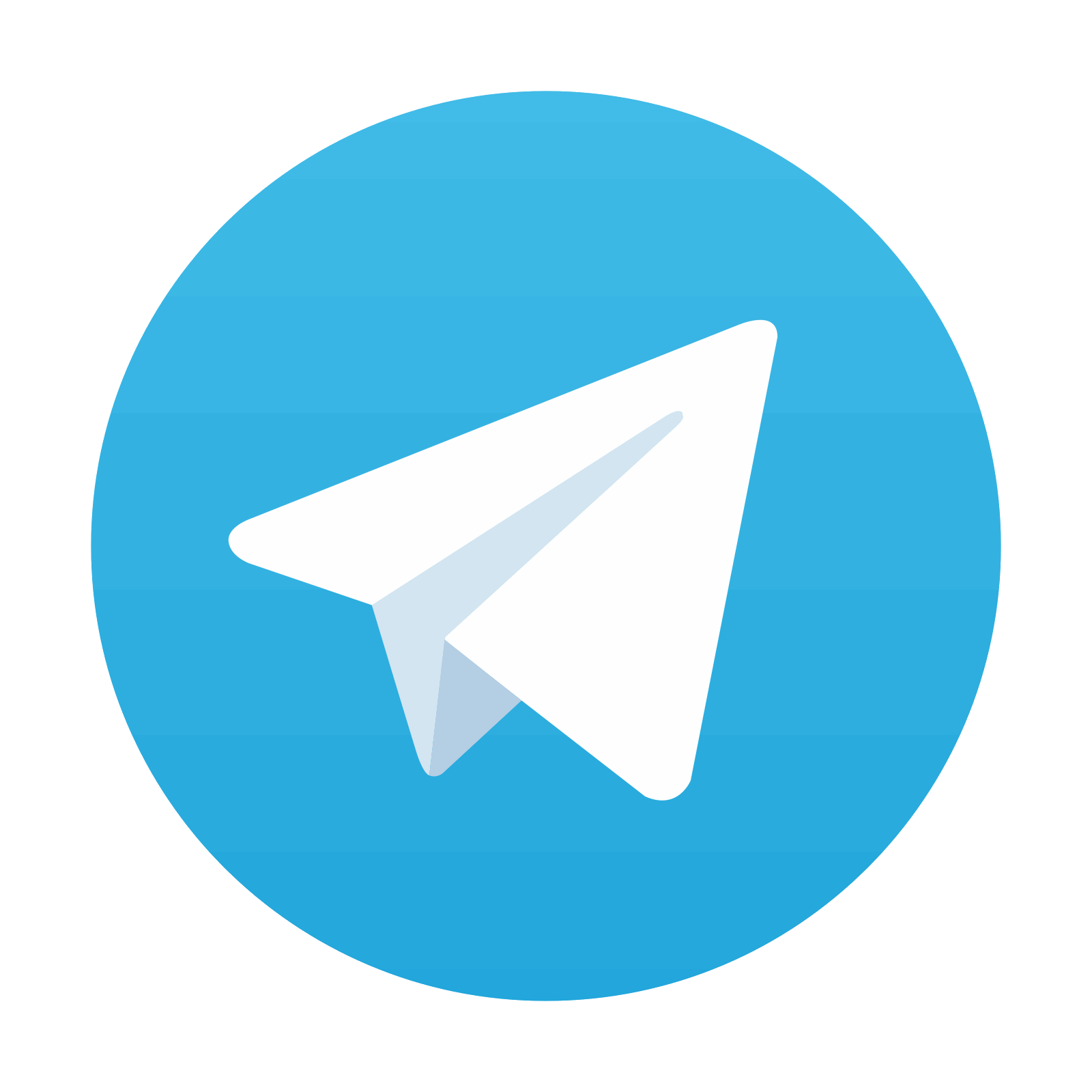
Stay updated, free articles. Join our Telegram channel

Full access? Get Clinical Tree
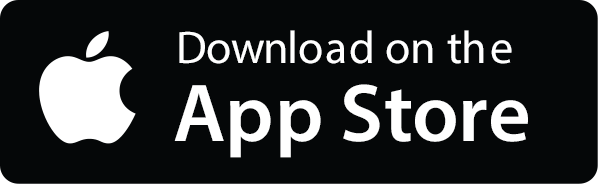
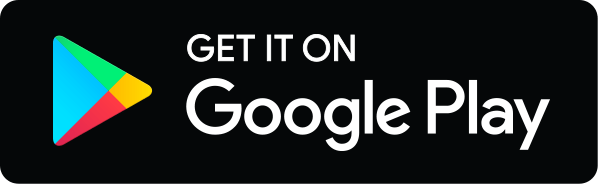