Lasers and Electrosurgery in Hysteroscopy
Robert D. Tucker
Michael S. Baggish
The use of energy devices, such as laser and electrosurgery, in hysteroscopy for the treatment of intrauterine disease allows physicians to control tissue destruction, decrease bleeding, and minimize patient discomfort. Regardless of whether laser light or electricity is used, the underlying mechanism is the heating of tissue to cut, coagulate, or ablate. Power supplies have become commonplace in open, hysteroscopic, and laparoscopic procedures principally because they provide a dry surgical field and reduce surgery time. Since energy sources have advantages and disadvantages, physicians need to become intimately familiar with the technology that they use to achieve the desired results without complications. Although the following sections discuss the basic uses of each technology, the reader is urged to read the manufacturer’s operator’s manual for specific details.
Lasers
History of the Laser
In 1900, the German physicist, Max Planck, developed the quantum theory, which described energy traveling in packets rather than by waves. In 1913, Niels Bohr postulated that the hydrogen atom had a number of orbits in which electrons rotate around the nucleus. The far orbits correspond to high energy states (unstable), and when electrons dropped from an outer orbit to one closer to the nucleus, a packet (quantum) of light was emitted called a photon. The process of stimulated emission of radiation was first described by Albert Einstein in 1917. Charles Townes became interested in microwave spectroscopy while working on radar technology during World War II. In 1957, Townes and Schawlow investigated the possibilities of making an optical maser and in 1958 published their data. Fox and Li and Boyd and Gordon further supported the work of Townes and Schawlow. In 1960, Maiman at Hughes Research constructed a ruby laser, and in 1961, Javan, Bennett, and Herriott invented the helium-neon laser. The carbon dioxide laser was invented by Patel in 1964. Since that time, many varied types of industrial and surgical lasers have appeared on the market. Virtually every specialty in medicine ranging from dermatology to cardiovascular surgery uses lasers.
Lasers provide an alternative energy source to electrosurgery. Although several wavelengths exist, the neodymium–yttrium-aluminum-garnet (Nd-YAG) laser has been used most often for operative hysteroscopy (Fig. 10.1). The reasons for this are as follows:
The Nd-YAG laser offers a high power range.
The laser energy is delivered to the operative site by quartz or silicone fibers ranging in diameter from 600 to 1,200 μm.
The wavelength penetrates all liquid media.
The laser provides excellent hemostasis; it is predominantly a coagulator.
The laser does not require conduction through tissue as do electrosurgical devices. Therefore, lasers can be used with any liquid or gaseous medium. Typically, when the laser is selected as an energy-emitting accessory for hysteroscopic surgery, saline is chosen as the distending medium.
What Is a Laser?
When external energy such as electrical current, light, or heat is applied to an atom, i.e., pumping, part of the energy increases the random motion of the atoms and part is absorbed, causing the electrons orbiting that atom to jump to a higher energy level. This unstable condition lasts for a very short time, and when the electron returns to the ground state, a packet of energy is emitted in the form of a photon (Fig. 10.2A). If the atoms are stimulated in a medium with parallel mirrors at either end, the photon will reflect back and forth between the two mirrors. As these generated photons hit other atoms in the unstable state, additional photons will be emitted from the atom as electrons drop back to their lower energy level. The additional photons will be in phase (i.e., flow in the same direction and frequency as the photon that initially struck the atom) (Fig. 10.2B). Continuing to put energy into these atoms will cause more and more photons to be discharged, thus creating a beam of light that has three characteristics distinguishing it from ordinary light:
Laser beams are collimated, or parallel, which creates a minimal amount of divergence as the light is transmitted from its source; the laser energy is coherent (i.e., all of the waves are in phase); and the laser light is monochromatic (i.e., a single color).
Laser beams are collimated, or parallel, which creates a minimal amount of divergence as the light is transmitted from its source; the laser energy is coherent (i.e., all of the waves are in phase); and the laser light is monochromatic (i.e., a single color).
When a partially transmitting mirror is placed at one end of the optical resonator, a portion of the laser light can be released from the chamber in a controlled fashion (Fig. 10.3). Thus, we have light amplification by the stimulated emission of radiation, from which comes the acronym laser (Tables 10.1 and 10.2).
Although the carbon dioxide laser has been used for many applications in gynecology because of its excellent tissue absorption, this laser has no applications for operative hysteroscopy.
The argon laser was first developed for the treatment of diabetic retinopathy in 1965. This laser produces a visible blue-green light (488 and 515 nm), which is easily transmitted through clear aqueous tissues. Certain tissue pigments such as melanin and hemoglobin will selectively absorb argon laser light. The interaction of low levels of blue-green light with highly pigmented tissue results in coagulation of these pigmented tissues. The argon laser can be transmitted to tissue sites by way of fine quartz fibers and can efficiently be conducted through liquid media (Fig. 10.4). It is a highly effective system for endoscopic delivery. The KTP-532 (potassium titanyl phosphate) laser has similar characteristics to those of the argon laser but emits pure green light and has superior cutting features compared with the argon mixed wavelengths (Fig. 10.5).
TABLE 10.1 Lasers for Hysteroscopic Surgery: Physical Properties | ||||||||||||||||||||||||||||||
---|---|---|---|---|---|---|---|---|---|---|---|---|---|---|---|---|---|---|---|---|---|---|---|---|---|---|---|---|---|---|
|
The Nd-YAG laser is a solid crystal made up of yttrium, aluminum, and garnet (YAG) with surrounding neodymium (Nd). The Nd-YAG light emits in the near infrared region with a wavelength of 1,064 nm. The beam is transmitted through clear liquids, which allows its optimal use in water-filled cavities such as the eye, the bladder, and the uterus. Its absorption is not highly color specific as is the argon laser; however, the beam is efficiently absorbed by dark pigment. The Nd-YAG laser has a characteristic physical property of front scatter and penetrates deeply, resulting in a homogenous zone of thermal coagulation that may extend from 1 to 4 mm beyond the site of impact (Fig. 10.6). The Nd-YAG laser is an excellent tool for tissue coagulation and, like the argon laser, can be delivered through fiberoptic systems.
Sculpted fibers have made it possible for an otherwise singularly coagulating device to become a focused cutting laser (Fig. 10.7).
Sculpted fibers have made it possible for an otherwise singularly coagulating device to become a focused cutting laser (Fig. 10.7).
TABLE 10.2 Lasers for Hysteroscopic Surgery: Clinical Features | |||||||||||||||||||||||||||
---|---|---|---|---|---|---|---|---|---|---|---|---|---|---|---|---|---|---|---|---|---|---|---|---|---|---|---|
|
The holmium-YAG laser is a highly pulsed instrument that can cut tissues with minimal coagulation artifact when the fiber is maximally focused. When the fiber is defocused, the same laser is a superior coagulator. Holmium-YAG lasers have been used extensively in orthopedics but relatively uncommonly in gynecology (Fig. 10.8).
Tissue Effects
When laser light strikes an object including cellular tissue, it may be reflected, transmitted, scattered, absorbed, or a combination of these (Fig. 10.9). Because lasers are a special form of light, they obey all of the physical principles of light energy. The result when a laser beam strikes tissue depends on the wavelength, intensity, duration of the irradiation, and the type of tissue impacted (Fig. 10.10). To exert a biologic action, the beam must be absorbed.
Lasers can be used in a continuous mode, that is, allowing an uninterrupted stream of light energy to flow from the laser tube. This energy can then be focused or defocused by a lens system, thus altering the spot size (laser beam diameter). A larger spot size will diffuse the energy over a greater surface of the target tissue, thus allowing for less penetration. A focused laser beam, on the other hand, will concentrate the laser energy onto a very small area of the tissue, permitting deeper penetration. A deeper tissue effect can also be obtained by increasing the amount of laser light passing through the partially transmitting mirror (raising the power). Thus, the term power density best describes the quantity of laser energy absorbed per unit of tissue (Fig. 10.11). One can increase the power density by decreasing the spot size or by increasing the power that is transmitted from the laser. Tissue penetration is also controlled by length of exposure. If the laser is allowed to strike the tissue for a long time, the beam will penetrate deeper.
![]() FIGURE 10.6 Typically, the neodymium-YAG laser produces front scatter with deep penetration into tissue. The longer the laser remains on the tissue, the wider the zone of coagulation. |
The principal effects on tissue of the lasers used in gynecology are thermal (i.e., light energy is converted to heat). In many respects the thermal action of lasers and electrosurgical devices is identical. Cutting of tissue occurs when temperatures of 100°C are reached rapidly (i.e., vaporization or explosive evaporation) (Figs. 10.12 and 10.13).
![]() FIGURE 10.7 A 1-mm ball-tip sculpted fiber. This bare laser fiber of relatively large caliber is ideal for endometrial coagulation and ablation. |
Laser Techniques
Fiberoptically delivered lasers such as the Nd-YAG laser exert varied effects depending on the position of the fiber relative to the target tissue. For cutting purposes, the operator should select higher powers (i.e., 50 to 60 W) and position the tip of the fiber perpendicular or nearly perpendicular to the tissue. The fiber should be in light contact or 1 to 2 mm above the tissue (Fig. 10.14). This permits the beam to create the smallest spot possible, minimizing divergence. If a sculpted fiber is available, a pointed type should be selected for cutting. The key for cutting such structures as septa, submucous myomas, adhesions, and polyps is high-power density on the one hand and controlled depth on the other. The time expended for fiber movement across the surface that is to be cut is as important as the power density in determining the depth of tissue penetration. The slower the motion,
the deeper the penetration. The shutter of the laser is controlled by the foot pedal. When the pedal is depressed, the laser fires and will continue to discharge until the operator lifts his or her foot from the pedal, which stops the laser emission.
the deeper the penetration. The shutter of the laser is controlled by the foot pedal. When the pedal is depressed, the laser fires and will continue to discharge until the operator lifts his or her foot from the pedal, which stops the laser emission.
![]() FIGURE 10.12 The neodymium-YAG fiber is seen cutting through an adhesion. Bubbles of gas are liberated as the ablation of the tissue occurs. |
For more precise control, the operator can pulse the laser, either electronically or manually. Electronic pulsing permits intermittent discharges of laser light lasting for fractions of seconds. The width of each packet of light (expressed as waves) can be set at 10 to 100 milliseconds, and the interval between pulses can be adjusted for a number per second time span (repetition rate) (e.g., 300 pulses per second) (Fig. 10.15). Allowing intervals of tissue cooling between laser pulses results in cleaner cuts, less thermally induced tissue injury, and greater precision. This property represents a strategic difference between lasers and electrosurgical devices.
![]() FIGURE 10.13 The adhesion is completely and bloodlessly cut by the neodymium-YAG laser, revealing an open cavity behind. |
![]() FIGURE 10.15 A: A pulsed laser creates periods in which the laser energy is on the tissue for a short period of time. The peak power of the beam is much greater than that produced by a continuous-wave laser. The duty cycle of most of these lasers is between 10% and 20%. B: Pulsing creates less thermal artifact because the tissue can recover from the thermal action of the laser between pulses. (A: From Baggish MS. Basic and Advanced Laser Surgery in Gynecology. Norwalk, CT: Appleton-Century-Crofts, 1985, with permission.) |
Tissue coagulation can be produced by lowering the power density. This is most effectively done by pulling the fiber back from the target tissue, causing the beam to diverge. As the beam diverges, the laser spot increases in diameter. Additionally, power can be decreased to 50 W.
Deeper coagulation can be obtained by holding the fiber away from tissue and dropping power density, while at the same time allowing the laser to discharge for relatively longer periods of time (seconds) (Fig. 10.16). In this case, as the laser penetrates the tissue, a sphere of heat forms and expands peripherally as the time period lengthens. Initially the higher temperatures are closer to the fiber but extend outwards with time. Temperatures of 50°C to 70°C desiccate the tissue and result in coagulation (slower action compared with cutting). Coagulation results in cell death and rather typical gross and microscopic changes. The gross changes are seen as whitening or blanching of tissue (Fig. 10.17A). Microscopically, the cells and nuclei are distorted (Fig. 10.17B). The stromal tissues take on a deeper eosinophilic stain. Blood vessels are thrombosed. Tissue sloughing occurs subsequently over a variable period of time following the acute event.
Deeper coagulation can be obtained by holding the fiber away from tissue and dropping power density, while at the same time allowing the laser to discharge for relatively longer periods of time (seconds) (Fig. 10.16). In this case, as the laser penetrates the tissue, a sphere of heat forms and expands peripherally as the time period lengthens. Initially the higher temperatures are closer to the fiber but extend outwards with time. Temperatures of 50°C to 70°C desiccate the tissue and result in coagulation (slower action compared with cutting). Coagulation results in cell death and rather typical gross and microscopic changes. The gross changes are seen as whitening or blanching of tissue (Fig. 10.17A). Microscopically, the cells and nuclei are distorted (Fig. 10.17B). The stromal tissues take on a deeper eosinophilic stain. Blood vessels are thrombosed. Tissue sloughing occurs subsequently over a variable period of time following the acute event.
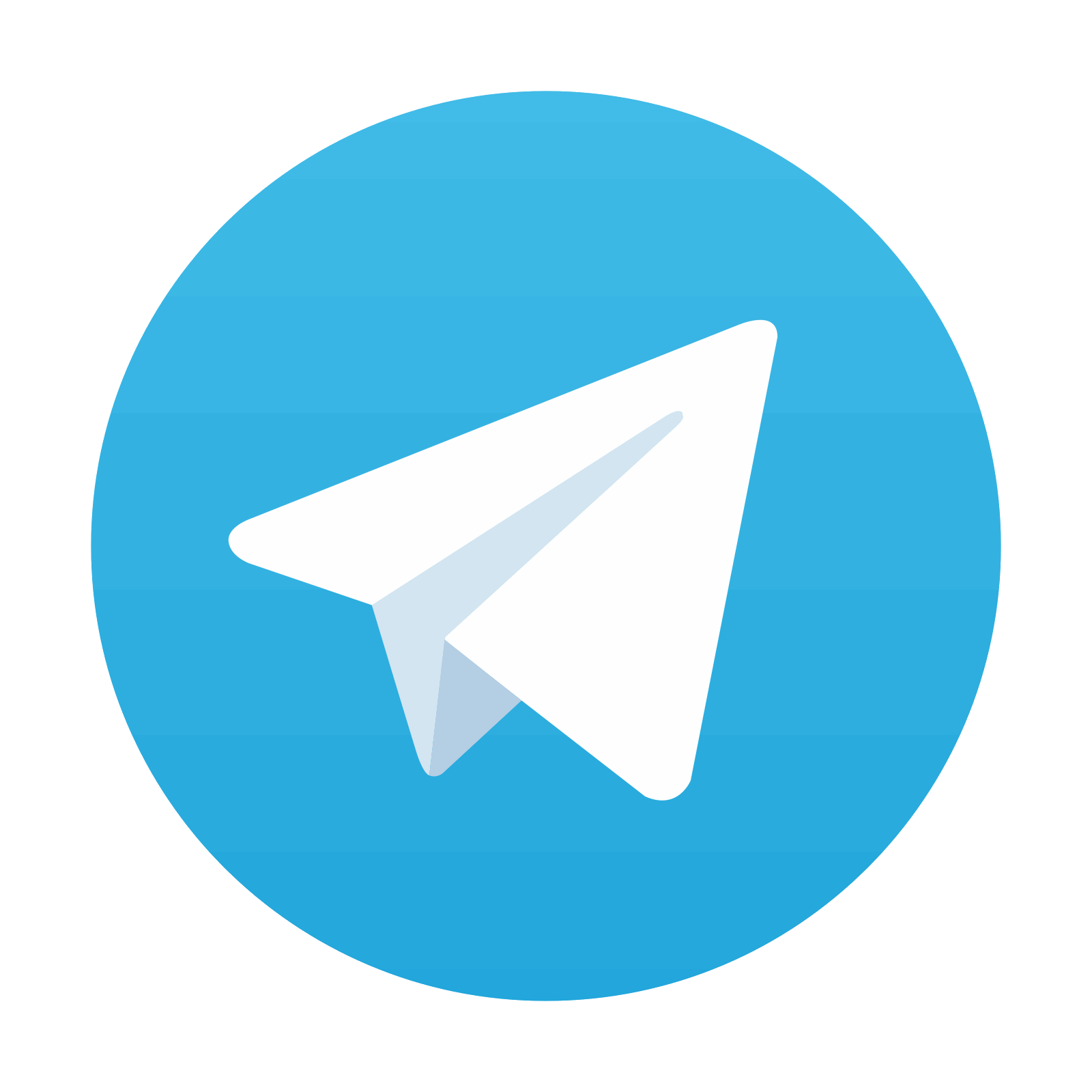
Stay updated, free articles. Join our Telegram channel

Full access? Get Clinical Tree
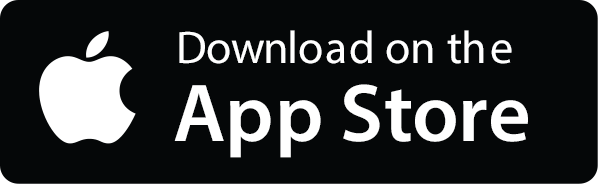
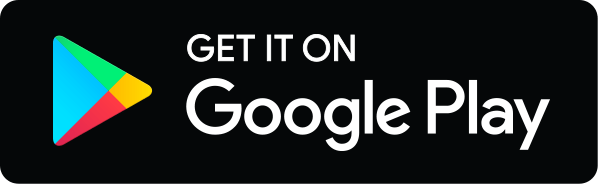