Fig. 32.1
Normal ultrasound. (a) Normal gray scale ultrasound obtained in the long axis through the renal transplant. The renal sinus is the bright central echo containing vessels and renal pelvis. It is surrounded by the gray hypoechoic cortex (arrow) with darker pyramids (arrowheads) at the corticomedullary junction. (b) Longitudinal color Doppler image shows flow throughout the allograft. (c) Longitudinal color Doppler along the course of the external iliac vasculature shows patency of the arterial (arrow) and venous (arrowhead) anastomoses to the external iliac artery (EIA) and vein (EIV). (d) Color Doppler image through the renal hilum shows an arterial patch (arrow) anastomosed to the iliac artery with two patent renal arteries (arrowheads)
Morphologic evaluation is supplemented by color/power and duplex Doppler evaluation for parenchymal blood flow and patency of the renal arteries (single or multiple) and veins (Fig. 32.1b–d). Doppler ultrasound is an ideal initial modality for the detection and evaluation of vascular complications such as thrombosis, stenosis, or arteriovenous fistulae. Pediatric en bloc transplants are technically more challenging because of the smaller size of the renal arteries and veins (Fig. 32.2a, b). Normal Doppler arterial waveforms consist of continuous antegrade flow throughout the cardiac cycle with low resistance diastolic flow (Fig. 32.3). Abnormal intrarenal arterial resistance can be quantified by calculation of indices such as the resistive index (RI) and the pulsatility index (Fig. 32.3). While both indices reflect altered hemodynamics, the resistive index (RI) is the most commonly used. Normal resistive index ranges from 0.65 to 0.70 [3, 4]. RI values depend on local vascular status rather than renal function, and measurements are neither very sensitive nor specific [5, 6]. Causes of increased intrarenal resistive index include rejection, acute tubular necrosis (ATN), hydronephrosis, and vascular thrombosis. Though nonspecific for diagnosis, elevated RI values after transplantation have been shown to be associated with poor allograft and patient survival [7].
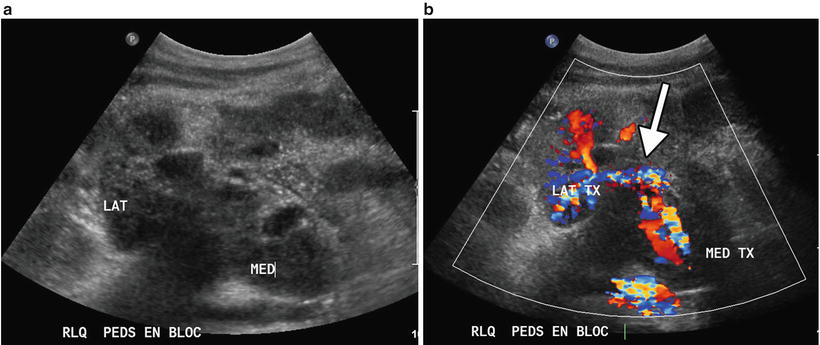
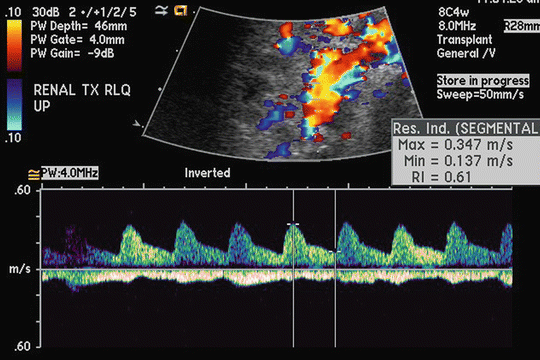
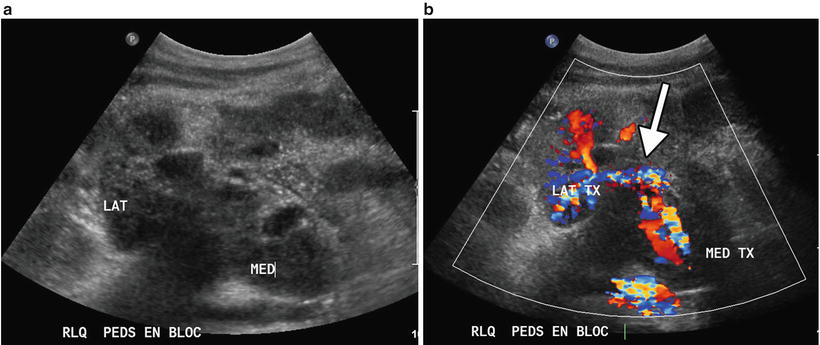
Fig. 32.2
Pediatric en bloc renal transplants. (a) Gray scale image of the two renal transplants (LAT, MED) shows the hila facing each other. (b) Color Doppler image shows the vascular anatomy with donor aorta and vena cava (arrow)
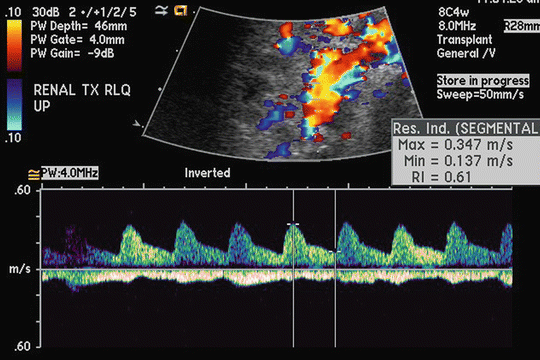
Fig. 32.3
Resistive index. Duplex Doppler tracing of an intrarenal artery demonstrates continuous antegrade, low resistance arterial flow throughout the cardiac cycle. The resistive index has been measured at 0.61
Research is ongoing in the evaluation of contrast-enhanced ultrasound using microbubble contrast agents, which is performed regularly outside of the United States, and has shown promise in the evaluation of renal transplant perfusion [8], specifically as a potential noninvasive means in the differentiation of acute rejection versus ATN as the cause of delayed graft function [9]. However, at this time, ultrasound cannot distinguish the causes of graft dysfunction, and biopsy remains the standard for diagnosis.
Ultrasound is a real-time, rapidly performed modality that is widely available and relatively cheap. It can be performed at the bedside, as is often required, and does not use ionizing radiation or nephrotoxic contrast. Limitations include suboptimal visualization in patients with large body habitus and extensive intestinal gas. Additionally, the quality of ultrasound examinations is dependent upon the technical skills and experience of the operator. Ultrasound is highly sensitive in the detection of hydronephrosis, fluid collections, and vascular complications. It is also an excellent modality for guidance during interventional procedures, such as biopsy (Fig. 32.4), aspiration or drainage of fluid collections, and access for antegrade pyelography or percutaneous nephrostomy placement.
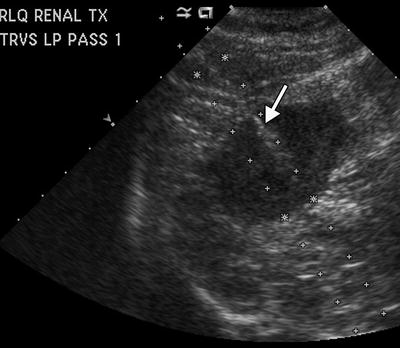
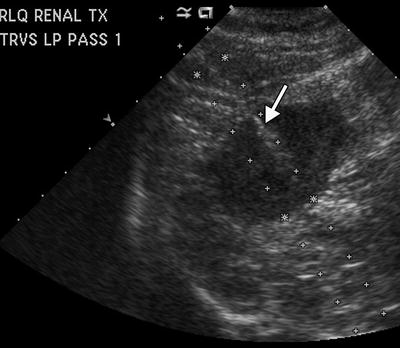
Fig. 32.4
Ultrasound-guided biopsy. The trajectory of the core needle biopsy can be seen in the lower pole peripheral cortex (arrow)
Computed Tomography
Computed tomography (CT) utilizes ionizing radiation to produce cross-sectional images with high spatial resolution. In general, computed tomography can be performed in the preoperative assessment of potential recipients and postoperatively for symptoms and signs outside of the renal transplant, e.g., fever, leukocytosis, or gastrointestinal complaints. An optimal CT examination requires iodinated contrast administered orally and intravenously. However, iodinated intravascular contrast may cause contrast-induced nephropathy (CIN) in patients with preexisting renal impairment and diabetes, and its use should be judicious in renal transplant recipients [10, 11]. Elevation in serum creatinine after the use of contrast agents within the early postoperative period is likely multifactorial, with contributory causes including ATN, rejection, cyclosporine toxicity, and dehydration. Prehydration prior to the CT study decreases the risk of contrast nephrotoxicity but does not eliminate it [10]. Although never specifically or adequately evaluated in the renal transplant population, other prophylactic measures such as administration of the antioxidant acetylcysteine are employed by many clinicians in hopes of preventing or reducing the nephrotoxicity of contrast agents.
In the immediate postoperative period, restoration of renal function may be delayed and it is therefore our practice to withhold intravenous contrast until renal function is adequate, or the use of vascular contrast considered absolutely necessary. A stable serum creatinine below 1.5–2.0 mmol/mL [1.5–2.0 mg/dL] or eGFR above 60 mL/min is a general cutoff for administering intravenous contrast media unless clinical urgency dictates the need for contrast-enhanced studies. When indicated, a reduced dose of iodinated contrast may be used. For most postoperative indications such as fever, pain, and diarrhea, an adequate CT study can be obtained without intravenous contrast but preferably with oral contrast. Alternatively, a magnetic resonance study may be substituted, as is discussed later.
CT is excellent for detection and anatomic localization of fluid collections and for guidance of interventional procedures. Transplant biopsies may also be performed using CT guidance instead of ultrasound for deep or less accessible allografts. A non-contrast CT scan may be useful in evaluating for stones in the native kidney or renal allograft and ureters.
Multidetector CT cystography has become the standard technique for evaluation of traumatic bladder injuries and is now standard at our institution for evaluation of bladder leaks after renal transplantation [12–14]. After a pre-contrast image acquisition, approximately 250–300 mL of diluted iodinated contrast is infused through a Foley catheter and another series of images is obtained when the bladder is distended. CT cystography is highly sensitive to small leaks and 3D multiplanar reformations increase diagnostic confidence. In parallel, CT nephrostography is extremely useful for the diagnosis of ureteral leaks and strictures but requires the prior placement of a percutaneous nephrostomy tube.
Magnetic Resonance Imaging
Magnetic resonance imaging (MRI) is a very versatile modality. MRI can be performed in multiple planes, does not use ionizing radiation, and has high contrast resolution, making it more sensitive than CT to pathologic change within tissues. The use of varying pulse sequences enables tissue characterization (such as fluid, fat, and hematoma) and differentiation of fluid collections. Masses in the allograft and native kidneys or other sites are readily evaluated. MR can detect flowing blood in major vessels without the use of iodinated contrast. In the past, gadolinium-based contrast agents (GBCAs) were considered preferential to iodinated intravascular contrast material in the evaluation of patients with renal failure. GBCAs increased image resolution and improved detection of small vessels allowing the acquisition of high quality angiographic images noninvasively, with a lack of nephrotoxicity [15]. However, current awareness of the association of GBCAs with nephrogenic systemic fibrosis (NSF) in patients with reduced renal function has significantly impacted the use of GBCAs, requiring careful screening of patients at risk and tailoring of examinations to maximize useful information while minimizing exposure.
Non-contrast-dependent angiographic techniques based on fast spin-echo, gradient-echo, phase-contrast, and inversion-recovery principles have been developed or maximized to assess the renal transplant vasculature, including ECG-gated 3D non-enhanced magnetic resonance angiography (MRA) performed with selective inversion-prepared fast imaging with steady state free-precession (TrueFISP). This has been shown to depict the renal transplant arteries comparable to contrast-enhanced MRA [16, 17], and is used most commonly at our institution. When contrast-enhanced MRI is unavoidable, the dose and type of GBCA should be carefully selected to minimize risk, as recent studies have shown that when restrictive GBCA administration guidelines based on glomerular filtration rate (GFR) are followed, the incidence of developing NSF significantly decreases [18].
Functional MRI of renal allografts is a field of active research. MR techniques such as contrast-enhanced perfusion imaging, diffusion-weighted imaging, BOLD (blood oxygen level-dependent) imaging, and arterial spin labeling are available to measure perfusion, blood oxygen level, and GFR [19–22]. This has great potential in the noninvasive diagnosis of transplant dysfunction. Newer techniques such as MR elastography which measures tissue stiffness may in future play a role in the detection and quantification of fibrosis [23]. Diffusion-weighted imaging has become increasingly useful in the detection and staging of cancer with future potential in tissue characterization and grading of neoplasms and lymphadenopathy [24].
MRI is contraindicated in patients with most pacemakers and some metallic implants such as intracranial aneurysm clips and heart valves. Patients with claustrophobia may be unable to tolerate MRI despite sedation. Other disadvantages of MRI include the cost, availability, and relative length of the studies. Many of the newer sequences are breath held and require patient cooperation. Artifacts from surgical clips and stents may degrade image quality and cause spurious stenoses [25, 26].
Nuclear Scintigraphy
Nuclear scintigraphic renal studies are of value for the assessment of renal allograft function. For many years, technetium 99m pentetate (DTPA) was the most frequently used radiopharmaceutical for evaluation of renal allografts. In the last decade, this agent has been replaced by technetium 99m mertiatide (MAG3). Technetium 99m MAG3 is an agent that undergoes predominantly tubular secretion, and is a more efficient imaging agent than technetium DTPA. Images are obtained over the kidney immediately after the intravenous injection of technetium MAG3 to assess perfusion of the transplant, with development of perfusion parameters for quantitative evaluation [27]. Normal, decreased, or absent allograft renal blood flow may be calculated by comparison of the intensity of radiotracer activity over the kidney with the adjacent aorta or iliac blood vessels. GFR and effective renal plasma flow (ERPF) may be calculated. However, serial studies are often necessary in the determination of abnormal renal perfusion and ideally, a baseline MAG3 study is obtained within several days of the transplantation. Study quality may be compromised by poor hydration, and evaluation of the transplant may also be limited by uptake and secretion of MAG3 within the native kidneys if these remain partially functional.
Renal transplant vascular complications may be identified during the perfusion phase of technetium MAG3 studies although these abnormalities are more optimally evaluated and detected with Doppler ultrasound techniques.
Delayed scintigraphic imaging of the renal transplant is obtained after 30 min. The secretion of MAG3 into the renal collecting system, ureter, and bladder is measured and imaged. MAG3 studies remain very helpful for the detection of urinary leak and ureteral obstruction that most often occur at the anastomosis of the transplant ureter to the recipient bladder. MAG3 studies may also be helpful in the detection of vesico-ureteric reflux. This complication is frequently seen in transplanted kidneys due to the absence of a sphincter at the ureterovesical junction. Radiotracer intensity over the ureter and kidney may alternate over time due to periodic reflux of radiotracer-labeled urine from the bladder into the ureter and renal pelvis.
Isotope renography can be used to investigate renal dysfunction. In ATN, perfusion is normal but excretion is delayed and decreased. With rejection both perfusion and excretion are abnormal. However since other causes of graft dysfunction may have a similar picture, isotope renography is not of major value for the detection of rejection in transplant kidneys.
Interventional Radiology
While conventional catheter-based angiography remains the gold standard for the diagnosis of arteriovenous fistulae, pseudoaneurysms, and renal artery or vein stenosis, in practice, vascular abnormalities are usually initially detected by Doppler ultrasound and may be confirmed by MR or CT angiography. Instead, conventional angiography with digital subtraction techniques is reserved for confirmation of suspected abnormalities immediately prior to percutaneous transcatheter interventions such as angioplasty and stent placement in transplant artery stenosis or embolization of a fistula [28, 29]. Allograft arterial and venous thrombosis is usually diagnosed by ultrasound and the role of interventional radiology is limited to mechanical thrombectomy and catheter-directed thrombolysis in select cases [30, 31].
The limitations of conventional angiography include the relative invasiveness and the required use of nephrotoxic iodinated intravascular contrast media. To limit the nephrotoxicity of administered iodinated contrast, catheter-based angiography can be performed using low doses of low- or iso-osmolar contrast material [32], possibly in conjunction with carbon dioxide gas, which has been used as a sole intravascular agent but has poor image contrast [33, 34]. Although GBCAs have historically been used as intra-arterial angiographic contrast agents, given the emergence of NSF and the relatively high doses needed for equivalent radio-opacity, they are no longer acceptable in patients with renal dysfunction [32].
Radiography
There is a limited role for abdominal radiography, limited to evaluation of stents, foreign bodies, renal calculi, and bowel complications. Chest radiography is widely used postoperatively.
Pre-transplant Work-up
Patients with long-standing renal disease often have many comorbidities which can affect graft and patient survival. Given the high demand for, and shortage of, donated kidneys, pre-transplant screening plays an important role in detecting coexisting illnesses and to evaluate feasibility of transplantation. This evaluation typically includes complete history and physical examination, with appropriate laboratory testing and up-to-date preventative health measures including colonoscopy and/or mammograms [38]. Basic radiologic imaging, including chest radiograph and abdominal ultrasound, is usually performed with more advanced imaging dictated by historical or clinical factors.
In many centers, preoperative contrast-enhanced CT arteriography (CTA) of the abdomen and pelvis is obtained in potential recipients. Selection criteria include age >50, chronic renal disease caused by diabetes and hypertension, known history of atherosclerosis or identification of atherosclerotic calcifications on radiography, or prior transplantation [39]. Important considerations include potential need for pre-transplant nephrectomy; evaluation for preexisting renal cell carcinoma or other malignancy, particularly in the dialysis population; and evaluation for sufficient atherosclerotic plaque-free patent vasculature for vascular anastomoses. In patients already on dialysis, normal dose contrast-enhanced CTA is performed. For patients who are predialysis, the risk of CIN can be lessened by hydration, before and after contrast administration [11, 40–42]. A recent study using 50–60 % of the standard dose of contrast for CTA with pre- and post-procedural hydration did not result in development of CIN in patients not yet on dialysis [43]. Additionally, at our institution, we are evaluating the potential of low kV (80 kV) CT scanning to potentiate lower doses of intravenous contrast (less than half the standard dose of 100 cc). Oral contrast is not administered as the high density interferes with volume rendering and creation of 3D reconstructions used for evaluation and presurgical mapping of vascular calcification.
Additionally, using CT, the native urinary tract can be assessed to determine need for concurrent nephrectomy (i.e., polycystic kidneys) or the presence of malignancy, given increased risk of renal cell carcinoma in the long-term dialysis-dependent patient [44].
Post-transplant Imaging Evaluation
The use of routine postoperative imaging is institution dependent. Common indications for imaging are absent or decreased urine output, delayed graft function, rising or persistently elevated creatinine, fever, pain over the allograft, or drop in hematocrit. Hypertension or hematuria may also prompt imaging evaluation. Patients with delayed graft function may have baseline imaging studies prior to discharge, which can be useful for comparison when follow-up studies are obtained. Ultrasound is the most commonly performed modality due to its unique advantages previously described. However CT, scintigraphy, and MR are complementary modalities in the radiologic armamentarium.
Complications After Renal Transplantation
Immediate complications, occurring in the first week, are mostly related to the surgical procedure and include renal artery or vein thrombosis, hemorrhage, and ureteral edema. Nonsurgical complications consist mainly of ATN and acute rejection. Early complications occur between 1 week and 1 month and include acute rejection, urinary leak, infection, fluid collections, and vascular thrombosis. After 1 month, lymphoceles, acute or chronic rejection, ureteral strictures, renal artery stenosis, infection, cyclosporine toxicity, recurrence of renal pathology, and neoplasms form the bulk of problems likely to be encountered. Overall, the most common complications are perinephric fluid collections which occur in up to 50 % of recipients [45, 46]. General complications relating to abdominal surgery are also encountered and include postoperative ileus, bowel obstruction, venous thromboembolic disease, and infections (systemic, pulmonary, renal, or bowel in origin). CT is the most useful imaging study when searching for infection or in patients with nonspecific chest or abdominal symptoms. Ultrasound is the study of choice for extremity deep venous thrombosis but is limited in the upper mediastinum or pelvis. For suspected thoracic, pelvic, or inferior vena cava thrombus, CT with contrast or MRI is the preferred modality. Multidetector CT pulmonary arteriography is the study of choice for pulmonary embolism.
Parenchymal Complications
Parenchymal complications include rejection, acute or chronic, delayed graft function, and calcineurin inhibitor toxicity. Time of onset of graft dysfunction and measurement of calcineurin inhibitor levels may be diagnostically helpful in distinguishing these complications. However in the majority of cases with elevation of serum creatinine or decreased urine output, ultrasound with Doppler is essential to exclude vascular and urologic complications.
Rejection
Since the development of more effective perioperative multidrug immunosuppressive therapies as well as the essential elimination of hyperacute rejection with modern cross matching techniques, rejection occurs less often in the first week after surgery. However, rejection remains a frequent cause of allograft dysfunction after that time and has a cardinal impact on patient and graft survival.
Despite early claims that altered transplant echogenicity, increased corticomedullary differentiation, and other subtle gray scale changes were predictive of acute rejection, it is now generally agreed that there are no specific gray scale sonographic characteristics of acute rejection [47, 48]. Allograft swelling and elevated resistive index (RI values > 0.8) or absent diastolic flow on Doppler arterial evaluation may be observed at sonography (Fig. 32.5a, b), but these findings are neither sensitive nor specific in the acute setting [49–52]. In one study, more than 50 % of allografts with biopsy-proven rejection had normal RIs of 0.7 or less [52]. The problem is that the RI is calculated in larger arteries, such as the interlobar or arcuate artery, and is interpreted as indirect evidence of disease at the capillary level. Unfortunately, there is susceptibility to error resulting from the effect of systemic disease, such as atherosclerosis, and renal artery stenosis [53]. Since acute rejection of a kidney graft primarily involves the subcapsular capillaries, early and detailed evaluation of blood flow in this area is highly desirable [54].
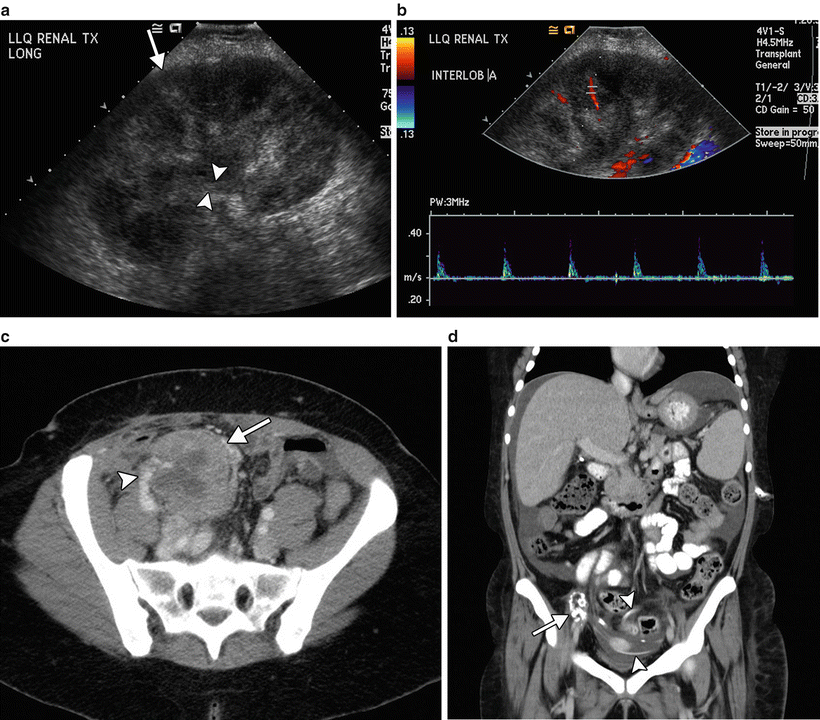
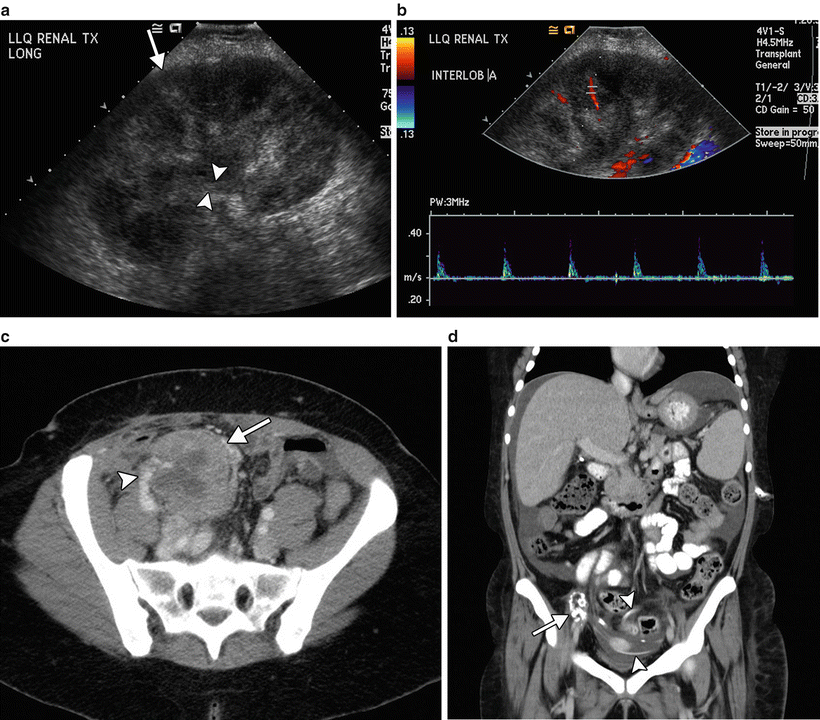
Fig. 32.5
Failed renal transplants. (a) Longitudinal gray scale ultrasound shows an enlarged, globular renal transplant with increased parenchymal echogenicity (arrow) and urothelial thickening (arrowheads). (b) Color and spectral Doppler imaging shows poor color flow and high resistance arterial waveforms with no diastolic flow. (c) Axial contrast enhanced CT through the pelvis shows an enlarged renal transplant (arrow) with patchy enhancement and patent vasculature (arrowhead), nonspecific imaging findings in a patient with biopsy proven acute on chronic rejection. (d) Coronal maximum projection-reconstructed CT image shows an atrophic calcified renal transplant (arrow) in the right lower quadrant. Also noted is a peritoneal dialysis catheter in the pelvis (arrowheads) and associated abdominopelvic ascites
Continued technical developments in US imaging and the introduction of ultrasound-specific contrast medium (microbubbles) have shown promise for the assessment and quantification of microvascular perfusion. Several studies have shown direct correlation of delayed parenchymal perfusion with pathologically proven rejection, compared to perfusion dynamics seen in patients with normal function or ATN [53–55]. However, more research needs to be done, and until ultrasound contrast medium is approved for use in the US, this work remains “in progress” [8, 9]. Therefore, at this time, the main value of ultrasound in acute transplant dysfunction is to identify ureteric obstruction or vascular complications such as ischemia or thrombosis as the underlying cause. If these potential causes of allograft dysfunction have been excluded, percutaneous biopsy with sonographic guidance is usually performed to determine the specific etiology (Fig. 32.4). Nuclear scintigraphy, CT, and MRI are not of value in this setting.
Chronic Rejection
Chronic rejection is one of the most common causes of renal allograft failure [56]. It may present after a few months, and usually is detected because of elevated serum creatinine, often with proteinuria. Ultrasound is performed to exclude structural causes of dysfunction. The allograft may be normal in size on ultrasound, but over time is likely to become progressively atrophic. A thin hyperechoic cortex with sparing of the medullary pyramids may be seen in advanced cases. As with acute rejection, Doppler arterial waveforms may be normal. However in a recent study, Doppler arterial resistive index measurements above 0.8 in a segmental branch on a single occasion more than 3 months after transplantation have been shown to be predictive of eventual graft failure [7]. Chronic rejection may be difficult to differentiate from acute rejection and cyclosporine toxicity on the basis of ultrasound findings, and unless the allograft is atrophic and calcified (Fig. 32.5d), with decreased color flow, percutaneous biopsy using sonographic guidance is usually performed to determine the cause.
Delayed Graft Function/Acute Tubular Necrosis
ATN is the most common cause of delayed graft function in the first week after surgery. Imaging is generally performed to exclude other causes of poor graft function as there are no specific imaging characteristics of ATN. Allograft swelling and elevated resistive index may be observed at sonography, but are nonspecific. As mentioned previously in the rejection section, contrast-enhanced ultrasound allows for assessment of the allograft microperfusion, and several studies have shown abnormal perfusion dynamics in patients with biopsy-proven ATN, compared to normal early graft function and acute rejection [9, 53]. Although the early results are interesting, more research is required, and biopsy remains the diagnostic gold standard [8]. At nuclear scintigraphy, relative preservation of perfusion with impaired clearance of tracer is noted [57, 58].
Vascular Complications
Up to 3 % of renal transplant recipients develop vascular complications [59, 60] with 66 % occurring within 1 month of transplantation. Early complications include renal artery or vein thrombosis, renal artery kinking or compression by collections, and hemorrhage. Renal artery thrombosis is the most common vascular cause of graft loss. After 1 month, the most common complication is renal artery stenosis. While not uncommon, biopsy-related complications such as arteriovenous fistulae are likely to resolve spontaneously and are not as clinically significant. Less common complications are renal vein stenosis and renal torsion [61].
Renal Vascular Thrombosis
Renal vein and renal artery thrombosis present with acute deterioration in graft function and urine output. Doppler ultrasound is the study of choice. An infarcted allograft will be swollen without evidence of parenchymal flow at color or spectral Doppler (Fig. 32.6a). With complete arterial thrombosis, there will be no detectable arterial flow in the allograft but a spiked preocclusive waveform may be detected in the renal artery proximal to the clot [2, 45, 62]. When there is more than one renal artery, accessory renal artery thrombosis may be suspected by failure to detect flow in all the arteries and by segmental absence or decrease of color flow in the renal parenchyma (Fig. 32.6b). Segmental infarcts may be wedge shaped and hypoechoic.
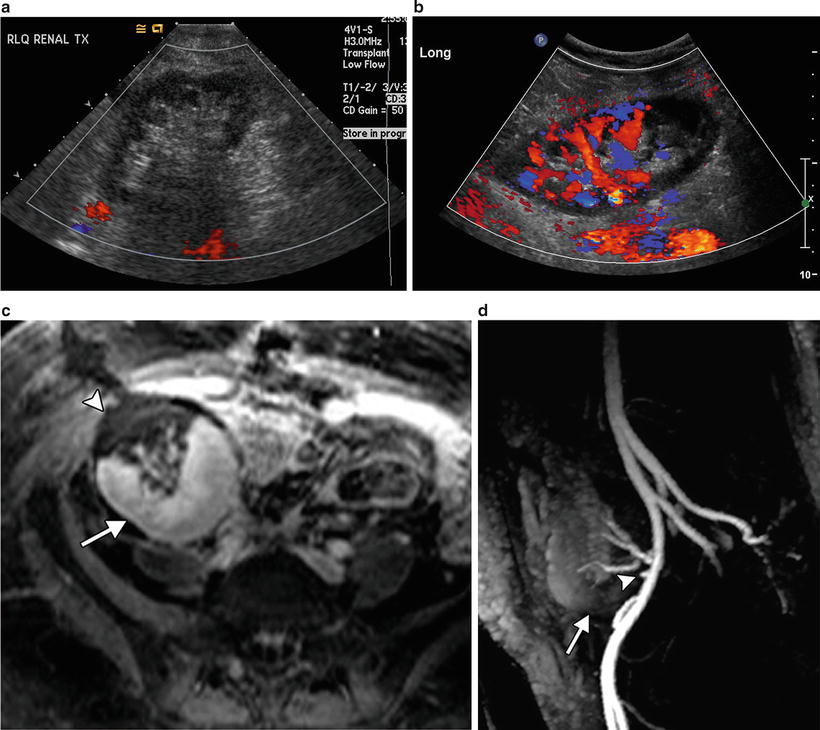
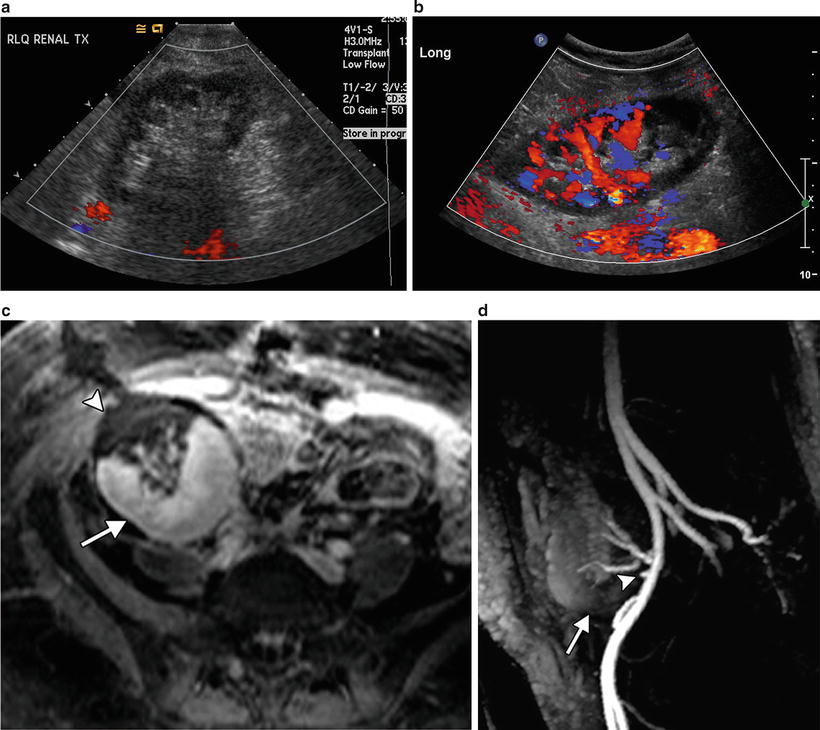
Fig. 32.6
Renal artery thrombosis. (a) Longitudinal color Doppler image shows the absence of color flow throughout the renal transplant. (b) Longitudinal color Doppler image shows segmental lower pole ischemia secondary to thrombosis of one renal artery in a patient with two renal arteries. (c) Fat suppressed, post-contrast T1 weighted MR image shows a wedge-shaped area of non-perfusion (arrowhead), with normal enhancement of the remainder of the transplant (arrow). (d) MRA shows occlusion of the inferior renal artery (arrowhead), with no enhancement of the lower pole (arrow)
In early stage venous thrombosis, the renal artery may still be patent with Doppler showing an abnormally high resistance waveform and reversal of flow in diastole (Fig. 32.7) [63, 64]. Confirmation of renal artery or vein thrombosis with other imaging modalities is rarely required and delays surgical management. There is rarely a role for interventional radiologic thrombolysis.
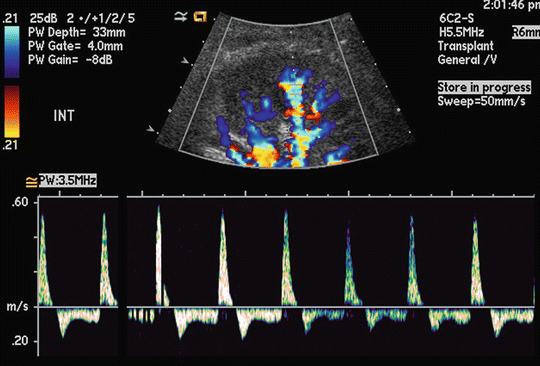
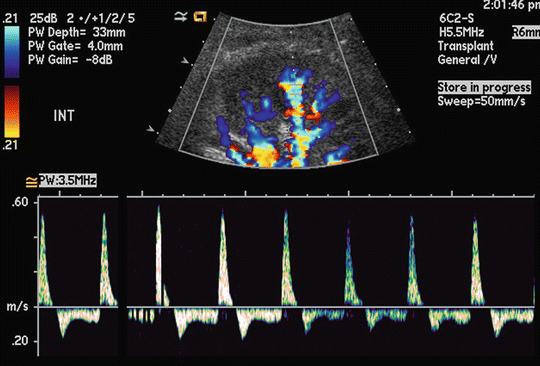
Fig. 32.7
Renal vein thrombosis. Duplex Doppler waveform shows high systolic arterial peaks with reversal of flow in diastole
Dissection of the main renal artery is rare. It is usually associated with technical intraoperative difficulties. The dissection flap will not usually be detected by ultrasound; however, decreased color flow and decreased peak velocities are indirect signs (Fig. 32.8a). CTA, MRA, or conventional angiography is superior for diagnosis (Fig. 32.8b) [65]. Treatment may be attempted by angioplasty and percutaneous stent placement (Fig. 32.8c) [65].
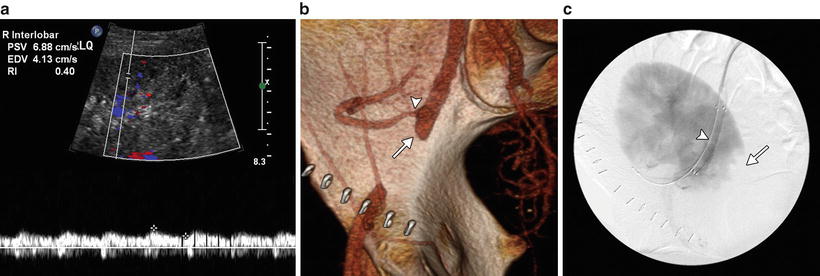
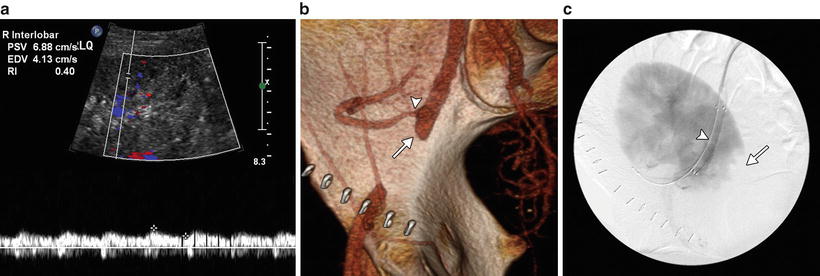
Fig. 32.8
Renal and iliac artery dissection. Doppler ultrasound shows a dampened intrarenal arterial waveform with a low resistive index of 0.40 (a). CT arteriogram shows occlusion of the EIA (arrow). There is stenosis of the transplant renal artery (arrowhead) (b). Digital subtraction image confirmed the findings which were treated with stents. Note the stent (arrowhead) and the lower pole infarct (arrows) (c)
Renal Artery Stenosis
It is not uncommon to have mild to moderate vessel narrowing at the arterial anastomosis in early postoperative period [67]. Close follow-up is recommended rather than intervention. Later, renal artery stenosis is the most common vascular complication of renal transplantation, occurring in 0.51–12 % of recipients and usually within 1 year [29, 59, 60, 68, 69]. Typical presenting features are hypertension and graft dysfunction, and occasionally a bruit. A hemodynamically significant stenosis is one which narrows the lumen by 50 %. The site of the stenosis can be at the anastomosis (in 50 %), in the donor artery, or on the recipient side. Etiology is multifactorial with contributory factors including atherosclerosis, surgical trauma and technique (e.g., torsion or angulation at the vascular anastomosis), rejection, and infection.
Ultrasound is very useful as a noninvasive screening tool. The iliac artery, renal artery anastomosis, and entire renal artery are evaluated with color Doppler for areas of altered color flow and aliasing (disturbance of color signal because of elevated velocity). Peak systolic velocity is measured at multiple sites to determine the highest peak systolic velocity. The most commonly used Doppler criteria for renal artery stenosis include an elevation of peak systolic velocity to 2–2.5 m/s (Fig. 32.9a), a velocity gradient between the stenotic and non-stenotic segments greater than 2:1, or a ratio of peak systolic velocity in the renal artery to external iliac artery (EIA) of 1.8–2 [70, 71]. In a high-risk population, these criteria achieve a sensitivity of 87–94 % and specificity of 86–100 % [45, 70, 72, 73]. However in a low risk population, the false-positive rate is high when a threshold of 2.5 m/s is used and follow-up rather than angiography should be considered [74]. Kinking and tortuosity may cause a spurious elevation of peak systolic velocity (Fig. 32.10).
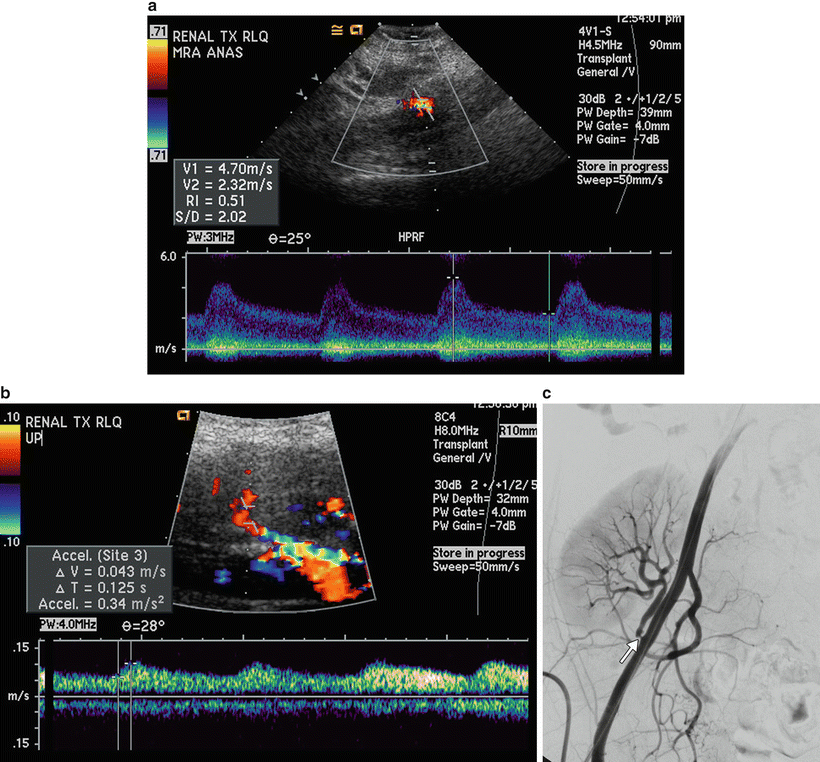
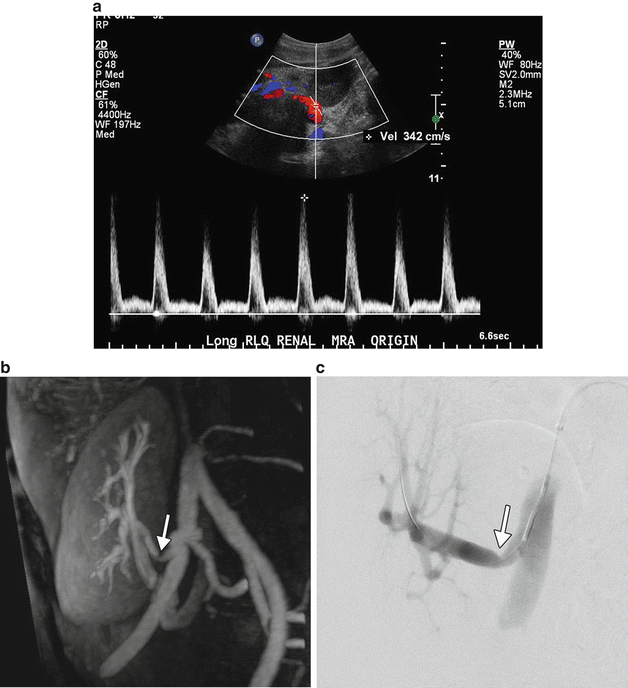
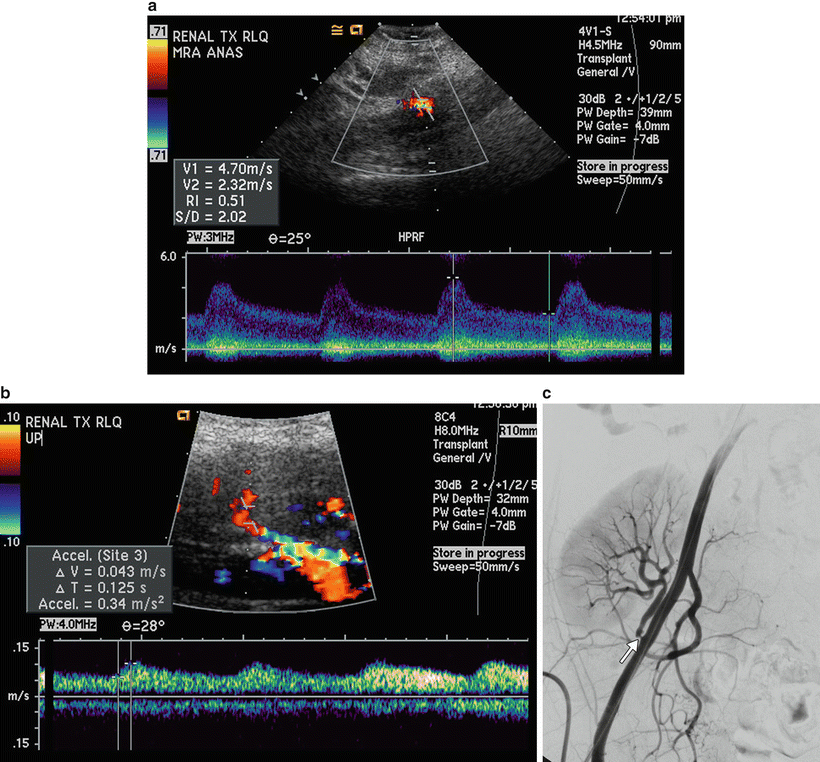
Fig. 32.9
Renal artery stenosis. (a) Duplex waveform at the renal artery anastomosis shows elevation of peak velocity, measuring 4.7 m/s. (b) Intrarenal waveform has a tardus–parvus pattern with decreased acceleration index and increased acceleration time of 0.125 s. (c) Catheter angiography confirming stenosis (arrow)
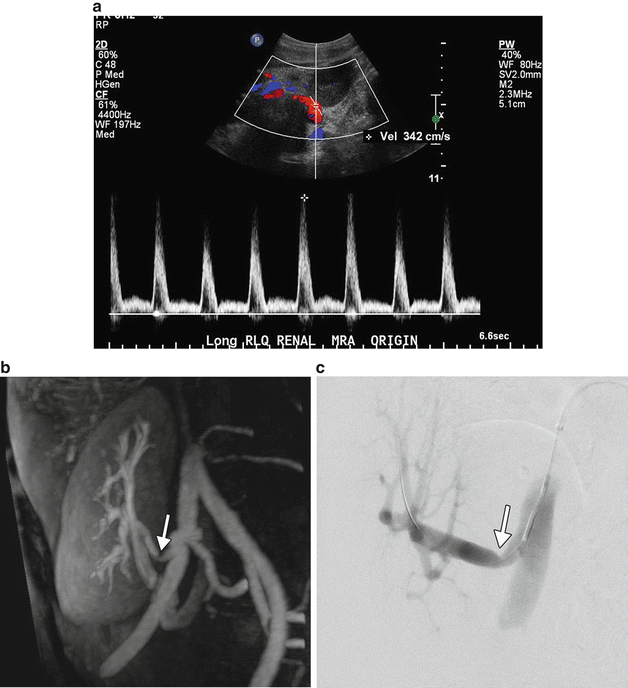
Fig. 32.10
Renal artery kink. (a) Duplex waveform shows elevation in peak velocity in the proximal renal artery, measuring 342 cm/s. (b) MRA performed without contrast shows angulation of the proximal renal artery (arrow) which was proven to be a kink (arrow) at angiography (c)
Due to technical difficulty in evaluating the renal artery anastomosis in some patients, indirect evaluation of renal artery stenosis can also be performed. Intrarenal arterial waveforms are evaluated for a delayed acceleration time (pulsus tardus) and slow rise to peak (pulsus parvus) (Fig. 32.9b). Threshold values in common use include a resistive index below 0.55 [45, 71], an acceleration time greater than 0.07–0.1 s [75]. Of note, these criteria are not applicable to pediatric transplants [76].
Catheter contrast angiography (with digital subtraction) is the reference standard for diagnosis and grading of renal artery stenosis (Fig. 32.9c). Pressure measurements can be performed to determine the significance of areas of narrowing and treatment can be performed at the same time. However angiography is invasive and requires contrast medium. Furthermore multiple injections and projections are necessary to analyze the aortoiliac segments and for tortuous or overlapping vasculature.
CT or MR contrast angiography may be performed prior to catheter angiography, in cases of non-diagnostic ultrasound or as a screening test. These modalities have the advantage of a wider field of imaging to include the entire aortoiliac and pelvic vasculature (after a single bolus of intravenous contrast) with the ability to manipulate the data in three dimensions. They are accurate in the diagnosis of transplant renal artery stenosis. Contrast-enhanced MRA is reported to have a sensitivity of 93.7 %, specificity of 80 %, accuracy of 88.5 %, positive predictive value of 88.2 %, and a negative predictive value of 88.9 % when compared to angiography (Fig. 32.11a) [15]. Pseudorenal artery stenosis from iliac stenosis and diffuse atherosclerotic disease may be detected, as well as perfusion defects and infarcts [26]. MRA may be limited by artifacts caused by certain arterial stents and metallic surgical clips. These cause signal loss and may prevent evaluation of vascular patency (Fig. 32.11b) [26]. Additionally MIP reconstruction techniques may overestimate degree of stenosis [26]. In allograft recipients with poor renal function, non-contrast MRA may be extremely helpful (Fig. 32.11c) [77–79].
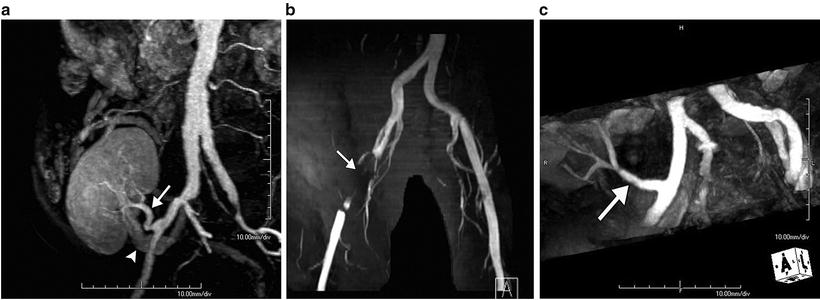
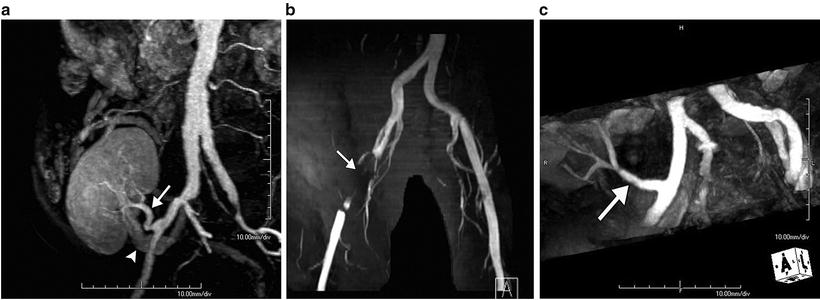
Fig. 32.11
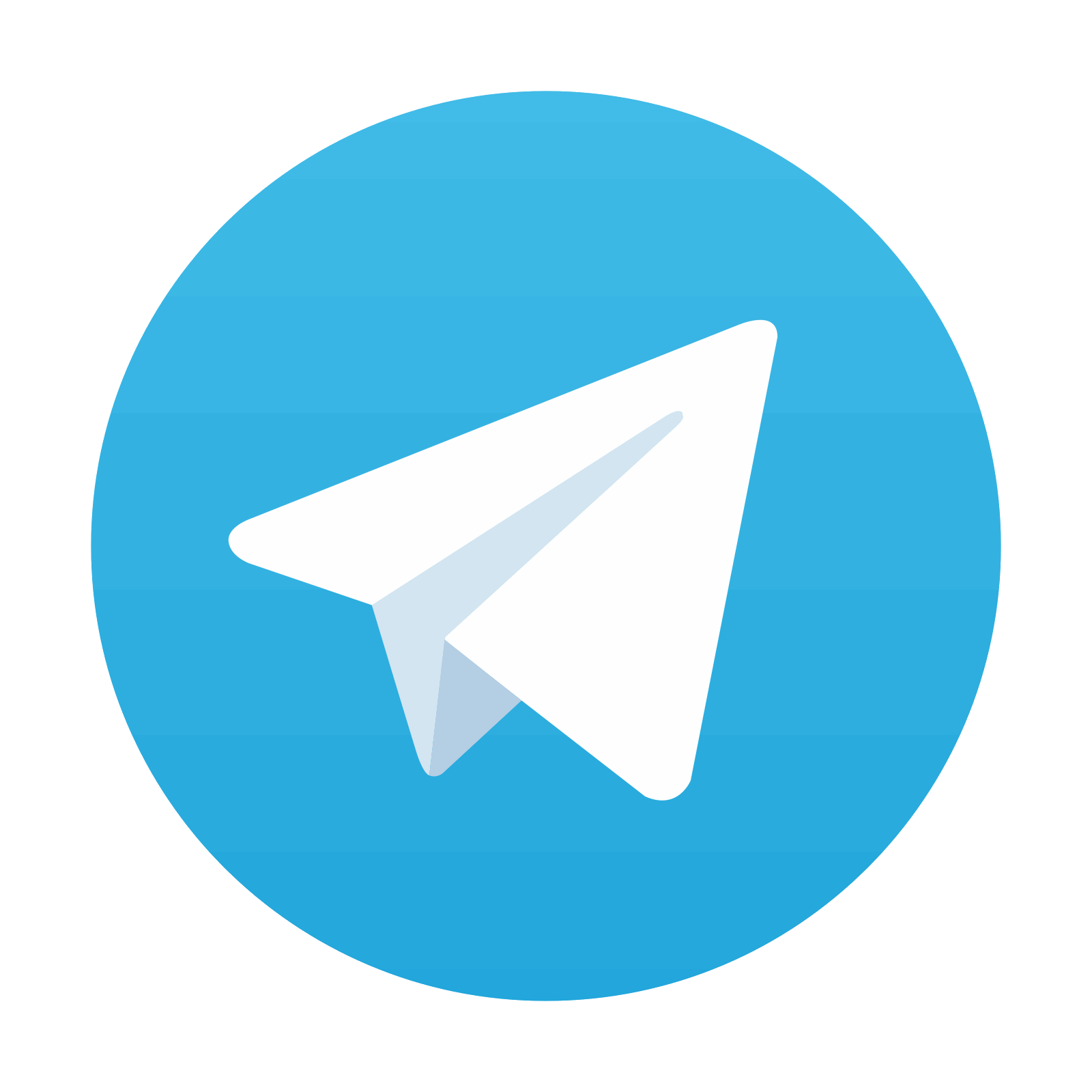
MRA. (a) Oblique reconstruction of contrast-enhanced MRA of the pelvic arterial supply demonstrates a normal renal artery (arrow) with minimal aortoiliac atherosclerosis. Incidentally noted is opacification of the main renal vein (arrowhead). (b) Contrast-enhanced MRA performed after stenting of iliac artery dissection. Loss of signal is noted at the level of the stent (arrow), precluding evaluation of the lumen. (c) Oblique reconstruction of non-contrast MRA of the pelvic arterial supply demonstrates a normal renal artery (arrow)
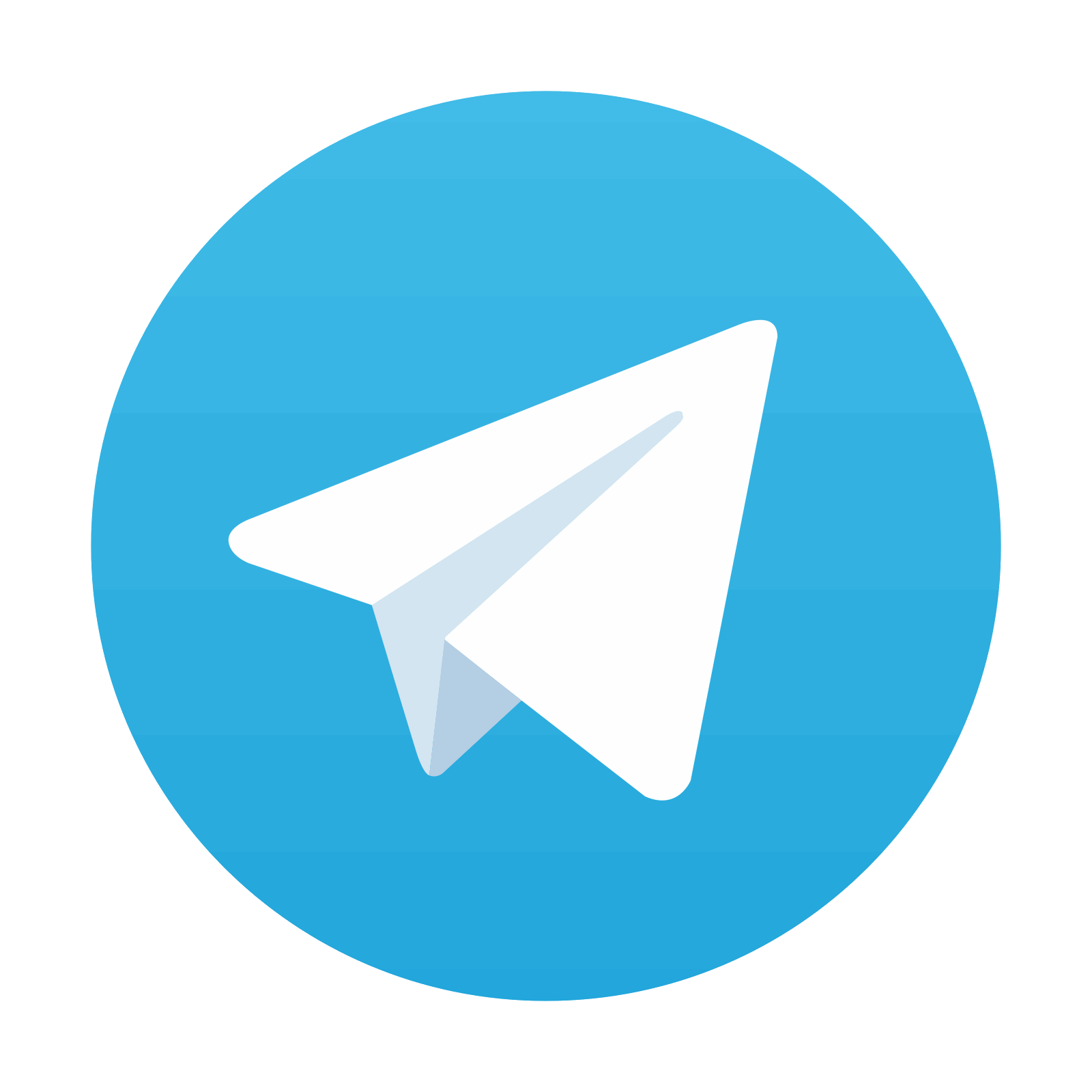
Stay updated, free articles. Join our Telegram channel

Full access? Get Clinical Tree
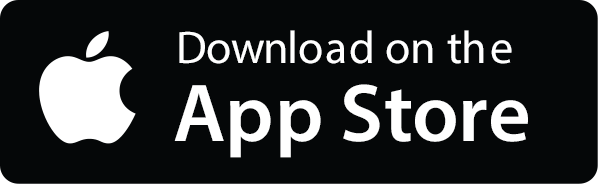
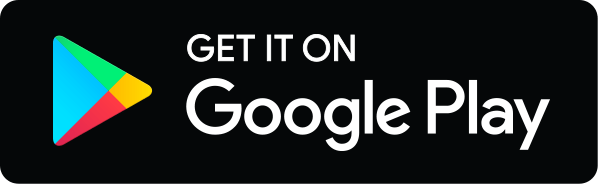
