Abstract
The transition from placental gas exchange to air breathing is a complex process that requires adequate removal of fetal lung fluid and a concomitant increase in perfusion of the newly ventilated alveoli. In neonates who are unable to make this transition, varying degrees of respiratory distress and impairment of gas exchange are common. Therapeutic approaches that can facilitate fetal lung fluid clearance are likely to reduce pulmonary morbidity in the neonatal period and help in designing therapies to combat lung edema formation in postnatal life.
Keywords
fetal lung fluid, neonatal transition, transient tachypnea of the newborn, respiratory distress syndrome
- •
Fetal Lung Liquid and Its Physiologic Significance
- •
How Is the Fetal Lung Fluid Cleared?
- •
Sodium Channel Pathology in the Lung
- •
What Causes the Neonatal Lung Epithelium to Switch to an Absorptive Mode?
Often signaled by a loud cry, the birth of a neonate marks a remarkable transition from its dependence for gas exchange on the placenta to an independent state of air breathing and gas exchange in the lungs. Clearing the fluid-filled lungs is a significant component of this transition. Scientists have long known that fetal lungs are full of fluid, initially presumed to be an extension of the amniotic fluid pool. However, studies have confirmed that fetal lungs themselves, rather than the amniotic sac, are the source of the chemically distinct liquid that fills the lungs during development. Through an active process involving chloride secretion by the respiratory epithelium, this liquid forms a slowly expanding structural template that prevents collapse and is essential for growth of fetal lungs.
For effective gas exchange to occur, rapid clearance of liquid from potential alveolar airspaces during and soon after birth is essential for establishing the timely switch from placental to pulmonary gas exchange. It is clear now that traditional explanations that relied on mechanical factors and Starling forces can only account for a small fraction of the fluid absorbed and that the normal transition from liquid to air inflation is considerably more complex than the characteristic “vaginal squeeze” theory suggests. Physiologic events beginning days before spontaneous delivery are accompanied by changes in the hormonal milieu of the fetus that pave the way for a smooth neonatal transition, including clearance of the large body of lung fluid. Respiratory morbidity resulting from failure to clear the lung fluid is common and can be particularly problematic in some infants delivered prematurely or when delivery occurs operatively before the onset of spontaneous labor. The same pathways are involved in the development of pulmonary edema in acute respiratory distress syndrome (ARDS) that develops in response to infections such as respiratory syncytial virus. This chapter considers some of the experimental work that provides the basis for our current understanding of lung liquid dynamics before, during, and after birth, focusing on the various pathways and mechanisms by which this process occurs.
Fetal Lung Liquid and Its Physiologic Significance
As stated, the lung is a secretory organ during development, displaying breathing-like movements, but without any contribution to respiratory gas exchange. The small fraction of the combined ventricular output of blood from the heart that circulates through the pulmonary circulation allows the delivery to the lung epithelium of the substrates needed to make surfactant and secretion of up to 5 mL/kg per hour lung fluid at term gestation. Several studies have shown that the presence of an appropriate volume of secreted liquid within the fetal respiratory tract is essential for normal lung growth and development before birth. Conditions that interfere with normal production of lung liquid, such as pulmonary artery occlusion, diaphragmatic hernia with displacement of abdominal contents into the chest, and uterine compression of the fetal thorax from chronic leak of amniotic fluid, also inhibit lung growth. Conversely, excessive accumulation of lung fluid, such as that after tracheal occlusion, leads to excessive but abnormal lung growth.
Fig. 14.1 is a schematic diagram showing the fluid compartments of the fetal lung. Potential air spaces are filled with liquid that is rich in chloride (≈150 mEq/L) and almost free of protein (<0.03 mg/mL). Whereas the lung epithelium has tight intercellular junctions that provide an effective barrier to macromolecules, including albumin, the vascular endothelium has wider openings that allow passage of large plasma proteins, including globulins and fibrinogen. Consequently, liquid in the interstitial space, which was sampled in fetal sheep by collecting lung lymph, has a protein concentration that is about 100 times greater than the protein concentration of liquid contained in the lung lumen. Despite the large transepithelial difference in protein osmotic pressure, which tends to inhibit fluid flow out of the interstitium, active transport of chloride (Cl − ) ions across the fetal lung epithelium generates an electrical potential difference that averages about −5 mV, luminal side negative.

The osmotic force created by this secretory process overcomes that of the protein gradient, pulling liquid from the pulmonary microcirculation through the interstitium into potential air spaces.
In vitro experiments using cultured explants of lung tissue and monolayers of epithelial cells harvested from human fetal lung have indicated that cation-dependent chloride transport, driven by epithelial cell Na + , K + -ATPase, is the mechanism responsible for liquid secretion into the lumen of the mammalian lung during fetal life. In fetal sheep and lambs, lung epithelial Cl − transport is inhibited by diuretics that block Na + , K + , 2Cl − cotransport. This finding supports the concept that the driving force for transepithelial Cl − movement in the fetal lung is similar to the mechanism described for Cl − transport across other epithelia, although the specific anion channels responsible have not yet been definitively identified. Accordingly, Cl − enters the epithelial cell across its basal membrane linked to sodium (Na + ) and potassium (K + ) ( Fig. 14.2 ). Na + enters the cell down its electrochemical gradient and is subsequently extruded in exchange for K + (three Na + ions exchanged for two K + ions) by the action of Na + , K + -ATPase located on the basolateral surface of the cell. This energy-dependent process increases the concentration of Cl − within the cell so that it exceeds its electrochemical equilibrium. Cl − then passively exits the epithelial cell through anion-selective channels that are located on the apical membrane surface. Na + traverses the epithelium via paracellular pathways or via nonselective cation channels that have been identified in fetal distal airway epithelium; water can flow either between epithelial cells or through water channels, one of which (aquaporin 5) is abundantly expressed in alveolar type I (AT-I) lung epithelial cells.

Although the Cl − concentration of liquid withdrawn from the lung lumen of fetal sheep is about 50% greater than that of plasma, the Na + concentration is virtually identical to that of plasma. The concentration of bicarbonate in lung liquid of fetal sheep is less than 3 mEq/L, yielding a pH of approximately 6.3 and indicating that the lung epithelium may actively transport bicarbonate out of the lung lumen. The demonstration that acetazolamide, a carbonic anhydrase inhibitor, blocks secretion of lung liquid in fetal sheep supports this view. Both physiologic and immunohistochemical studies have shown that H + -ATPases are present on the respiratory epithelium of fetal sheep, where they likely provide an important mechanism for acidification of liquid within the lung lumen during development. In vitro electrophysiologic studies using fetal rat lung epithelial cells provided evidence that exposure to an acid pH might activate Cl − channels and thereby contribute to the production of fetal lung liquid. In fetal dogs and monkeys, however, the bicarbonate concentration of lung luminal liquid is not significantly different from that of fetal plasma. Thus, the importance of lung liquid pH and acidification mechanisms during human lung development in utero remains unclear.
The volume of liquid within the lung lumen of fetal sheep increases from 4 to 6 mL/kg at midgestation to more than 20 mL/kg near term. The hourly flow rate of lung liquid increases from approximately 2 mL/kg body weight at midgestation to approximately 5 mL/kg body weight at term. Increased production of luminal liquid during development reflects a rapidly expanding pulmonary microvascular and epithelial surface area that occurs with proliferation and growth of lung capillaries and respiratory units. The observation that unilateral pulmonary artery occlusion decreases lung liquid production in fetal sheep by at least 50% shows that the pulmonary circulation, rather than the bronchial circulation, is the major source of fetal lung liquid. Intravenous infusion of isotonic saline at a rate sufficient to increase lung microvascular pressure and lung lymph flow in fetal lambs had no effect on liquid flow across the pulmonary epithelium. Thus, transepithelial Cl − secretion appears to be the major driving force responsible for the production of liquid in the fetal lung lumen. In vitro studies of epithelial ion transport across the fetal airways indicate that the epithelium of the upper respiratory tract also secretes Cl − , thereby contributing to lung liquid production. However, most of this liquid forms in the distal portions of the fetal lung, where total surface area is many times greater than it is in the conducting airways.
How Is the Fetal Lung Fluid Cleared?
Several studies have demonstrated that both the rate of lung liquid production and the volume of liquid within the lumen of the fetal lung normally decrease before birth, most notably during labor. Thus, lung water content is about 25% greater after premature delivery than it is at term, and newborn animals that are delivered by cesarean section without prior labor have considerably more liquid in their lungs than do animals that are delivered either vaginally or operatively after the onset of labor ( Table 14.1 ). In studies with fetal sheep, extravascular lung water was 45% less in mature fetuses that were in the midst of labor than in fetuses that did not experience labor, and there was a further 38% decrease in extravascular lung water measured in term lambs that were studied 6 hours after a normal vaginal birth.
Failure of antenatal decrease in fetal lung fluid |
|
Excessive production of fluid |
|
Decreased epithelial transport of sodium and water |
|
To achieve this, the lung epithelium is believed to switch from a predominantly Cl − -secreting membrane prior to birth to a predominantly Na + -absorbing membrane after. Work performed over the past two decades to understand the mechanism(s) responsible for fetal lung fluid clearance have shown that active Na + transport across the pulmonary epithelium drives liquid from lung lumen to the interstitium, with subsequent absorption into the vasculature. In the lung, Na + reabsorption is a two-step process ( Fig. 14.3 ). The first step is passive movement of Na + from the lumen across the apical membrane into the cell through Na + permeable ion channels. The second step is active extrusion of Na + from the cell across the basolateral membrane into the serosal space. Several investigators have demonstrated that the initial entry step primarily involves sodium-specific apical channels (epithelial sodium channels [ENaC]) that are particularly sensitive to amiloride, a diuretic. Indeed, cDNAs that encode amiloride-sensitive Na + channels in other Na + transporting epithelia have also been cloned from airway epithelial cells. This is consistent with studies by O’Brodovich et al., who have shown that intraluminal instillation of amiloride in fetal guinea pigs delays lung fluid clearance.

More recent studies using the patch-clamp technique have confirmed the role of ENaC channels in AT-I and AT-II cells in the vectorial transport of Na + from the apical surface. Increased production of the mRNA for amiloride-sensitive ENaC in the developing lung has been correlated with the transition from a secretory to absorptive state. Much of this information has come from studies using AT-II cells. Recent studies have shown that AT-I cells also express functional Na + channels and other transporters capable of salt and fluid transport. Based on work in animals and humans, ENaC channels are thought to be responsible for 40% to 70% of sodium transport. Nonspecific cation channels and cyclic nucleotide gated channels are also present in alveolar cells and contribute to the amiloride-insensitive sodium and fluid uptake.
Sodium Channel Pathology in the Lung
ENaC channels are primarily composed of three subunit types, α, β, and γ, each with a role in sodium transport but with varied relative importance in mammalian species. A fourth subunit, δ, first identified in the brain and since shown to be coexpressed with the other subunits in lung tissue, is of uncertain significance. Hummler et al. have shown that inactivating the α-ENaC (α-subunit of the ENaC) leads to defective lung liquid clearance and premature death in mice. Inactivating β- and γ-ENaC subunits also leads to early death in newborn mice, though this is due to fluid and electrolyte imbalances, suggesting that α-ENaC expression is critical for fetal lung fluid absorption. In later work, Hummler et al. showed that a mouse model of increased α-ENaC activity demonstrated increased alveolar fluid clearance after induction of pulmonary edema. This is direct evidence that in vivo ENaC constitutes the rate-limiting step for Na + absorption in epithelial cells of the lung, and thus in the adaptation of newborn lungs to air breathing. It also supports the hypothesis that in many newborns who have difficulty in the transition to air breathing, Na + channel activity may be diminished, albeit transiently.
Studies in human neonates have shown that immaturity of Na + transport mechanisms contributes to the development of transient tachypnea of the newborn (TTN) and respiratory distress syndrome (RDS; Table 14.2 ). Gowen et al. were the first to show that human neonates with TTN had an immaturity of the lung epithelial transport, measured as an amiloride-induced drop in the potential difference between the nasal epithelium and subcutaneous space. Nasal potential difference is a good measure of the net electrogenic transport of Na + and Cl − across the epithelial layer and has been shown to mirror image ion transport occurring in the lower respiratory tract. The potential difference was reduced in infants with TTN, suggesting deficient Na + transport, and recovery from TTN in 1 to 3 days was associated with an increase in potential difference to normal level.
Decreased sodium and water transport |
|
Excessive sodium and water transport |
|
Similar studies have now been conducted in premature newborns with RDS, and the results are consistent with impaired Na + transport in these infants. Barker et al. measured nasal transepithelial potential difference in premature infants less than 30 weeks’ gestation. Authors found that maximal nasal epithelial potential difference increased with birth weight and was lower in infants with RDS. Premature infants without RDS had a nasal potential difference similar to normal full-term infants. Furthermore, the ability of amiloride to affect the potential difference was lower in preterm infants with RDS on day 1 of life, reflecting lower amiloride-sensitive Na transport. A clear gender difference is seen in RDS in humans, with male infants at higher risk, though the mechanisms are not clearly identified. Fetal lung cells from male rats have shown impaired sodium transport compared to those from female pups, potentially due to differences in responsiveness to sex hormones.
Additional evidence indicates that the ability of various agents to increase lung fluid absorption in fetal lambs is gestational-age dependent. The mechanism for poor response of immature lungs to agents that stimulate Na + transport is not known. Deficiencies could exist in one or more of several steps, including β-receptor, GTP-binding proteins, adenyl cyclase, protein kinase A, or the Na + channel and its regulatory proteins. Studies have shown that the expression of α-subunit of ENaC is developmentally regulated in rats and in humans. Helve et al. studied ENaC RNA levels in full-term and preterm infants with and without RDS. Lower α- and β-ENaC levels were noted in preterm infants, correlated with decreasing gestational age. This continues in late preterm (LPT) infants and up to early term, with infants less than 39 and 0/7 weeks having lower α-ENaC mRNA levels just after birth. Together, these studies provide important evidence for the role of Na channel activity in the pathogenesis of RDS and TTN.
Pseudohypoaldosteronism type I (a renal salt-wasting disease) has been reported to be associated with mutations involving the α-subunit of ENaC. Indeed, patients with the autosomal recessive form of the disease have been reported with severe RDS at birth and childhood pulmonary disease of varying degrees, with documented gene abnormalities in alpha ENaC. However not all patients have neonatal lung disease, which is unexpected given that the α-subunit is so critical for ENaC function.
A complex and yet incompletely defined relationship exists between Na + and Cl − channels. Cystic fibrosis (CF) is a genetic disease caused by mutations of the CF transmembrane conductance regulator (CFTR), which has been identified as a cyclic AMP (cAMP)-dependent Cl channel. In the lungs of CF patients, amiloride-sensitive Na + absorption is increased, and aerosolized amiloride has been used to reverse this imbalance. However, despite the absence of functional CFTR activity in fetuses who will go on to develop CF, fetal lung fluid production during gestation is unaffected, and the lungs are normally developed at birth. These findings contrast with recent studies on infants with congenital diaphragmatic hernia (CDH), who do not have a specific deficiency in ENaC or other channels. However, these infants do have respiratory distress at birth and may have this compounded by decreased alveolar fluid clearance. Animal models of term equivalent CDH rats show net lung fluid secretion rather than the absorption seen in control subjects. Animal and human studies have also shown decreased levels of various ENaC subunits (α- and β-subunits in rats; β- and γ-subunits in humans) in term infants with CDH, the cause of which is unknown. Therefore, examining the molecular mechanisms and the cellular regulation of Na + reabsorption is important in understanding both normal lung development and physiology, but also abnormalities in lung Na and water balance in both fetal and adult lungs.
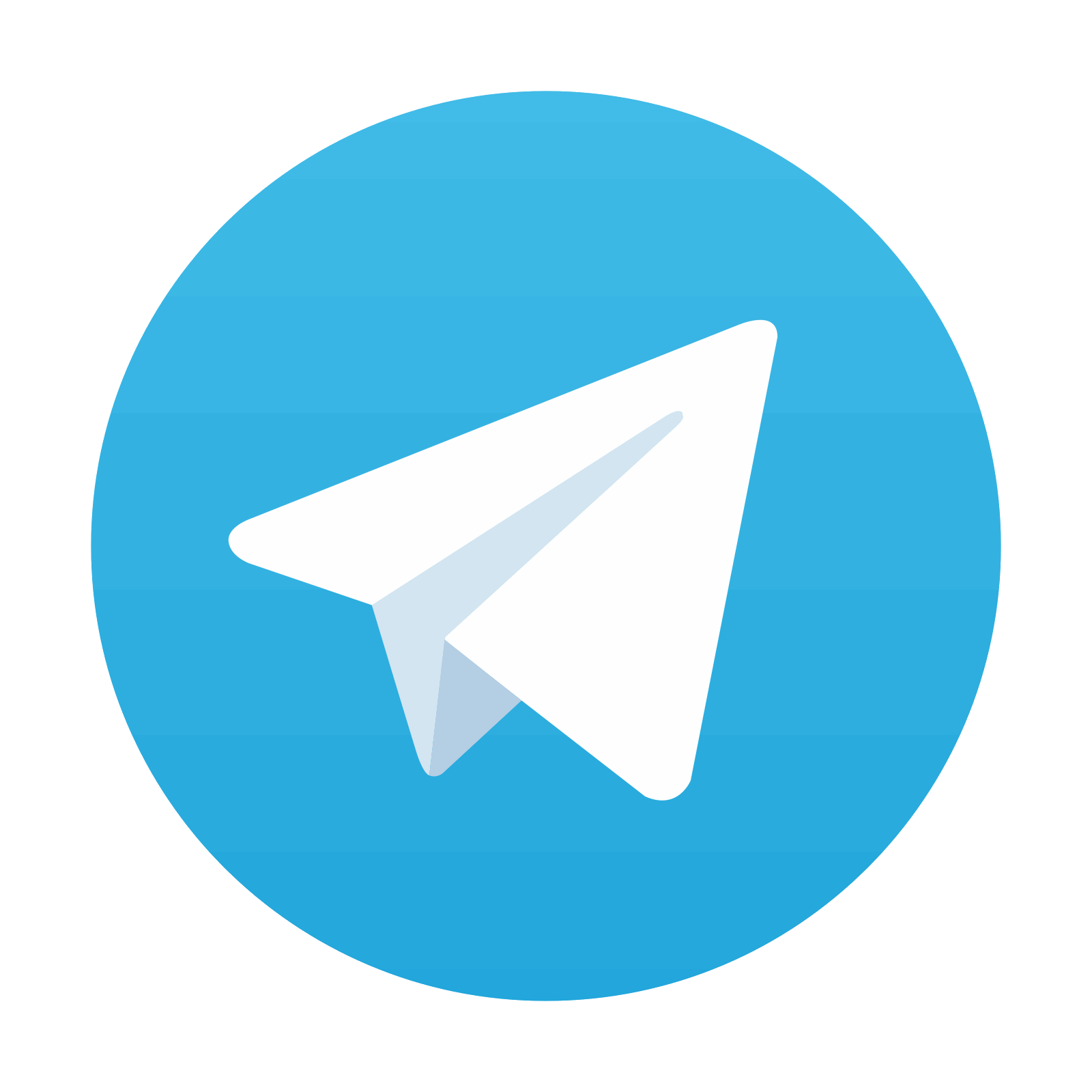
Stay updated, free articles. Join our Telegram channel

Full access? Get Clinical Tree
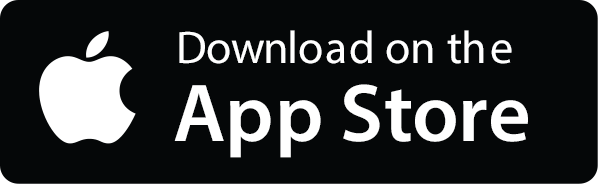
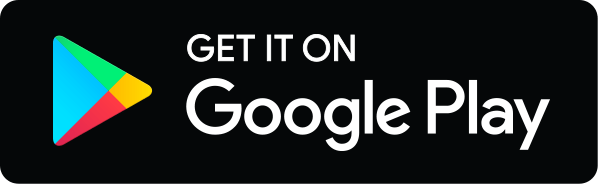