ACUTE KIDNEY INJURY IN CHILDREN
A full picture of the incidence and outcome of AKI in children is made more complicated by the use of varying definitions: the Pediatric Risk, Injury, Failure, Loss, End-Stage Renal Disease (pRIFLE) (5), the AKI network (6), and the Kidney Disease: Improving Global Outcomes (KDIGO) (7) assessments. The incidence of AKI in a large pediatric study varied from 37% to 51% depending upon the definition; capture rates and stages of AKI differed between methods (8). However, AKI, by any definition, was associated with higher mortality and longer length of stay. Furthermore, pediatric intensive care unit (PICU) patients showed increasing mortality with greater severity of AKI irrespective of definition.
The causes of AKI in children differ substantially from those in adults and between industrialized and developing countries (9,10). Common causes of AKI in referral centers in industrialized countries include complications of cardiac surgery, treatment of malignancies, and nephrotoxins (11,12). Additional causes common to both settings include hemolytic uremic syndrome, sepsis with multiorgan dysfunction, ischemia, glomerulonephritis, and congenital kidney disease (9,13,14). In a larger referral center, two decades of observation showed neonates and infants were commonly affected and had higher mortality than older children (15). Several comprehensive reviews on pediatric AKI are available (16–19).
Conservative management of the child with AKI includes careful fluid resuscitation, if intravascular volume depletion is present. Once euvolemia has been restored, if oligoanuria is present, maintenance of fluid balance is attempted through the use of diuretics and fluid restriction. Additional measures include diet modification (restriction of potassium and phosphorus, using specialized formula in infants) to minimize development of metabolic disturbances and the use of medications (sodium polystyrene, sodium bicarbonate, and phosphate binders) to correct electrolyte disturbances. Conservative measures, however, are often not sufficient to allow optimal management particularly with prolonged and severe AKI when fluid restriction often compromises the ability to provide appropriate nutrition, which is essential for recovery (20).
INDICATIONS FOR RENAL REPLACEMENT THERAPY
The indications for initiation of RRT in pediatric AKI are similar to those in adults. The indications include (a) oligoanuria requiring fluid and/or electrolyte removal to optimize nutritional and medical support; (b) hypervolemia complicated by congestive heart failure, pulmonary edema, or severe hypertension despite diuretic therapy and fluid restriction; (c) hyperkalemia refractory to medical management or associated with cardiac involvement as evidenced by electrocardiogram changes; (d) metabolic acidosis refractory to medical management with sodium bicarbonate or limited by sodium overload; (e) symptomatic uremia with pericarditis, neuropathy, or encephalopathy; (f) tumor lysis syndrome or severe hyperuricemia; and (g) toxic ingestions (21). Furthermore, RRT in children is used for specific nonkidney disease indications including inborn errors of metabolism with hyperammonemia or unremitting acidosis and toxic ingestions (22–24). These rare conditions require prompt recognition and emergency resuscitation to allow nephrologists to provide rapid, efficient clearance of accumulated metabolites and toxins (see below).
Fluid overload is recognized to be an independent risk factor for mortality in pediatric patients in PICU settings (25,26). Single-center and multicenter retrospective studies have supported the observation that survivors of AKI are more likely to have a lesser degree of volume overload at the time of initiation of CRRT (27–29). This has led some pediatric centers to develop protocols for initiation of CRRT when patients become 10% fluid volume overloaded or at initiation of mechanical ventilation regardless of glomerular filtration rate (GFR) (30–32).
PERITONEAL DIALYSIS
PD offers several advantages for the care of the pediatric patient with AKI. Technically, it is a simple procedure that does not require specialized personnel. Nurses in PICUs can be trained to perform the procedure with an acceptably low infection rate. Currently available automated cycler devices permit frequent dialysis exchanges without repeatedly opening the circuit, further lowering the infection risk. Fluid and solute removal occur gradually, making the procedure well tolerated in the hemodynamically compromised child and eliminating the risk of hypotension or dialysis disequilibrium (33–35). From a practical standpoint, placement of a PD catheter is technically easier than placement of vascular access, which can be particularly challenging in the small infant (36) (TABLE 38.1).
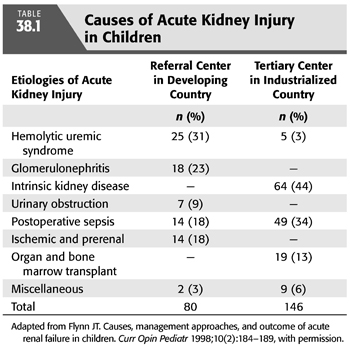
PD provides particularly efficient solute and fluid removal in the youngest patients. Peritoneal membrane surface area correlates with body surface area rather than with body mass; this ratio is most favorable in infants and young children who can thus achieve large peritoneal clearance (37,38). Use of PD also avoids the need for anticoagulation and blood exposure through priming of a blood circuit. Because PD is the preferred mode of dialysis for children with chronic kidney disease, initiating the therapy in the acute setting can facilitate the transition to chronic dialysis. This form of dialysis is less expensive to perform and requires a smaller initial capital investment than other CRRTs (see subsequent text) (1).
PD has been used to manage volume overload and AKI after cardiac surgery in infants with some success (39–41). However, not all studies demonstrate benefit from PD or superiority over CRRT (42,43).
PD, however, is not appropriate for all patients. It is contraindicated in those with abdominal wall defects (e.g., bladder exstrophy, omphalocele, and gastroschisis) and diaphragmatic lesions (e.g., diaphragmatic hernia and surgical defects). It cannot be used immediately after abdominal surgery. The presence of a ventriculoperitoneal shunt is a relative contraindication because of the risk of ascending infection should peritonitis develop; many pediatric nephrologists choose another modality in that setting. PD may not be successful after extensive abdominal surgery because adhesions can cause failure of dialysate drainage, which is manifested as slow outflow rates or poor ultrafiltration.
In clinical situations in which rapid removal of solute (e.g., hyperkalemia), toxin (ingestion), or metabolite (e.g., ammonia) is required, PD does not provide an optimal response. The gradual nature of the treatment, which is advantageous in uremia, will limit the rapid response those emergencies require. Furthermore, in states of acute volume overload with pulmonary edema or congestive heart failure, the ultrafiltration provided by PD may not be rapid or predictable enough to prevent clinical deterioration or mortality (42). Nonetheless, in life-threatening emergencies, PD may be the most accessible and familiar dialysis modality available and can be used until alternate modalities can be arranged (24,44).
Peritoneal Dialysis Catheters
Neonatal and pediatric sizes of most adult catheter configurations are available. These include acute “temporary” catheters and chronic catheters appropriate for operative placement. Acute catheters can be placed percutaneously at the bedside after filling the abdomen with dialysate, as is done in adults (36,45). This permits dialysis therapy to be initiated quickly even in patients too unstable for surgery. Before placement of the dialysis catheter, a Foley catheter should be inserted to empty the bladder and decrease the risk of bladder perforation.
Temporary catheters have several disadvantages: Percutaneous placement can result in injury to an abdominal viscus; bowel and bladder perforations are recognized complications (46). Older catheters were stiff, and even after successful placement, they could cause bowel injury; this often necessitated immobilizing the child while the catheter was in place. Newer more flexible catheters perform better (45). In addition, temporary catheters are uncuffed and, therefore, carry a much greater risk of dialysate leakage at the exit site and subsequent infection. The risk of catheter complications increases significantly after 6 days of use, and it is usually impossible to predict renal recovery at the initiation of PD (47). Therefore, many nephrologists prefer an operatively placed catheter that is used immediately.
The Tenckhoff catheter, although designed as a chronic catheter, is often used in the acute setting (47,48). The catheter can be placed in the intensive care unit or in an operating suite; laparoscopic placement may have advantages (49). There is an increased risk of leakage at the exit site when the catheter is used immediately after placement, and strategies to limit that complication are described in the subsequent text.
Data from the North American Pediatric Renal Transplant Cooperative Study (NAPRTCS) do not suggest that one type of chronic catheter design is superior to the others. However, data do suggest a downward (caudally) oriented exit site is preferable because this has been associated with a reduced risk of peritonitis in chronic dialysis (50,51). In infants, it is preferable to position the exit site above the diaper area to reduce the risk of fecal contamination. Similarly, in children with ostomies, it is preferred that the dialysis catheter be positioned on the contralateral side to maximize the distance between the ostomy and the catheter exit site, thereby minimizing the risk of exit-site contamination and infection. Omental obstruction resulting in poor outflow is common especially in small children; therefore, many advocate performing an omentectomy at the time of the original surgery to avoid outflow obstruction (52–55). This issue, however, remains controversial because other authors do not believe that it is necessary (56). Intraoperatively, should any hernial defects be noted, they should be repaired to minimize this potential complication of PD.
Peritoneal Dialysis Solutions
Acute pediatric PD is usually performed using the commercially available lactate-based dialysis solution. Calcium concentrations (1.25 and 1.75 mM) are chosen based on the need for calcium-based phosphorus binders and vitamin D supplements, but that choice may be less relevant in the acute setting. Lactate-based dextrose solutions have the disadvantages of low pH and glucose absorption with prolonged dwells. Low pH can contribute to pain on inflow and impaired phagocytic activity resulting in increased risk for infection (57). More biocompatible PD solutions, such as neutral-pH low-glucose degradation product solution (58) and bicarbonate-based solution (59), have been tested in children. Short-term and intermediate-term safety has been demonstrated in chronic PD. A comparison of bicarbonate and lactate PD solution in adults with AKI showed improved serum bicarbonate, lactate, and pH with the former solution (60). There are no similar data in children.
Because peritoneal surface area in children is large relative to body surface area, glucose absorption during PD can, unfortunately, prove to be too efficient (38). Such glucose absorption during prolonged dwells dissipates the osmotic gradient between plasma water and dialysate leading to suboptimal ultrafiltration, hyperglycemia, hyperinsulinemia, and hyperlipidemia (61,62). Short dwells are used to limit glucose absorption. Alternative osmotic agents such as amino acids may prove useful, at least permitting nutritional benefit from the enhanced absorption. Combined amino acid–glucose-based dialysate has been used in children for acute PD (63). There are no data on icodextrin use in acute PD in children, although it has been studied in chronic PD patients (64).
Dialysis Prescription Considerations
Acute PD is usually initiated with small volumes to limit leakage around a new catheter. Small fill volumes of approximately 10 to 20 mL/kg are often the starting range, although poor drainage, slow clearance, and inadequate ultrafiltration may limit the effectiveness of low-volume therapy. Fill volumes are gradually increased over many days to a goal of 40 to 50 mL/kg, as tolerated. Short exchange times (45 to 90 minutes) may be used to overcome the limitations of low fill volume and to facilitate ultrafiltration by limiting glucose absorption (65). Even shorter dwell times are possible; however, they are less efficient because a larger proportion of time is spent filling and draining leaving less time for actual dialysis (66).
Although efficient peritoneal glucose absorption may necessitate high dextrose concentrations for infants and small children to achieve ultrafiltration with long dwell times, short exchanges usually provide acceptable or even excessive ultrafiltration using 1.5% dextrose. Therefore, frequent dialysis exchanges for adequate clearance may result in unpredictable fluid removal in small children; careful reassessment of volume status and supplemental fluid, either enteral or parenteral, must be provided to prevent intravascular volume depletion that, in turn, may impair renal recovery.
PD can be performed manually or with an automated cycler. Low-volume manual PD exchanges use a Y-type connector, one limb of which is attached to the inflow line and dialysate bag and the other limb is attached to the drain line and bag to avoid repeatedly opening the catheter connection. A commercially available product (Gesco Dialy-Nate Set) is available as a closed system which permits repeated small exchanges by using an in-line graduated measuring device inserted between the dialysate bag and inflow tubing; this allows more precise measurement of fill volumes and infrequent opening of the dialysis circuit to limit potential contamination. An automated cycling PD machine can be programmed for short dwell treatments appropriate for acute PD; this method also limits opening the catheter circuit to once a day, which decreases the risk of contamination. Currently available cyclers that permit very low volume exchanges (beginning at 60 mL) and low-volume tubing (to decrease the dead space or recirculation volume) facilitate therapy in infants and small children.
Complications of Peritoneal Dialysis
The most common complications of acute PD are catheter malfunction and infection. Catheter malfunction includes dialysate leakage, inflow problems, and outflow problems. Leakage can be external, occurring around the exit site or the incision used to insert the catheter, or internal, resulting in a hernia. Risk factors for external leaks include use of a stiff temporary catheter, frequent catheter manipulation, malnourishment, and initiation of PD with large fill volumes immediately after catheter placement. Strategies to minimize the risk of leakage include use of small fill volumes, minimal catheter manipulation, and two purse-string sutures to seal the peritoneum around the catheter and to seal the posterior rectus sheath opening (67). Temporary discontinuation of PD and use of smaller fill volumes is the initial approach to catheter leaks, but surgical repair is sometimes required. Three retrospective reviews of pediatric acute PD found an increased rate of complications with temporary catheters compared with Tenckhoff catheters (1,47,68).
Obstruction to dialysate flow or excessively slow flow is a frequent catheter complication. Inflow obstruction is usually due to a mechanical blockage: a kink in the catheter, clamp on the catheter, or the presence of blood clot or fibrin. Addition of heparin to the dialysate at a concentration of 250 to 1,000 U/L may diminish fibrin and blood clots. Outflow obstruction is more common and is usually a greater impediment to successful therapy. Catheter entrapment or occlusion by omentum can limit flow and may require reoperation for omentectomy; many surgeons, therefore, perform this at the time of catheter placement. Intra-abdominal adhesions may prevent free flow of dialysate throughout the abdomen, and poor outflow will be noted. Catheter migration can occur and result in painful inflow of dialysate and poor outflow; this may be correctable using a stylet under fluoroscopy or it may require laparoscopic repositioning. Constipation and intestinal distention often limit outflow and should be managed with stool softeners, enemas, or laxatives (avoiding magnesium and phosphorus) (69).
Infectious complications may involve the exit site, tunnel, and/or peritoneum. In the acute setting, an exit-site infection is essentially a surgical wound infection and should be managed as such with parenteral antibiotics. The risk for dialysate leakage and contamination of the peritoneal space is high. Peritonitis is a serious complication of acute PD. It presents a large inflammatory burden to already debilitated, catabolic patients. Resistant organisms and fungi are a greater risk in the intensive care setting where patients are often already receiving antibiotic therapy. Frequent surveillance cell counts and cultures are advisable in this setting because the typical features of fever, abdominal pain, and cloudy effluent may be difficult to discern. Empiric broad-spectrum antibiotic therapy is often required until culture results are available. Intravenous or intraperitoneal therapies are appropriate, depending on the severity of the infection; combined therapy has been used in debilitated patients. Risk factors for developing peritonitis include use of a temporary catheter for more than 3 days, leakage around the exit site, age younger than 2 years, and poor dialysis technique (46).
Hernias are more often a complication of chronic PD, resulting from dialysis performed in an upright posture and increased intra-abdominal pressure. However, a diaphragmatic defect (pleuroperitoneal fistula) can result in hydrothorax even at initiation of dialysis, compromising ventilation and preventing adequate dialysis drainage. A patent processus vaginalis can cause a hydrocele or genital edema. Although these hernias could be corrected surgically, in the acute setting, one is more likely to turn to an alternate modality of dialysis.
Acute PD can cause metabolic complications, most often resulting from glucose absorption. Hyperglycemia may occur and require insulin therapy. Hypertriglyceridemia can result from glucose absorption and be difficult to distinguish from the effects of hyperalimentation. There is spontaneous loss of albumin into dialysate, which can cause hypoalbuminemia; this loss is dramatically increased if peritonitis complicates therapy (46). Lactic acidosis secondary to lactate absorption from the dialysate can occur in small children, but most patients see an improvement in acidosis with initiation of PD. Hyponatremia is common, particularly in very young patients. It is exacerbated by the administration of hypotonic fluids; hypernatremia can develop with excessive ultrafiltration and insufficient free water intake.
HEMODIALYSIS
Acute HD can be safely and effectively performed in infants and children of all sizes (70–73). It requires highly trained personnel, specialized equipment, and a well-functioning vascular access. Acute HD is often preferred in situations requiring rapid removal of fluid, solute, or toxins (e.g., hyperammonemic coma or other inborn errors of metabolism, ingestion, or hyperkalemia) (21,22,74–77). In fact, HD treatments in small children can be strikingly efficient because body water space (V) is small relative to the potential clearance that one can provide with standard or high-flux dialyzers and typical blood flows (QB) (78). Improvements in HD machinery and availability of size-appropriate equipment (blood lines, dialyzers, and vascular access) have facilitated the use of HD in infants and small children. However, the ability to perform HD is contingent on vascular access, which is a challenge in infants and small children.
Technical Considerations
Although the principles of HD are the same in children as in adults, there are several technical aspects unique to children.
Personnel
Skilled dialysis nurses with pediatric experience are required to perform acute HD in children. A nurse-to-patient ratio of 1:1 is needed to provide focused continuous attention to small patients. Keen observation skills and an awareness of age-dependent norms for vital signs are necessary to assess pediatric HD patients and intervene appropriately. Children who are ill are often unable to communicate their distress verbally. Warning signs of decompensation such as agitation or poor perfusion must be recognized quickly. Normal blood pressure in small children is much lower than in adults, leaving little margin for error and hypotension may develop precipitously (79).
Hemodialysis Machines
Technologic improvements in the design of HD machines that have benefited children include the incorporation of volumetric ultrafiltration controllers and blood pumps capable of being calibrated for neonatal, infant, and pediatric blood lines. The blood pump must be able to accurately deliver blood-flow rates within the range 20 to 300 mL/min, appropriate for neonates through older adolescents. The presence of an accurate volumetric ultrafiltration controller is also essential because even small errors in ultrafiltration volume of a few hundred milliliters can result in symptomatic fluid overload or intravascular volume depletion and hypotension.
Extracorporeal Circuit—Blood Lines
In infants and small children, the extracorporeal circuit volume may represent a significant fraction of total blood volume, and severe hypotension can occur at initiation of the treatment. The typical extracorporeal circuit for adult patients may exceed 150 mL; neonatal, infant, and pediatric blood lines are available to limit the circuit volume for some manufacturers. Low demand has caused many manufacturers to withdraw pediatric-specific dialysis supplies. In some cases, the blood pump must be calibrated for the chosen blood line for accurate blood flow. Neonatal lines may not be compatible with available volumetric dialysis machines. However, even using low-volume bloodlines and dialyzers, the total circuit volume cannot always be reduced to less than 10% of the patient’s blood volume, particularly in the case of newborns and small infants. When the circuit volume exceeds 10% of the patient’s blood volume or when the patient is severely anemic, the circuit can be primed with blood to maintain hemodynamic stability (71). Packed red blood cells may be diluted 1:1 with normal saline to decrease the hematocrit to approximately 35%, thereby decreasing the viscosity and risk for clotting. Blood priming carries its own particular risks. The greatest concern is that of potential antigen exposure, which will complicate future renal transplantation in patients who do not regain renal function. The risk of sensitization to antigens is multiplied by the number of HD treatments required, because each will require a separate blood prime. In addition, a blood prime is a potential infectious exposure, and young children may acquire cytomegalovirus infection as a result. Furthermore, even if the primed blood circuit is infused at a low blood-flow rate (20 to 50 mL/min), it represents a rapid rate of blood transfusion. This may result in a transfusion reaction or hypocalcemia from citrate infusion. Finally, the potassium load associated with transfusion of packed red blood cells may produce sudden hyperkalemia with cardiac arrhythmias, which may not be corrected quickly enough by HD. This risk is diminished by washing packed red blood cells before the procedure. At the end of an HD treatment which began with a blood prime, the blood circuit is not returned to the patient.
Dialyzers
A wide variety of dialyzers have been used in children; no pediatric data suggest an advantage of one type of membrane. Extrapolating from studies in adults suggesting a benefit of biocompatible membranes on survival and recovery of AKI (80–82), these dialyzers have become standard in children. A more significant challenge is finding dialyzers of sufficiently small volume, surface area, and ultrafiltration coefficient (Kuf). Choices in dialyzers with surface area less than 0.4 m2 are limited, and availability changes often. Small priming volume is an advantage when dialyzing infants and small children because it may allow one to avoid priming the circuit with blood. Dialyzers with relatively large surface area may be advantageous when rapid clearance is needed, but in most cases, clearance will be determined by the blood flow that one can achieve throughout the treatment, rather than the surface area or KoA of the dialyzer. In practice, all standard and high-flux dialyzers have a higher Kuf than is needed for adequate ultrafiltration in small children. Earlier, this was a great concern because small errors in setting the dialysis machine transmembrane pressure (TMP) could result in large ultrafiltration errors and hypotension. In addition, because pediatric vascular access is generally of small caliber, one finds high venous pressures that, in turn, result in greater TMP. This concern has been alleviated by the widespread use of machines with volumetric ultrafiltration controllers. However, during isovolemic HD (or even during modest ultrafiltration) with a high-flux dialyzer, backfiltration of dialysate is to be anticipated; this can be avoided by increasing the ultrafiltration rate sufficient to reverse negative TMP and infusing saline through the treatment (TABLE 38.2).
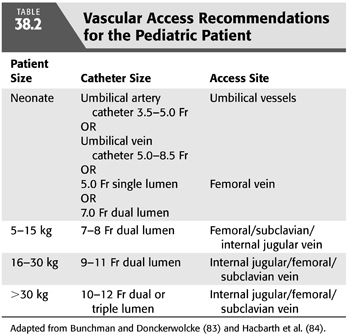
Vascular Access
Obtaining a well-functioning vascular access remains a challenge in small patients. Vascular access for acute HD is usually established by percutaneous placement of a double-lumen catheter. Whether a temporary or permanent (tunneled) dialysis catheter is chosen depends on one’s estimate of the duration of dialysis therapy needed. This is often merely an approximation; patients typically begin urgent dialysis therapy with a temporary catheter, and a tunneled catheter is placed electively. Double-lumen catheters are available in sizes from 7 Fr to 14 Fr and come in various lengths; manufacturers and availability of the smallest catheters change frequently. Single-lumen catheters of 5 Fr and smaller are used for neonates. TABLE 38.2 provides guidelines for catheter selection based on patient size. Ideally, the catheter should offer low resistance to blood flow and, therefore, should be a stiff catheter with a short length but large internal diameter (85). Broviac catheters, often already placed in oncology patients, are inappropriate because of their flexibility, length, and small lumen size. Acute dialysis catheters can be placed in the femoral vein, subclavian vein, or internal jugular vein; the choice depends on the size of the patient and the availability of central venous sites. Femoral catheters are technically easier to insert and are not associated with the risks of pneumothorax and pneumomediastinum or hemothorax and hemomediastinum. However, there is an increased risk of infection when left in place for more than a few days and patients are confined to bed. Catheters placed in the subclavian vein or internal jugular vein should have their tips positioned at the junction of the superior vena cava and right atrium to allow adequate blood flow and minimize recirculation. They may be left in place for several weeks, although a tunneled catheter would be a better choice for such a duration of dialysis.
In an infant, vessel size may make placement of even a small-caliber double-lumen catheter impossible, and one may be obliged to place single-lumen catheters in two sites. In the newborn, HD can be performed through small single-lumen catheters placed in the umbilical artery and vein. Small-caliber catheters increase the risk of hemolysis from sheer stress and can cause wide swings in access pressures affecting ultrafiltration and compromising the overall success of the treatment.
Dialysis Prescription
There are no prospective trials to assist clinicians in determining the adequacy of acute HD therapy in children with AKI. Because the spectrum of diseases causing AKI is different from that in adults, extrapolation from that literature may be difficult. Therefore, the duration, frequency, and efficiency of the HD treatments are a matter of judgment aided by an understanding of kinetic modeling and of the modifiable variables (blood flow, dialysate flow). Often, a single metabolic derangement (e.g., intoxication, hyperammonemia) defines the length and efficiency of the treatment. Nonetheless, when children are dialyzed for advanced uremia with standard dialyzers, the urea clearances that are achieved may be sufficient to cause true disequilibrium or seizures (which are a more common complication in children than in adults) (86,87). Blood-flow rate for the first few treatments may be decreased to target urea clearance of 2 to 3 mL/kg/min, and treatment length will usually be shortened to 1.5 to 2 hours to avoid precipitous falls in blood urea nitrogen (BUN) (86). Single-pool Kt/V for a first treatment in a uremic child should probably not exceed 0.6. Short daily treatments are often the most appropriate way to initiate HD therapy without patient discomfort or instability. Subsequent treatments are lengthened to 3 to 4 hours or longer if needed, and higher urea clearance rates can be targeted (blood-flow rates of 4 to 5 mL/kg/min). Smaller blood vessels and catheters cause higher venous resistance than in adults, and this will eventually limit blood flow, perhaps as low as 25 to 100 mL/min.
Acute HD treatments in children should only be performed with bicarbonate-based dialysate. Otherwise, small patients will be presented with a disproportionately large acetate load, and their small muscle mass will be inadequate to metabolize it. Dialysate flow rates range from 300 to 900 mL/min, as in adults. For treatments using low blood flows and dialyzers of low KoA, dialysate flow rates are not rate limiting for clearance and the lower range will be sufficient.
Ultrafiltration is targeted to the patient’s clinical situation. If severe volume overload is present, rapid ultrafiltration may be appropriate. Otherwise, one attempts to limit total fluid removal per session to 5% of the patient’s body weight (71). Critically ill patients in intensive care units often require large volumes of medications, feedings, and blood products resulting in ongoing fluid overload and hemodynamic instability with HD treatments. Close monitoring of blood pressure, ideally by an arterial line, is required. If isolated ultrafiltration without dialysate is planned in an infant or small child, hypothermia may develop because the dialysis circuit acts as a radiator that is relatively large for the patient’s total blood volume.
Some form of anticoagulation is generally needed when performing HD in children particularly because the blood-flow rates are often slow, increasing the risk of clotting. Systemic heparinization is used most commonly with doses scaled to body size and then adjusted according to activated clotting times (ACTs). A loading dose of 10 to 30 U/kg is given at the start followed by a maintenance dose of 10 to 20 U/kg/hr. ACTs are followed at the bedside when machines and trained personnel are available, and the heparin dose is titrated to keep the ACT between 150 and 200 seconds (88).
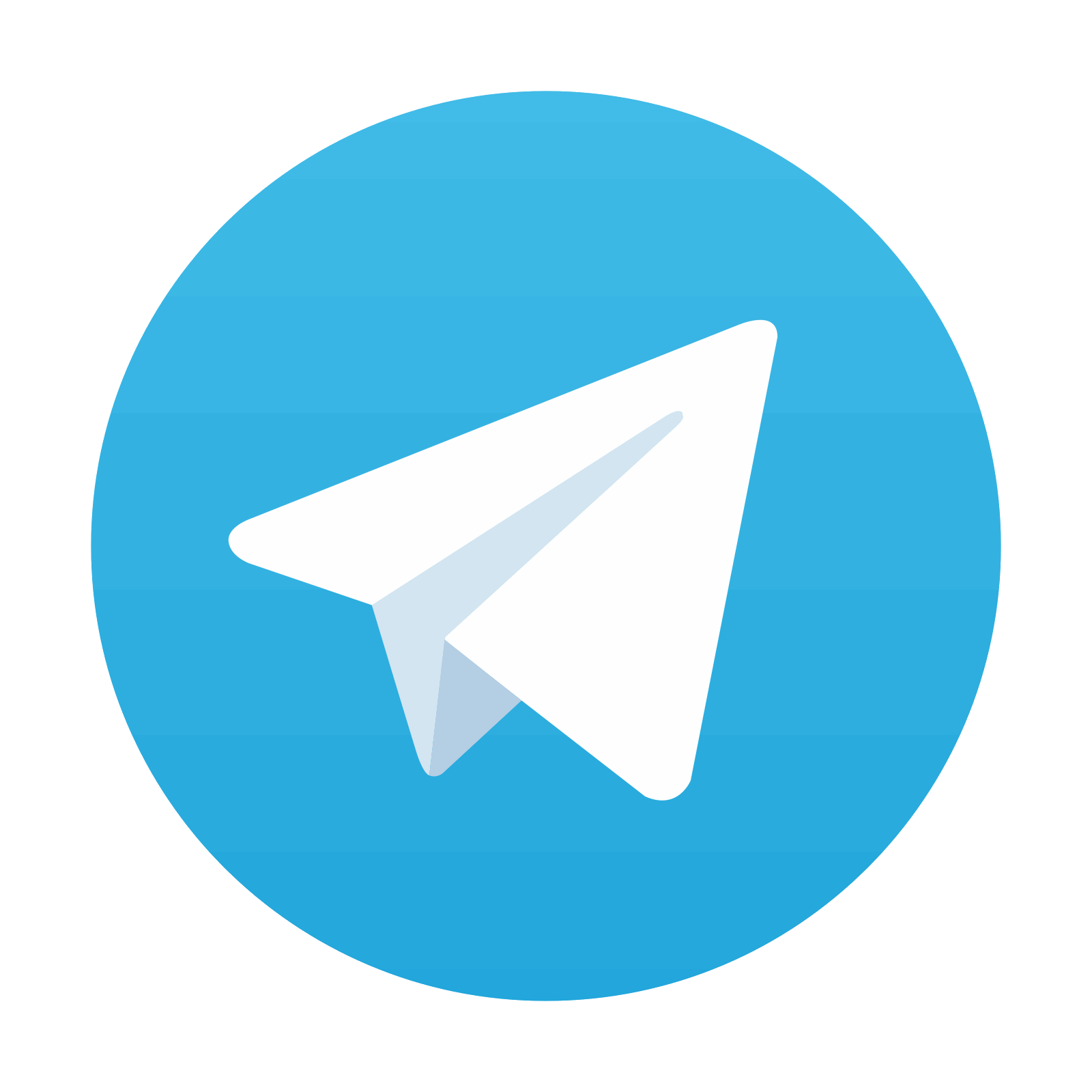
Stay updated, free articles. Join our Telegram channel

Full access? Get Clinical Tree
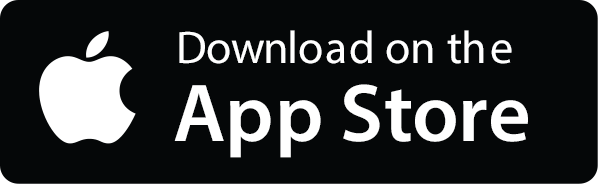
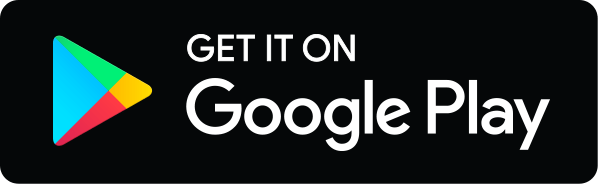