Neuropathic Bladder
Innervation of the lower urinary tract (LUT)
Motor innervation of the bladder
Parasympathetic motor innervation of the bladder
Preganglionic, parasympathetic nerve cell bodies are located in the intermediolateral column of spinal segments S2-4. These preganglionic, parasympathetic fibres pass out of the spinal cord through the anterior primary rami of S2, S3, and S4 and contained within nerves called the nervi erigentes, they head towards the pelvic plexus. In the pelvic plexus (in front of the piriformis muscle), the preganglionic, parasympathetic fibres synapse within ganglia with the cell bodies of the post-ganglionic parasympathetic nerves which then run to the bladder and urethra. Fifty percent of the ganglia of the pelvic plexus lie in the adventitia of the bladder and bladder base (the connective tissue surrounding the bladder) and 50% are within the bladder wall. The post-ganglionic axons provide cholinergic excitatory input to the smooth muscle of the bladder.
Sympathetic motor innervation of the bladder
In the male, preganglionic sympathetic nerve fibres arise from the intermediolateral column of T10-12 and L1-2. These preganglionic neurons synapse in the sympathetic chain and post-ganglionic sympathetic nerve fibres travel as the hypogastric nerves to innervate the trigone, blood vessels of the bladder, and the smooth muscle of the prostate and preprostatic sphincter (i.e. the bladder neck). In the female, there is sparse sympathetic innervation of the bladder neck and urethra.
In both sexes, some post-ganglionic sympathetic nerves also terminate in parasympathetic ganglia (in the adventitia surrounding the bladder and within the bladder wall) and exert an inhibitory effect on bladder smooth muscle contraction.
Afferent innervation of the bladder
Afferent nerves from receptors throughout the bladder ascend with para sympathetic neurons back to the cord and from there, up to the pontine storage and micturition centres or to the cerebral cortex. They sense bladder filling.
Other receptors are located in the trigone and afferent neurons from these neurons ascend with sympathetic neurons up to the thoracolumbar cord and thence to the pons and cerebral cortex.
Other receptors are located in the urethra. The afferent neurons pass through the pudendal nerve and again ascend to the pons and cerebral cortex. All these neurons have local relays in the cord.
Somatic motor innervation of the urethral sphincter: the distal urethral sphincter mechanism
Anatomically, this is located slightly distal to the apex of the prostate in the male (between the verumontanum and proximal bulbar urethra) and in the mid-urethra in the female. It has three components:
Extrinsic skeletal muscle: this is the outermost layer, the pubourethral sling (part of levator ani). Composed of striated muscle and
innervated by the pudendal nerve (spinal segments S2-4, somatic nerve fibres). It is activated under conditions of stress and augments urethral occlusion pressure.
Smooth muscle within the wall of the urethra: cholinergic innervation. Tonically active. Relaxed by nitric oxide (NO).
Intrinsic striated muscle (i.e. skeletal muscle within the wall of the urethra, hence known as the ‘intrinsic rhabdosphincter’): it forms a ‘U’ shape around the urethra and around the anterior and lateral aspects of the membranous urethra and is absent posteriorly (i.e. it does not completely encircle the membranous urethra). It may produce urethral occlusion by kinking the urethra rather than by circumferential compression.
Preganglionic somatic nerve fibres (i.e. neurons which innervate striated muscle) are, along with parasympathetic nerve fibres (which innervate the bladder), derived from spinal segments S2-4, specifically from Onuf’s nucleus (also known as spinal nucleus X) which lies in the medial part of the anterior horn of the spinal cord. (Onuf’s nucleus is the location of the cell bodies of somatic motoneurons that provide motor input to the striated muscle of the pelvic floor—the external urethral and anal sphincters.) These somatomotor nerves travel to the rhabdosphincter via the perineal branch of the pudendal nerve (documented by direct stimulation studies and horseradish peroxidase (HRP) tracing—accumulates in Onuf’s nucleus following injection into either the pudendal or pelvic nerves). There also seems to be some innervation to the rhabdosphincter from branches of the pelvic plexus (specifically the inferior hypogastric plexus) via pelvic nerves. In dogs, complete silence of the rhabdosphincter is seen only if both the pudendal and pelvic efferents are sectioned. Thus, pudendal nerve block or pudendal neurectomy does not cause incontinence.
The nerve fibres that pass distally to the distal sphincter mechanism are located in a dorsolateral position (5 and 7 o’clock). More distally, they adopt a more lateral position.
Sensory innervation of the urethra
Afferent neurons from the urethra travel in the pudendal nerve. Their cell bodies lie in the dorsal root ganglia and they terminate in the dorsal horn of the spinal cord at S2-4, connecting with neurons that relay sensory information to the brainstem and cerebral cortex.
The pudendal nerve (a somatic nerve derived from spinal segments S2-4) innervates striated muscle of the pelvic floor (levator ani, i.e. the pubo-urethral sling). Bilateral pudendal nerve block1 does not lead to incontinence because of maintenance of internal (sympathetic innervation) and external sphincter function (somatic innervation, S2-4, nerve fibres travelling to the external sphincter alongside parasympathetic neurons in the nervi erigentes).
Clinical consequences of damage to the nerves innervating the LUT
Bladder neck function in the female
About 75% of continent young women and 50% of perimenopausal continent women have a closed bladder neck during the bladder filling phase. Twenty-five percent of continent young women and 50% of perimenopausal continent women have an open bladder neck and yet they remain continent (because of their functioning distal sphincter mechanism, the external sphincter).2, 3 Presacral neurectomy (to destroy afferent pain pathways) does not lead to incontinence because of maintenance of the somatic innervation of the external sphincter.
Sympathetic motor innervation of the bladder
Division of the hypogastric plexus of nerves during a retroperitoneal lymph node dissection for metastatic testis tumours results in paralysis of the bladder neck. This is of significance during ejaculation where normally sympathetic activity results in closure of the bladder neck so that the ejaculate is directed distally into the posterior and then anterior urethra. If the bladder neck is incompetent, the patient develops retrograde ejaculation; they remain continent of urine because the distal urethral sphincter remains functional, being innervated by somatic neurons from S2-4.
During pelvic fracture, the external sphincter and/or its somatic motor innervation may be damaged such that it is incompetent and unable to maintain continence of urine. Preservation of bladder neck function (the sympathetic innervation of the bladder neck usually remains intact) can preserve continence. However, if in later life, the patient undergoes a TURP or bladder neck incision for symptomatic prostatic obstruction, they may well be rendered incontinent because their one remaining sphincter mechanism (the bladder neck) will be divided during these operations.
1 Brindley GS (1974) The pressure exerted by the external sphincter of the urethra when its motor nerve fibres are stimulated electrically. Br J Urol 46:453-62.
2 Chapple CR, Helm CW, Blease S, Milroy EJ, Rickards D, Osborne JL (1989) Asymptomatic bladder neck incompetence in nulliparous females. Br J Urol 64:357-9.
3 Versi E, et al. (1990) Distal urethral compensatory mechanisms in women with an incompetent bladder neck who remain continent and the effect of the menopause. Neurourol Urodyn 9:579-90.
The physiology of urine storage and micturition
Urine storage
During bladder filling, bladder pressure remains low despite a substantial increase in volume. The bladder is thus highly compliant. Its high compliance is partly due to the elastic properties (viscoelasticity) of the connective tissues of the bladder and partly due to the ability of detrusor smooth muscle cells to increase their length without any change in tension. The detrusor is able to do this as a consequence of prevention of transmission of activity from preganglionic parasympathetic neurons to post-ganglionic efferent neurons—a so-called ‘gating’ mechanism within the parasympathetic ganglia. In addition, inhibitory interneuron activity in the spinal cord prevents transmission of afferent activity from sensors of bladder filling.
Micturition
A spino-bulbar-spinal reflex, coordinated in the pontine micturition centre in the brainstem (also known as Barrington’s nucleus or the M region), results in simultaneous detrusor contraction, urethral relaxation, and subsequent micturition. Receptors located in the bladder wall sense increasing tension as the bladder fills (rather than stretch). This information is relayed, by afferent neurons to the dorsal horn of the sacral cord. Neurons project from here to the periaqueductal grey (PAG) matter in the pons. The PAG is thus informed about the state of bladder filling. The PAG and other areas of the brain (limbic system orbitofrontal cortex) input into the pontine micturition centre (PMC) and determine whether it is appropriate to start micturition.
At times when it is appropriate to void, micturition is initiated by relaxation of the external urethral sphincter and pelvic floor. Urine enters the posterior urethra and this, combined with pelvic floor relaxation, activates afferent neurons, which results in stimulation of the PMC (located in the brainstem). Activation of the PMC switches on a detrusor contraction via a direct communication between neurons of the PMC and the cell bodies of parasympathetic, preganglionic motoneurons located in the sacral intermediolateral cell column of S2-4. At the same time that the detrusor contracts, the urethra (the external sphincter) relaxes. The PMC inhibits the somatic motoneurons located in Onuf’s nucleus (the activation of which causes external sphincter contraction) by exciting GABA and glycine-containing inhibitory neurons in the intermediolateral cell column of the sacral cord, which in turn project to the motoneurons in Onuf’s nucleus. In this way, the PMC relaxes the external sphincter.
Micturition is an example of a positive feedback loop, the aim being to maintain bladder contraction until the bladder is empty. As the detrusor contracts, tension in the bladder wall rises. The bladder wall tension receptors are stimulated and the detrusor contraction is driven harder. One of the problems of positive feedback loops is their instability. Several inhibitory pathways exist to stabilize the storage-micturition ‘loop’.
Tension receptors activate bladder afferents, which via the pudendal and hypogastric nerves, inhibit S2-4 parasympathetic motor nerve output. An ongoing detrusor contraction cannot be overridden.
Afferents in the anal and genital regions and in the distribution of the posterior tibial nerve stimulate inhibitory neurons in the sacral cord and these neurons inhibit S2-4 parasympathetic motor nerve output. This pathway can override an ongoing detrusor contraction. It is hypothesized that this system prevents involuntary detrusor contraction during sexual activity, defaecation, and while walking, running, and jumping.
Excitatory neurotransmission in the normal detrusor is exclusively cholinergic and reciprocal relaxation of the urethral sphincter and bladder neck is mediated by NO released from post-ganglionic parasympathetic neurons.
Further reading
De Groat WC (1993) Anatomy and physiology of the lower urinary tract. Urol Clin NA 20: 383-401.
Bladder and sphincter behaviour in the patient with neurological disease
A variety of neurological conditions are associated with abnormal bladder and sphincter function (e.g. SCI, spina bifida (myelomeningocele), MS). The bladder and sphincters of such patients are described as ‘neuropathic’.
They may have abnormal bladder function or abnormal sphincter function or, more usually, both. The bladder may be over- or underactive, as may the sphincter and any combination of bladder and sphincter overor underactivity may coexist. ‘Activity’ here means bladder and sphincter pressure.
In the normal LUT during bladder filling, the detrusor muscle is inactive and the sphincter pressure is high. Bladder pressure is, therefore, low and the high sphincter pressure maintains continence. During voiding, the sphincter relaxes and the detrusor contracts. This leads to a short-lived increase in bladder pressure, sustained until the bladder is completely empty. The detrusor and sphincter thus function in synergy—when the sphincter is active, the detrusor is relaxed (storage phase) and when the detrusor contracts, the sphincter relaxes (voiding phase).
An overactive bladder is one that intermittently contracts during bladder filling so developing high pressures when normally bladder pressure should be low. In between these waves of contraction, bladder pressure returns to normal or near normal levels. In a patient with an underlying neurological problem, bladder overactivity is called detrusor hyperreflexia (DH). In other patients, the bladder wall is stiffer than normal, a condition known as poor compliance. Bladder pressure rises progressively during filling, such bladders being unable to store urine at low pressures. Some patients have a combination of DH and poor compliance. The other end of the spectrum of bladder behaviour is the underactive bladder which is low pressure during filling and voiding. This is called detrusor areflexia.
An overactive sphincter generates high pressure during bladder filling, but it also does so during voiding when normally it should relax. This is known as detrusor-external sphincter dyssynergia (DESD or DSD; Fig. 14.1). During EMG recording, activity in the external sphincter increases during attempted voiding (the external sphincter should normally be ‘quiet’ during voiding; see Fig. 3.16). An underactive sphincter is unable to maintain enough pressure in the face of normal bladder pressures to prevent leakage of urine.
![]() Fig. 14.1 Detrusor-external sphincter dyssynergia (DESD) seen during video cystourethrography. |
The neuropathic lower urinary tract: clinical consequences of storage and emptying problems
Neuropathic patients experience two broad categories of problems— bladder filling and emptying—depending on the balance between bladder and sphincter pressures during filling and emptying. The effects of these bladder filling and emptying problems include incontinence, retention, recurrent UTIs, and renal failure.
High-pressure sphincter
High-pressure bladder
If the bladder is overactive (detrusor hyperreflexia) or poorly compliant, bladder pressures during filling are high. The kidneys have to function against these chronically high pressures. Hydronephrosis develops and ultimately, the kidneys fail (renal failure). At times, the bladder pressure overcomes the sphincter pressure and the patient leaks urine (incontinence). If the sphincter pressure is higher than the bladder pressure during voiding (DSD), bladder emptying is inefficient (retention, recurrent UTIs).
Low-pressure bladder
If the bladder is underactive (detrusor areflexia), pressure during filling is low. The bladder simply fills up—it is unable to generate enough pressure to empty (retention, recurrent UTIs). Urine leaks at times if the bladder pressure becomes higher than the sphincter pressure (incontinence), but this may occur only at very high bladder volumes or not at all.
Low-pressure sphincter
High-pressure bladder
If the detrusor is hyperreflexic or poorly compliant, the bladder will only be able to hold low volumes of urine before leaking (incontinence).
Low-pressure bladder
If the detrusor is areflexic such that it cannot develop high pressures, the patient may be dry for much of the time. They may, however, leak urine (incontinence) when abdominal pressure rises (e.g. when coughing, rising from a seated position, or when transferring to or from a wheelchair). Their low bladder pressure may compromise bladder emptying (recurrent UTIs).
Bladder management techniques for the neuropathic patient
A variety of techniques and procedures are used to treat retention, incontinence, recurrent UTIs, and hydronephrosis in the patient with a neuropathic bladder. Each of the techniques described here can be used for a variety of clinical problems. Thus, a patient with a high-pressure, hyperreflexic bladder that is causing incontinence can be managed with an ISC (with intravesical botox injections, if necessary) or an SPC or by sphincterotomy with condom sheath drainage or by deafferentation combined with a sacral anterior root stimulator (SARS). Precisely which option to choose will depend on the individual patient’s clinical problem, their hand function, their lifestyle, and other ‘personal’ factors such as body image, sexual function, etc. Some patients will opt for an SPC as a simple, generally safe, generally very convenient, and effective form of bladder drainage. Others wish to be free of external appliances and devices because of an understandable desire to look and ‘feel’ normal. They might opt for deafferentation with a SARS.
External sphincterotomy
Deliberate division of the external sphincter to convert the high-pressure, poorly emptying bladder due to DSD to a low-pressure, efficiently emptying bladder. Indications: retention, recurrent UTIs, hydronephrosis.
Techniques
Surgical (with an electrically heated ‘knife’ or laser). Disadvantages: irreversible, post-operative bleeding, septicaemia, and stricture formation.1
Intra-sphincteric botox (botulinum toxin). A minimally invasive and reversible alternative to surgical sphincterotomy. Disadvantage: repeat injection required every 6-12 months; in the authors opinion (based on years of experience of botox and surgical sphincterotomies), probably not as effective at lowering bladder pressure and improving bladder emptying as surgical sphincterotomy (but no trials have compared the two techniques).
Augmentation
Technique of increasing bladder volume to lower pressure by implanting detubularized small bowel into the bivalved bladder (‘clam’ ileocystoplasty) (Fig. 14.2) or by removing a disc of muscle from the dome of the bladder
(auto-augmentation or detrusor myectomy). In the botox era, augmentation is becoming less and less frequently used because repeat (every 6-12 months) botox injections are often all that is required to achieve an acceptable level of continence, but also because of the short- and long-term morbidity of augmentation (bladder stones in 15%, bladder perforation, high grade invasive bladder cancers).4 Indications: incontinence unacceptable to the patient or hydronephrosis despite full conservative therapy (regular ISC combined with anticholinergics and a trial of bladder botox injections).
(auto-augmentation or detrusor myectomy). In the botox era, augmentation is becoming less and less frequently used because repeat (every 6-12 months) botox injections are often all that is required to achieve an acceptable level of continence, but also because of the short- and long-term morbidity of augmentation (bladder stones in 15%, bladder perforation, high grade invasive bladder cancers).4 Indications: incontinence unacceptable to the patient or hydronephrosis despite full conservative therapy (regular ISC combined with anticholinergics and a trial of bladder botox injections).
![]() Fig. 14.2 A ‘clam’ ileocystoplasty. (Reproduced from Reynard, J, Mark, S. et al., Urological Surgery. Oxford University Press, with permission from OUP.) |
Intravesical botlinum toxin injections
Recently, intravesical botulinum toxin type A injections at multiple sites in the bladder every 6-12 months have produced impressive reductions in bladder pressure and increases in volume (bladder capacity), with a low risk of side effects. As a consequence, surgical augmentation is nowadays only rarely done, being reserved for cases where botox has failed to work (where the patient is still wet between passing ISC catheters or where there is persistent hydronephrosis).
Botulinum toxin is a potent neurotoxin produced by the Gram-negative anaerobic bacterium Clostridium botulinum. Of the seven serotypes, only types A (BoNT-A) (‘Botox®’, Allergan, CA in the United States; ‘Dysport®’, Ipsen, Slough, UK) and B (‘Myobloc®’, Elan Pharmaceuticals, NJ, United States) are used clinically. BoNT-A is synthesized as an inactive single chain of 1285 amino acid polypeptide and is activated when cleaved by Clostridial protease into a two-chain polypeptide (50kDa light chain, 100kDa heavy chain).
Botulinum toxin type A (‘Botox®’ or ‘Dysport®’) binds to the SV2 receptor (synaptic vesicle protein) on the presynaptic nerve terminal where it is internalized by endocytosis. It causes proteolysis of the synaptosomal associated protein, SNAP-25, which is one of a group of SNARE proteins—with neuronal cell membranes (BoNT-B cleaves synaptobrevin). Thus, botulinum toxin inhibits neurotransmission at motor cholinergic, noradrenergic, and other (sensory) nerve terminals. It also reduces the expression of the vanilloid receptor, TPRV1, and the purinoreceptor, P2X3, both of which are sensory neuron receptors.
Thus, while we tend to think of botulinum toxin type A as inhibiting neuromuscular nerve transmission and, therefore, as a muscle paralysing agent, it is likely that it also has effects on sensory nerve transmission. BoNT-A inhibits the release of calcitonin gene-related peptide, substance P, glutamate, nerve growth factor, and ATP, which are neurotransmitters involved in pain pathways. In rat pain models, BoNT-A reduces pain behaviour.5, 6 How this effect on sensory nerve function translates into a role, if any, in the management of sensory urological conditions (sensory urgency, interstitial cystitis) remains to be established.
Recovery of neurotransmission requires removal of BoNT and restoration of intact SNARE proteins.
Intravesical botox injections can be administered using a flexible cystoscope (with a flexible injection needle) or a rigid scope. Multiple techniques of injection have been described and all seem to be effective. Some surgeons dilute the botox in 5mL of saline whereas others use the same number of units in 10 or 20mL of saline. Some use ‘Dysport®’ (Ipsen) while
others use ‘Botox®’ (Allergan). Some inject in ten sites, others in 20 sites, while others make 50 injections. Whether one technique or concentration or formulation of botox is superior to any other remains to be established. Precisely where the botox is actually administered (into the detrusor muscle or into the suburothelium) is debatable (the bladder wall is thin).
others use ‘Botox®’ (Allergan). Some inject in ten sites, others in 20 sites, while others make 50 injections. Whether one technique or concentration or formulation of botox is superior to any other remains to be established. Precisely where the botox is actually administered (into the detrusor muscle or into the suburothelium) is debatable (the bladder wall is thin).
For intravesical injection in neuropathic patients (e.g. those with SCI or MS), the author uses a standard dose of 1000 units of Dysport® (though not infrequently between 1000 and 1500 units in those failing to respond for an adequate duration with 1000 units), diluted in 10mL of saline, and injects 0.33mL per site (three doses per 1mL syringe) in approximately 30 sites (roughly 30-35 units per site), using (usually) a flexible cystoscope and flexible injection needle. Some surgeons spare the trigone (i.e. avoid injecting the trigone), the theory (not proven) being that this avoids disrupting the valve mechanism of the VUJ. Other surgeons (the author included) inject in the trigone. Since the trigone has a dense sensory innervation, trigonal injections may (unproven) be more effective for sensory or painful bladder syndromes.
Intravesical botox injections are indicated for hydronephrosis which has failed to respond to increased frequency of ISC combined with oral anticholinergic medication; inter-ISC leakage which has failed to respond to increased frequency of ISC combined with oral anticholinergic medication; urethral leakage in patients with SPC where the leakage is thought to be due to uninhibited bladder contractions.
Outcomes of bladder botulinum toxin injections in the neuropathic patient
Outcomes of bladder botulinum toxin injections in the non-neuropathic patient
In non-neuropathic patients, three randomized placebo-controlled trials have shown that patients treated with 200-300 units of Botox® dissolved in between 2.5 to 20mL of saline and given at 10-20 sites had reduced urinary frequency and on average 3.88 fewer incontinence episodes per day.9 The botox took effect within 3-14 days and lasted for a median of 307 days.
What dose of bladder botulinum should be used?
There have been few studies to determine the ‘correct’ dose of botox either in neuropaths or non-neuropaths, e.g. the minimal effective dose or the dose giving an adequate duration of effect (balanced against a low frequency of side effects). For the patient who has to change their clothes several times a day, knowledge of the undoubted efficacy of intravesical botox injections makes it is difficult to decide to enter a placebo-controlled study or one where a low, possibly ineffective, dose might be used.
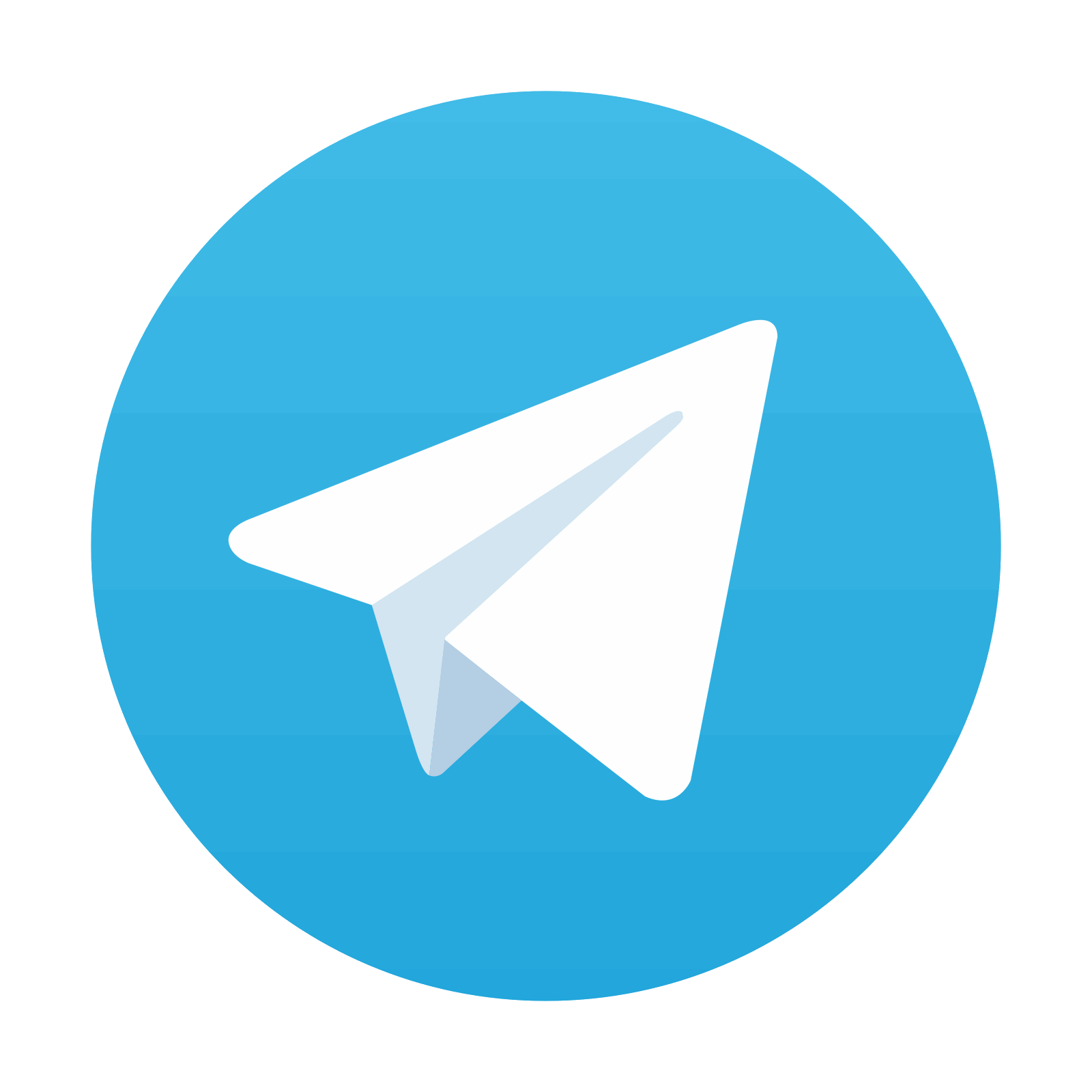
Stay updated, free articles. Join our Telegram channel

Full access? Get Clinical Tree
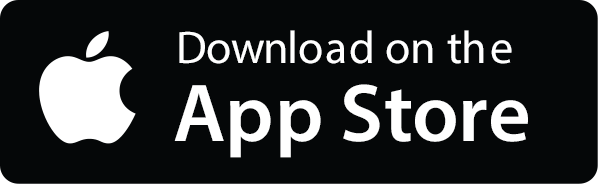
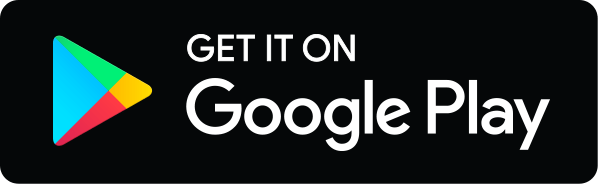
