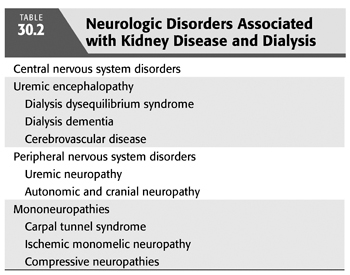
This chapter is divided into two sections. The first discusses central nervous system disorders in patients with CKD and dialysis patients, and the second addresses the peripheral nervous system in patients with CKD and in dialysis patients.
CENTRAL NERVOUS SYSTEM ABNORMALITIES
Uremic Encephalopathy
CKD may result in cognitive impairment associated with clouding of consciousness, especially when the glomerular filtration rate (GFR) falls below 10 mL/min (4). The clinical features of uremic encephalopathy are nonspecific and include confusion, psychomotor agitation, alteration of sleep–wake cycle, disorientation, impaired memory, inattention, paranoid ideation, impaired abstraction, visual hallucinations, myoclonus, and seizures (4–8). The clinical course is usually insidious in onset with waxing and waning of intellectual function, a typical feature of most metabolic encephalopathies.
Progression of renal insufficiency may subsequently result in gradual obtundation followed by coma unless dialysis is performed. Dialysis initiation usually results in gradual clinical improvement (4).
There is no absolute correlation between neurologic impairment and the blood urea nitrogen level, although it has been suggested that the rate of rise of urea and creatinine may be related to the development of neurologic symptoms (6,8). Neurologic examination is usually nonlateralizing; impaired higher cortical functions, hyperreflexia, and asterixis may be present.
Asterixis, a sudden loss of muscle tone that results in a “flapping” tremor, is best assessed by having the patient hyperextend at the elbow and wrist with the fingers spread apart; the asterixis is then best seen at the metacarpophalangeal joints and the wrist. This phenomenon is also seen in hepatic and other metabolic encephalopathies, and, although a nonspecific finding, it may be useful in assessment of a patient with declining renal function (2).
Patients with uremic encephalopathy characteristically may also manifest multifocal myoclonus. Myoclonus is a sudden rhythmic movement of muscle groups; it is asymmetric and usually not related to seizures. In addition, focal neurologic findings such as extensor plantar response and grasp or snout reflexes have been described. Myoclonus may also occur in kidney disease patients associated with medications such as but not limited to serotonin reuptake inhibitors and narcotic analgesics.
The presence of focal neurologic findings should be fully investigated to look for other causes of altered mentation. Neuroimaging studies such as computed tomography (CT) or magnetic resonance imaging (MRI) typically show cortical atrophy (9,10). Rarely, changes suggestive of edema are seen involving the basal ganglia, cerebral cortex, and centrum semiovale (10). Interestingly, these changes are similar to those seen in hypertensive encephalopathy. The cause and significance of these changes are unknown in the absence of marked hypertension, but they are thought to be related to transient ischemia or cerebral edema. The possibility that these changes are related to uremic toxins or other metabolic derangement cannot be excluded because these changes usually resolve after dialysis (10).
Posterior reversible leukoencephalopathy syndrome (PRLS) is a well-defined syndrome seen in associated with severe hypertension and acute renal insufficiency (11). Clinical features include headaches, altered mentation, visual changes, and even cortical blindness related to the predilection for involvement of the occipital lobes (12). These findings have also been described in patients with organ transplantation and with use of certain immunosuppressant medications such as cyclosporine and tacrolimus (13,14). Typically, this condition is reversible with complete resolution of symptoms, clinical and radiologic changes with control of blood pressure and/or discontinuation of offending agents (FIGURE 30.1).
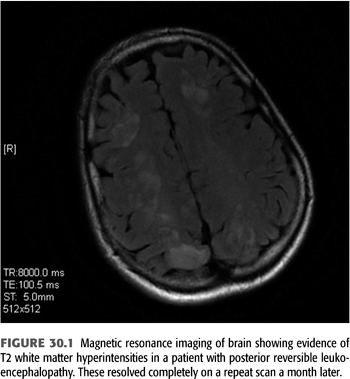
Cerebrospinal fluid (CSF) examination usually is normal, although mildly elevated protein is seen in more than half of the patients with uremia and lymphocytic pleocytosis may be seen in up to 10% of patients (2,15). It is of critical importance that encephalitis, meningitis, and other causes of CSF abnormalities be excluded before attributing the CSF changes to uremia alone.
One of the most interesting questions in uremia relates to the pathogenesis of uremic encephalopathy. The substance responsible for the encephalopathy is not known. There is no direct relationship between urea or creatinine levels and the level of encephalopathy (5–7). Most likely, uremic encephalopathy is a result of a combination of factors, including accumulation of various organic acids and substances such as urea guanidine (16–17), myoinositol, purines, organic phosphates, oxalate, ascorbic acid, amino acid peptides, parathyroid hormone (PTH), β2-microglobulin, methylguanidine, guanidinosuccinic acid, hippuric acid, polyamines, phenols, and indoles, in addition to urea and creatinine (6,7,18–20). In animal models, a number of these compounds of different molecular weights produce toxicity similar to that seen in uremic syndrome.
Urea and creatinine are considered low molecular weight compounds (less than 300 Da), whereas myoglobulin is considered high molecular weight (greater than 12,000 Da). Similarly, compounds with molecular weight between 300 and 12,000 Da such as β2-microglobulins are considered “middle molecules.” Because the middle molecules are removed less efficiently than urea and creatinine during hemodialysis, these are considered likely candidates that contribute to uremic encephalopathy. These compounds include PTH, peptides, glucuronated conjugates, and β2-microglobulin. Compounds such as methylguanidine, hippuric acid, polyamines, phenols, and indoles have lower molecular weight, but their kinetics of removal during dialysis are similar to the “classic” middle molecules (19).
As stated previously, PTH has been implicated in the pathogenesis of uremic encephalopathy (20–22) because of secondary hyperparathyroidism and effect of PTH on neuronal function (21). Even in the absence of renal insufficiency, elevated levels of PTH can result in confusion and altered mental status. In animal models of uremia, there is a marked increase in intracellular calcium (7,20), suggesting a possible link between PTH and neuronal dysfunction. In these animals, blockade of PTH function reverses the symptoms associated with uremic encephalopathy. Increased intracellular calcium results in impairment of energy metabolism with reduced adenosine triphosphate (ATP) levels because of abnormal mitochondrial function. There is also evidence to suggest abnormal phospholipid metabolism related to the previously mentioned events causes membrane instability and further neuronal injury (3,15–20).
Accumulation of various organic acids and the middle molecules described previously (6,7,18,19), either from increased permeability of the blood–brain barrier or from impaired cellular transport mechanisms, may further compromise neuronal function. Biasioli et al. (8) describe an alteration in amino acid profile in serum and CSF of uremic patients, which indicates an increase in glycine, dopamine, and serotonin and a decrease in γ aminobutyric acid (GABA). Increased dopamine, serotonin, and glycine accumulation may result in increased irritability, sensorial clouding, tremors, and unsteadiness. Low GABA levels may lead to seizures and myoclonus and may exacerbate other uremic symptoms (8,20). However, no single abnormality fulfills all the criteria for being the putative neurotoxin, and uremic encephalopathy is most likely the result of derangements of neuronal function at multiple levels. Positron emission tomography (PET) scans in patients with CKD show reduced brain metabolism that correlates well with cognitive dysfunction and may be explained by the molecular events discussed previously (23). Additional metabolic derangements such as hyponatremia and hypernatremia have been associated with CKD and dialysis, these may result in altered mental status, encephalopathy, and seizures and addressed elsewhere in this text.
Seizures
Seizures may occur during the course of uremic encephalopathy in as many as 10% to 20% of patients and are usually generalized tonic clonic, although simple or complex partial seizures may also be seen (2,6). It is important to exclude drug toxicity as a reversible cause of seizures, because a number of commonly used drugs are associated with seizures in kidney disease. Drugs such as quinolones, penicillins, cephalosporins, acyclovir, and erythropoietin lower the seizure threshold and can lead to clinical seizure activity (24,25). The diagnosis is one of exclusion, and withdrawal of the presumed offending agent is enough to control the seizure activity.
Electroencephalography (EEG) in patients with seizures usually shows marked slowing of the background with increased θ (4 to 7 Hz) and δ (1 to 3 Hz) activity, as well as bilateral frontal paroxysmal slowing and occasional frontal epileptiform activity in the form of spikes and sharp waves (FIGURE 30.2) (6–8,26). It is important to note that these epileptiform abnormalities may be seen in patients with uremia and without a history of seizures as well. This finding in patients without seizures most likely represents evidence of cortical irritability and by themselves do not require any treatment. A characteristic EEG finding in patients with uremic encephalopathy is triphasic waves, which are seen with advanced stages (FIGURE 30.3). However, this pattern may also be seen in other metabolic disorders such as hepatic encephalopathy, severe hyponatremia, and certain drug intoxications (e.g., lithium) (26).
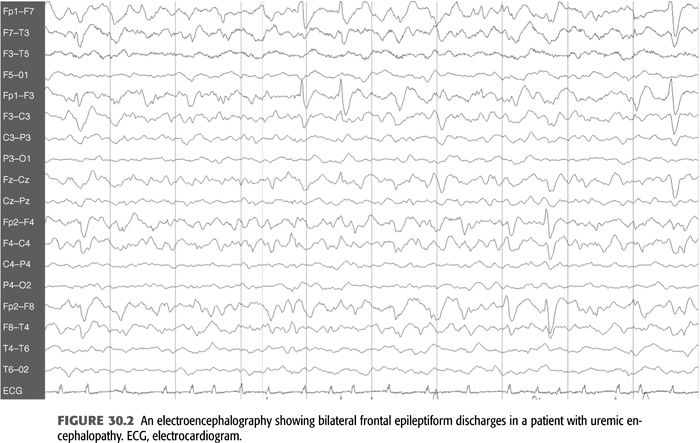
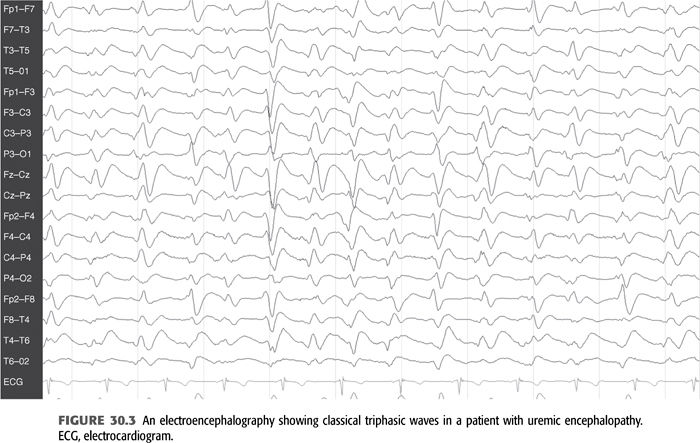
The seizures should be treated the same as in other patients with metabolic encephalopathies. Long-term antiepileptic drug (AED) therapy is only required in patients with a high risk of recurrence, such as those with multiple seizures, history of recent status epilepticus, focal neurologic deficits, focal abnormality involving the cerebral cortex on imaging, and seizures in the absence of any specific metabolic derangement. If long-term antiepileptic medication is indicated, the impact of renal insufficiency and dialysis on drug metabolism should be taken into account (TABLE 30.3) (27–30). Phenytoin, carbamazepine, and valproic acid are all reasonable first-line agents for the treatment of seizures, although the newer agents have less systemic toxicity associated with them and are preferred by most neurologists. Phenytoin, levetiracetam, lacosamide, and valproate have the advantage of being available in intravenous (IV) form and can be given rapidly in an emergency. Because phenytoin is one of the most commonly used antiepileptic medications and its metabolism is affected by uremia (2,31,32) the pharmacokinetics in kidney disease must be well understood before using them in these medically complex patients. Because of expanded volume of distribution there is a reduction in total level, although this is offset by a marked increase of the free fraction of the drug from 10% to 25% (31,32). This may result in clinical toxicity, even though the total level may be low; therefore, free phenytoin levels, which are easily available in most centers, should be used as a guide to dosing. The half-life of phenytoin is also reduced from 13 hours to approximately 8 hours; therefore, three times a day dosing is recommended.
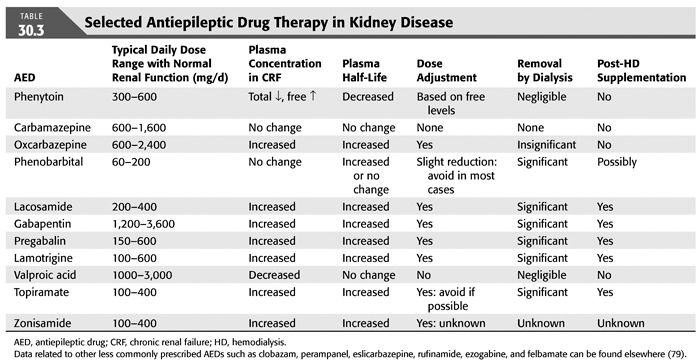
Valproic acid is now also available in IV formulation as is levetiracetam and lacosamide, although there is very little confirmatory data regarding its use in management of status epilepticus. We prefer to use these agents for seizures initially, and it requires careful monitoring for toxicity due to the impact of renal insufficiency on their respective serum levels (as noted in TABLE 30.3). Drugs that are metabolized by the cytochrome P-450 such as phenytoin, carbamazepine, oxcarbazepine, and phenobarbital are enzyme inducers and may interfere with metabolism of a number of other drugs including tacrolimus, a commonly used antirejection drug; therefore, caution is advised when using these antiepileptic agents in medically complex patients. We typically tend to avoid use of phenobarbital in most patients due to numerous other options available and its significant toxicity. A number of new antiepileptic agents have been approved by the U.S. Food and Drug Administration (FDA) over the last few years and may be a reasonable alternative for these patients (TABLE 30.3). The choice of AED is based on the need for rapid dosing (phenytoin, valproic acid, levetiracetam, lacosamide), side effect profile (potential renal toxicity with topiramate and zonisamide), and significant dosing adjustment of the drug (gabapentin, levetiracetam, lacosamide, oxcarbazepine). Phenytoin is still preferentially used for rapid IV dosing in a patient with acute repetitive seizures or status epilepticus. However, in a patient with focal seizures who does not have status epilepticus one may choose a drug like carbamazepine as dosing adjustments are not necessary in patients with renal insufficiency. AEDs such as lamotrigine and levetiracetam are additional exceedingly effective and safe options for add-on therapy if a single drug is ineffective at its maximally tolerable dose. However, these drugs are renally excreted and substantial dose adjustments are required especially with hemodialysis. A neurologic consultation may also be helpful in choosing the most appropriate AED.
Cerebrovascular Disease in Chronic Kidney Disease
Patients on chronic hemodialysis have a fivefold higher risk of cerebrovascular disease compared with the normal population (33–36). This may be related to the high prevalence of hypertension (33), underlying diabetes, hyperhomocysteinemia, dyslipidemia, and atherosclerosis in hemodialysis patients (34). There are considerable data that suggest hyperhomocysteinemia is an important risk factor in patients with ischemic cerebrovascular disease and chronic renal insufficiency (34). It is important to identify the cause of hyperhomocysteinemia, which may be related to folate, cobalamin, or pyridoxine deficiency or because of a mutation of methylene tetrahydrofolate reductase (MTHFR) enzyme. Folic acid at 1 to 5 mg is recommended for most patients with MTHFR mutation, for folate deficiency, or when no other cause of hyperhomocysteinemia is identified.
The stroke risk is increased in patients with CKD and those undergoing dialysis. In a paper published recently (35), stroke occurred at a rate of 49.2/1,000 patient-years with a greater risk of ischemic stroke in patients undergoing hemodialysis compared to peritoneal dialysis. However, the risk of hemorrhagic stroke did not appear to show such an association. The management of ischemic cerebrovascular disease is the same as in patients without kidney disease. Addressing associated risk factors such as hypertension, hypercholesterolemia, and other modifiable risk factors is critically important. Antiplatelet agents such as aspirin and clopidogrel are recommended for atherothrombotic disease, whereas patients with embolic infarction may need to be anticoagulated with warfarin. The decision to anticoagulate is based on the size of the infarct, the risk of recurrence, and the risk of systemic or intracranial bleeding in a particular patient.
In another older study intracerebral hemorrhage was seen more commonly than cerebral infarct as the cause of stroke and most likely is related to hypertension (36). The actual incidence of intracerebral hemorrhage was noted to be 12.3 per 1,000 patient-years, and that of cerebral infarct is 3.9 per 1,000 patient-years (32). This was also associated with an overall 30-day stroke mortality that is 74.4%, compared with 12.3% in the general population. This high mortality could be partly related to the disproportionately high incidence of intracerebral hemorrhage and associated systemic disease that was noted in this particular study. However, a more recent study has shown a higher incidence of ischemic infarction compared to intracerebral hemorrhage in patients with CKD who are receiving hemodialysis (35).
Patients with autosomal dominant polycystic kidney disease (ADPKD) have a higher prevalence of intracranial aneurysms (35). These aneurysms are usually small, involve the anterior circulation, and are frequently multiple. The prevalence of these aneurysms is approximately 5% to 12%, depending on the screening method used. Cerebral angiography is the gold standard diagnostic method for locating these aneurysms (FIGURE 30.4) (11); however, magnetic resonance angiography (MRA) is sometimes a useful screening tool. It is safe in patients with kidney disease when no contrast is needed.
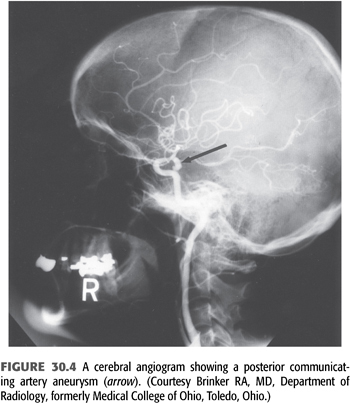
Screening is recommended for patients above the age of 20 as aneurysms are typically not noted in individuals younger than that. Risk factors for aneurysms include a family history of aneurysms, a previous history of aneurysm or subarachnoid hemorrhage, polycystic kidney disease, as well as certain connective tissue disorders such as Marfan or Ehler-Danlos syndrome type IV (35).
Clinical features may be related to mass effect in the case of a large aneurysm or because of a sudden rupture. Patients with subarachnoid hemorrhage usually present with sudden explosive headache that is frequently described as the “worst headache of their life.” Nuchal rigidity and third cranial nerve (CN) palsy may also be present but are not seen in all patients. The initial evaluation must be rapid in patients with suspected subarachnoid hemorrhage, and urgent admission to an intensive care unit is warranted. The overall mortality of a ruptured aneurysm is approximately 30% to 50% in patients with or without ADPKD. Timely intervention can be lifesaving, and most of interventions are now planned and done before development of vasospasm, which usually occurs 3 to 4 days after rupture. In addition to open craniotomy, endovascular procedures are being more commonly utilized in these medically challenging patients (37).
Management of asymptomatic aneurysms guidelines have previously been published (38). Patients with ADPKD should undergo screening every 5 years due to a high prevalence of intracranial aneurysms (39). There is a strong correlation between size of the aneurysm, location (posterior circulation > anterior circulation), family history, and risk of rupture. Aneurysm location does also affect management because those located in the posterior circulation such as the basilar artery or posterior communicating arteries are more likely to rupture irrespective of size. Other factors that may have an impact on management of unruptured aneurysms include previous subarachnoid hemorrhage; age; family history; coexisting medical conditions; aneurysm characteristics such as size, location, and morphology; and neurosurgical expertise. In the authors’ opinion, surgical decisions in an asymptomatic patient with intracranial aneurysms should be individualized after an informed discussion between the neurosurgeon, endovascular neurologist, nephrologist (in case of associated renal disease), and the patient.
Approach to a Patient with Confusion and Kidney Disease
In a patient who develops confusion associated with renal insufficiency, it is imperative to exclude other causes that may mimic uremic encephalopathy. A complete history and physical including a careful neurologic examination is mandatory. A systematic approach should be adopted to evaluate these patients. In these medically complex patients, systemic infections may result in profound confusion and should be looked for in addition to a complete neurologic evaluation. The differential diagnosis and the diagnostic workup for some of the neurologic diseases are listed in TABLE 30.4. It is important to exclude nonconvulsive status epilepticus as a cause of confusion in a patient with uremia. It is a potentially life-threatening disorder that can be easily diagnosed by EEG and responds to antiepileptic therapy. If unrecognized and untreated, nonconvulsive status epilepticus can lead to irreversible neurologic injury.
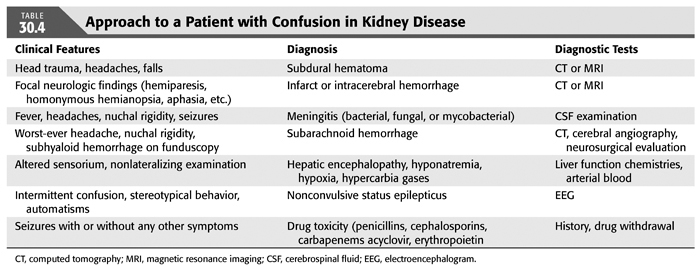
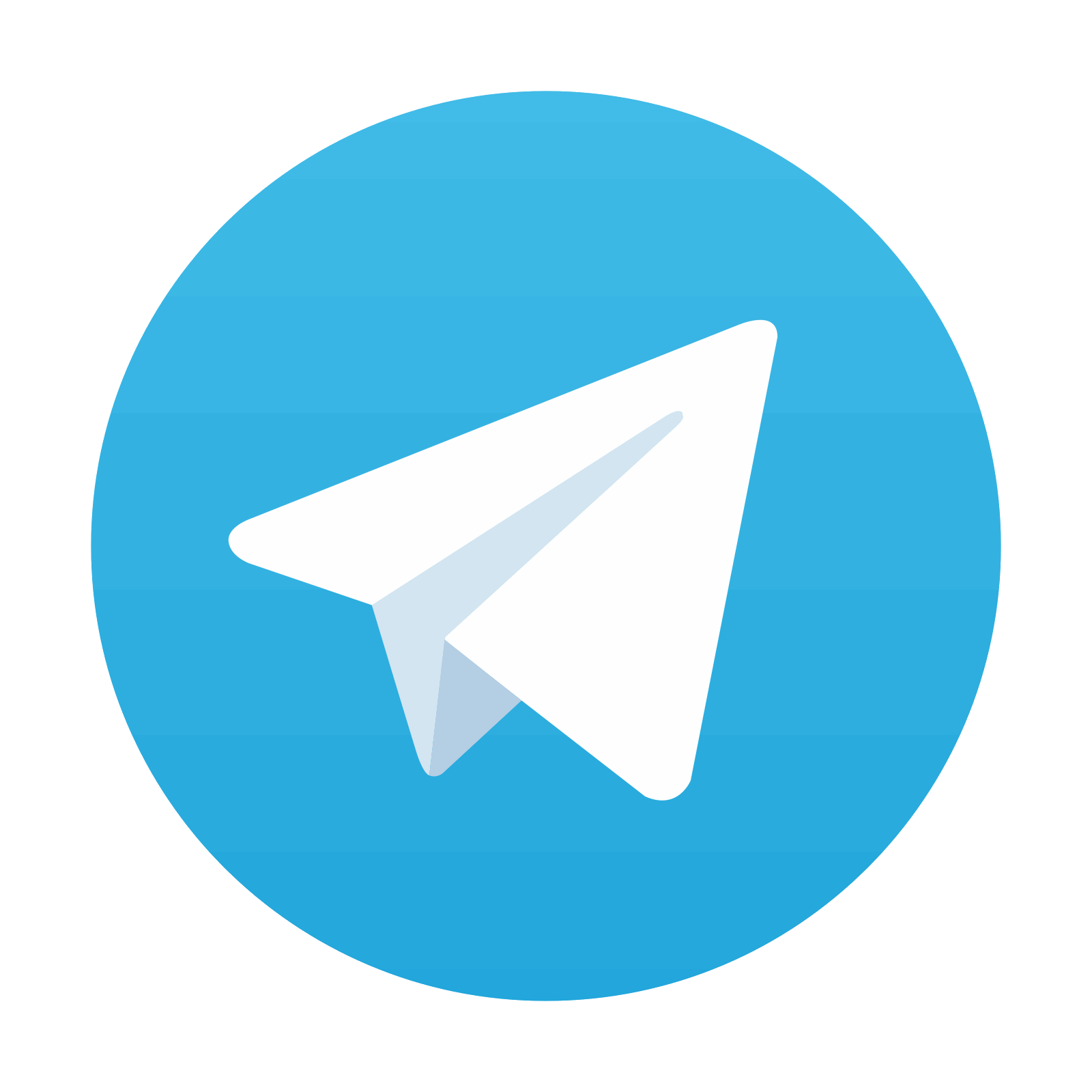
Stay updated, free articles. Join our Telegram channel

Full access? Get Clinical Tree
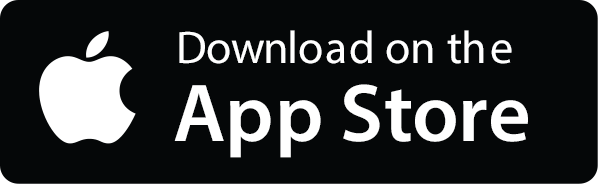
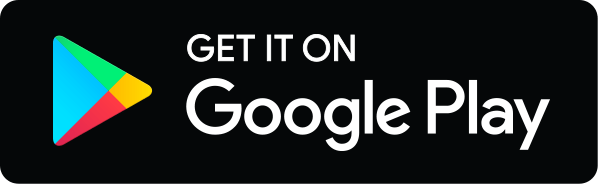