Fig. 20.1
Normal and abnormal 24-h blood pressure monitor tracings (sleep time is designated by the bar at the bottom of each figure). (a) Represents a normal 24-h day–night blood pressure change in a healthy person being considered for kidney donation. In this case nocturnal SBP dropped by 22 % compared to the corresponding diurnal value consistent with a dipping pattern. In (b), nocturnal BP fails to drop but remains unchanged compared to diurnal value (non-dipper) and in (c), nocturnal BP is 7 % higher than daytime SBP (reverse dipper)
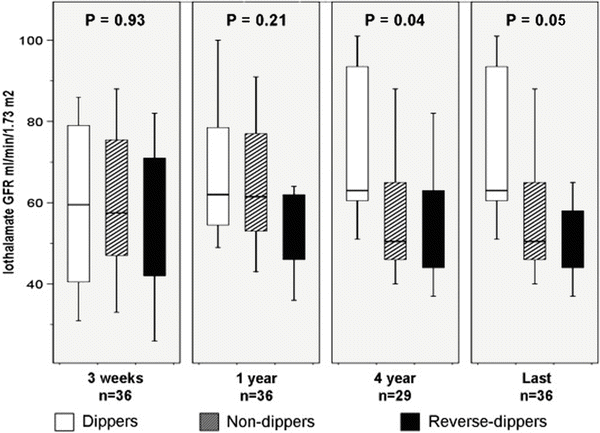
Fig. 20.2
Box plots of iothalamate GFR in 36 kidney transplant recipients without rejection at 3 weeks, 1 and 4 years and at last follow-up grouped according to the day–night dipping pattern. Despite similar GFR at 3 weeks and 1 year, non-dipper and reverse dippers had lower GFR at 4 years and at last follow-up compared to dippers (P = 0.04 and P = 0.05, respectively). Reprinted with permission [36]
When ABPM is not readily available, self-measured blood pressure is a reasonable alternative as home blood pressure readings better correlate with ABPM-derived values than blood pressure obtained in office settings [37]. Therefore, self-measured blood pressure should be encouraged in kidney transplant recipients.
Post-transplant Hypertension: Target Blood Pressure Control
No dedicated studies are available to define the optimal target blood pressure goal in the kidney transplant population, and therefore there is no universal agreement as to the BP goals of these patients. Published recommendations have been inferred from clinical trials in non-transplant populations. The KDIGO Clinical Practice Guidelines for the management of blood pressure in the patients with chronic kidney disease and following kidney transplantation have recently been updated and published [38, 39]. According to these guidelines the target goal in kidney transplant recipients is a blood pressure <130/80 mmHg with lower targets in proteinuric patients [40]. European best practice guidelines specify a target BP <125/75 mmHg in patients with proteinuria [41]. These guidelines emphasize that target BPs in kidney transplant recipients should be similar to the ones provided by the Seventh Report of the Joint National Committee on Prevention, Detection, Evaluation, and Treatment of High Blood Pressure (JNC7) [42]. Importantly, the KDIGO guidelines acknowledge that different targets should be set according to the method of blood pressure readings (office vs. home readings vs. ABPM) (Table 20.1).
Table 20.1
Adult blood pressure thresholds for defining hypertension
Method of measurement | Threshold (mmHg) |
---|---|
Office or clinic | 140/90 |
Overall ABPM average | 125a–130/80 |
ABPM daytime | 130a–135/85 |
ABPM nighttime | 120/70 |
Home | 130–135/85 |
BP control remains suboptimal in the kidney transplant population. Results from a multinational study that included 4,107 kidney transplant recipients with stable graft function after a mean of 5 years from kidney transplantation demonstrate that the target goal of BP <130/80 mmHg was not met in 69 % of participants and uncontrolled hypertension defined as BP >140/90 mmHg was present in 44 % of those taking antihypertension medication [9]. Data from the Collaborative Transplant Study that included 29,751 patients indicate that 55 % of kidney transplant patients have SBP higher than 140 mmHg and only 9.8 % have SBP less than 120 mmHg 1 year after transplantation [3]. In another study that used ABPM-derived overall average BP of <130/80 mmHg (which is similar to the target BP advocated by the KDIGO recommendations) to define adequate BP control found that only 5 % of the studied population had well-controlled hypertension despite the fact that 33 % of this cohort used three or more antihypertensive medications [33]. Some reports indicate that kidney transplant recipients are using more antihypertensive medications and have better BP control in recent years compared to historical controls [10].
Patients’ adherence, medication tolerability, medication effect on electrolyte and serum creatinine, and interactions with immunosuppression drugs are potential reasons for the limited use of BP medications that partly explain the suboptimal rate of BP control in the kidney transplant population. Another important factor to consider is the medication cost and the lack of insurance coverage. In one study that surveyed 254 US-based kidney transplant programs, 70 % of the responders reported that their patients have difficulties paying for their immunosuppression medications, reflecting the financial hardships that contribute to the inadequate blood pressure control in kidney transplant recipients [43].
Pathogenesis of Post-transplant Hypertension
Both recipient- and donor-related factors contribute to the generation and maintenance of post-transplant hypertension. Some recipient-related factors originate before the transplant while others are related to post-transplant immunosuppressive medications, delayed graft function (DGF), and other complications. Some factors that potentiate arterial hypertension differ in the early and late post-transplant periods. These include the effects of volume changes, calcineurin (CNI) inhibitors use and trough level, high-dose steroids, poor or delayed allograft function, development of proteinuria, and transplant renal artery stenosis (TRAS) [14]. Some of the major mechanisms contributing to hypertension following kidney transplantation are summarized in Table 20.2.
Table 20.2
Mechanisms that contribute to the generation and maintenance of hypertension following kidney transplantation
Pre-transplant factors |
Increased vascular stiffness |
Vascular calcification |
Post-transplant factors |
Volume overload |
Delayed allograft function |
Immunosuppression medications |
Calcineurin inhibitors (CNIs) |
Corticosteroids |
Transplant renal artery stenosis |
Poor allograft function |
Presence of native kidneys |
ESRD patients have functional and structural alterations in the arterial walls with increased stiffness of large conduit arteries and with evidence of vascular calcification. These changes are present even in the pediatric ESRD population who has been subjected to a short course of dialysis [44, 45]. These pathologic changes persist after transplantation and contribute to post-transplant hypertension following both living and deceased donor kidney transplantation [46, 47]. For example, Bahous and colleagues showed that aortic pulse wave velocity, a correlate of aortic stiffness, is higher in 101 living donor kidney transplant recipients compared to healthy controls and is related to elevated post-transplant mean arterial blood pressure [46]. Similarly, Delahousse demonstrated that aortic stiffness directly correlates with arterial blood pressure at 3 and 12 months from transplantation in 74 cadaveric kidney transplant recipients [47]. Interestingly, aortic stiffness is affected by donor age with kidney transplant patients who receive a kidney from younger donors demonstrating lower aortic stiffness compared to pre-transplant values. The opposite occurs in those who receive a kidney from older donors [47]. This may partially explain why kidneys from older donors are associated with higher risk of post-transplant hypertension [15]. Post-transplant factors also affect arterial stiffness. For example, new-onset diabetes and elevated fasting blood sugar level are related to increased arterial stiffness in kidney transplant recipients [48, 49]. Immunosuppression regimens alter arterial elasticity, and studies indicate that aortic stiffness is lower in kidney transplant recipients maintained on sirolimus compared to those using cyclosporine [50]. Switching from CNI to everolimus is associated with stabilization of arterial stiffness in 17 stable kidney transplant recipients while pulse wave velocity continued to deteriorate in the 10 patients that continued CNI use [51].
Under normal conditions, the kidney itself regulates arterial blood pressure [52]. Multiple mechanisms are involved but reduced GFR is a major factor. Therefore it is not surprising that DGF is an important risk factor for post-transplant hypertension. With DGF, the kidney’s ability to excrete sodium is reduced with subsequent lag in daily sodium excretion, salt and water retention, rightward shift of the pressure natriuresis curve, and rise in blood pressure. Management of hypertension associated with DGF ideally would include aggressive fluid removal which in many cases requires dialysis. However, due to concerns regarding hypoperfusion and further aggravation of the existing ischemic injury to the allograft, dialysis is often delayed and if implemented fluid removal is usually limited. As a result kidney transplant recipients experiencing DGF remain in a state of volume overload even in the absence of peripheral edema and their arterial blood pressure is often difficult to control. In many cases, improvement in arterial blood pressure occurs once kidney function starts to recover.
The effects of CNI on arterial blood pressure elevation develop after renal, liver, heart, and bone marrow transplantation as well as in patients using CNI for non-transplant indications [53–56]. The mechanisms involved in CNI-induced hypertension are multifactorial and have recently been reviewed [57]. The primary hemodynamic change attributed to CNI is an increase in systemic vascular resistance due to widespread arterial vasoconstriction [58]. The mechanisms of CNI-induced vasoconstriction are complex. CNI activates the renin–angiotensin–aldosterone system through a direct effect on the juxtaglomerular cells and indirectly through renal vasoconstriction [59–61]. Both human and animal studies document increased intra-renal renin activity with cyclosporine exposure despite low circulating renin and aldosterone levels [62, 63]. Cyclosporine also augments the vasoconstrictive effect of angiotensin II [60]. CNI fosters an imbalance favoring vasoconstrictive factors, such as endothelin-1 and thromboxane A2, over vasodilator compounds, such as prostacyclin and nitric oxide [58]. The fact that experimental administration of endothelin receptor antagonists improves cyclosporine-induced hypertension and renal blood flow in animal models suggests that endothelin plays a role in CNI-induced hypertension [64, 65]. Unfortunately, an important drug interaction develops with concurrent use of cyclosporine and the endothelin receptor blockers bosentan and ambrisentan that interferes delays with hepatic metabolism of both and increases blood levels. Therefore concomitant use of CNI and endothelin receptor blockers is currently contraindicated. CNIs also cause afferent arteriolar vasoconstriction leading to reduction in the GFR and increased tubular reabsorption of sodium even after short-term exposure to the medication [66–69]. Early studies indicated that dietary sodium restriction blunts the BP changes during cyclosporine administration, leading to the designation as “salt-sensitive” hypertension [70]. Recently, mounting evidence indicates that CNI directly activates the renal sodium chloride co-transporter (NCC) inducing hypertension in a mechanism analogous to pseudo-hypoaldosteronism type II (also known as Gordon syndrome) [71, 72]. The mechanism by which CNI activates NCC is still elusive, but in normal mouse distal convoluted tubules, both calcineurin and NCC co-localize, suggesting that calcineurin has a role in regulating the NCC activity [73] (Fig. 20.3). Importantly, CNI-induced activation of the NCC is reversed by hydrochlorothiazide supporting the use of thiazide diuretics in the treatment of CNI-induced hypertension [71]. Known mechanisms involved in CNI-induced hypertension are summarized in Table 20.3.
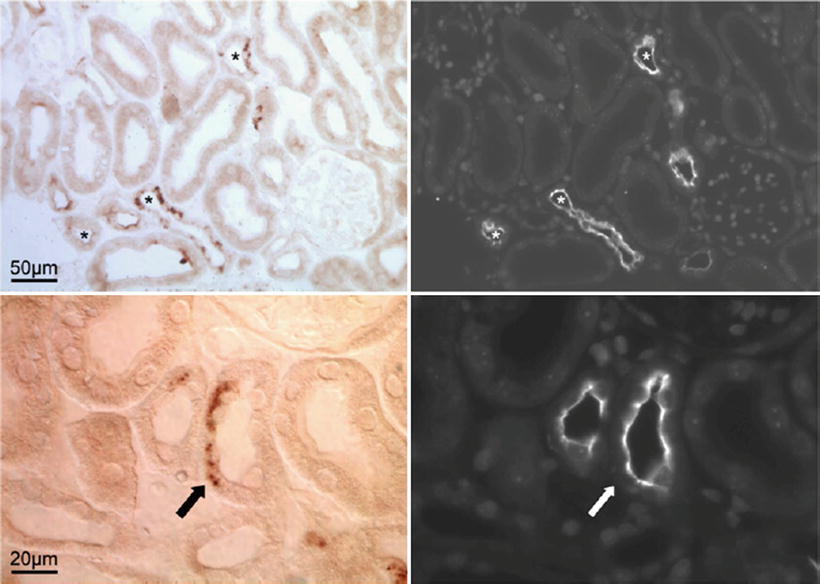
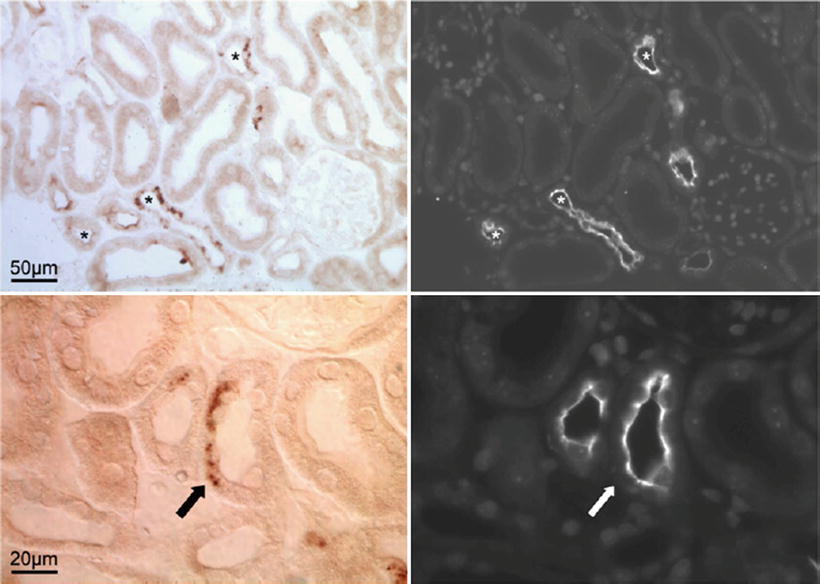
Fig. 20.3
Localization of calcineurin in the mouse kidney. Upper panel represents overview images (50 μm) of the distal convoluted tubules. The (asterisk) in the left image represents the immunohistochemical detection of calcineurin. Right image demonstrates NCC delineating distal convoluted tubules in the same field as calcineurin (asterisk). Lower panel is a detailed view (20 μm). The arrows point to a site of a partial overexpression of both calcineurin and NCC in a distal convoluted tubule. These observations support a role for facilitated tubular sodium transport as a mechanism by which CNIs such as cyclosporine accelerate hypertension. Reprinted with permission [73]
Table 20.3
Different mechanisms of calcineurin inhibitor (CNI) induced rise in blood pressure
Systemic vasoconstriction |
• Imbalance between vasoconstrictive and vasodilators |
Increase in vasoconstrictive substance: endothelin-1 and thromboxane A2 |
Decrease in vasodilator compounds: prostacyclin and nitric oxide |
• Increase intra-renal renin activity |
• Accentuation of angiotensin II action |
• Increase in sympathetic nervous activitya |
Effect on the renal allograft |
• GFR reduction |
• Increase tubular sodium reabsorption and activation of NCC |
Although both cyclosporine and tacrolimus cause systemic arterial vasoconstriction, tacrolimus has less severe and more delayed effect on increasing systemic vascular resistance and arterial blood pressure when compared to cyclosporine [55]. Allograft microperfusion visualized by contrast-enhanced sonography revealed significant vasoconstriction following cyclosporine intake, an effect that was not observed with tacrolimus and was abrogated following calcium channel medication use [74]. Aortic stiffness by pulse wave velocity is higher in cyclosporine-treated kidney transplant recipients compared to those maintained on tacrolimus [75]. Conversion from cyclosporine to tacrolimus in kidney transplant recipients is associated with modest but measurable reduction in both systolic and diastolic blood pressures which revert to their original values after cyclosporine re-exposure [76, 77]. When used as a primary immunosuppressive agent, results from multicenter studies both from the USA and Europe document a lower incidence of new-onset hypertension and less antihypertensive drug use at 5 years with tacrolimus compared to cyclosporine [78, 79]. These data suggest that tacrolimus has less pronounced effects on blood pressure as compared to cyclosporine.
Both cumulative and daily doses of steroids including bolus steroid treatment for episodes of rejection have been implicated in the rise of blood pressure, especially in the early post-transplant period [80]. Although the mechanism of glucocorticoid-induced hypertension is commonly attributed to the activation of the mineralocorticoid receptor promoting sodium and water retention, recent evidence suggests that glucocorticoid receptor-mediated activation of the vascular smooth muscles plays a role in glucocorticoid-induced hypertension [81].
The reported incidence of TRAS varies widely (from 1 to 25 %) partially due to the reliance on elevated peak systolic velocity at the anastomosis on Doppler ultrasound to diagnose TRAS [82–86]. In one study, the incidence of TRAS increased from 2.4 to 12.4 % after the introduction of Doppler ultrasound [87]. But elevated systolic velocities are not uniformly associated with hemodynamically significant TRAS. For example, only 26 out of 98 (26 %) kidney transplant recipients with elevated systolic velocity on ultrasound had angiographic evidence of TRAS [84]. When severe, TRAS-mediated hypertension can be refractory to medical treatment, can be associated with otherwise unexplained worsening allograft function, and the presence of diuretic-resistant peripheral edema may progress in some cases into pulmonary congestion and/or edema. Despite the activation of the renin–angiotensin–aldosterone system, there is little role for measuring plasma renin activity or aldosterone level since renin can be released from the native kidneys [88]. Even if TRAS is confirmed with angiography and managed with angioplasty with or without stenting, improvement in blood pressure control is not consistently achieved suggesting that TRAS may not be the reason for worsening hypertension. In one study, blood pressure control occurred in 53 % and 75 % of patients 1 and 3 months after angioplasty, respectively [89]. A study by Henning and colleagues showed that despite 93 % technical success rate for angioplasty, the mean dose of antihypertensive medications did not change [90]. Benefit of angioplasty needs also to be weighed against possible complications including immediate renal artery occlusion, dissection, and restenosis which can occur in up to 50 % of cases [83, 84, 91]. Taken together, these data suggest that most TRAS identified on Doppler ultrasound is rarely responsible for the elevated blood pressure in kidney transplant recipients. In some cases, worsening allograft function is the cause of poor blood pressure control and the trigger for Doppler ultrasound examination. Intervention to treat TRAS should be made on a case-by-case basis and should be offered to those with uncontrolled hypertension refractory to medications and those with signs of volume overload [92].
The role of the native kidneys in the overall prevalence of hypertension post-kidney transplantation is unknown. Native kidneys can induce hypertension via a renin-mediated mechanism or through increased sympathetic nerve activity (SNA) [88, 93, 94]. Hausberg and colleagues demonstrated that SNA level remains elevated after successful kidney transplantation and bilateral nephrectomy resulted in reduction in SNA in kidney transplant recipients to a level similar to that of healthy controls [93]. In a study by Huysmans et al., the prevalence of hypertension was lower in 92 recipients with absent native kidneys compared to 237 patients who intact native kidneys (57.8 % vs. 23.9 %, respectively, P < 0.001) [95]. Bilateral native nephrectomy leads to better blood pressure control in the majority, but not all patients, with resistant hypertension irrespective of whether the nephrectomy is performed before or after kidney transplantation [96–99]. However, native kidney removal has been used less frequently in recent decades, likely due to improved antihypertensive drug therapy and the morbidity associated with surgical nephrectomy. Laparoscopic native nephrectomy has lower rates of surgical complications and is an alternative in selected group of patients who are unresponsive to medical therapy alone [100]. Catheter-based renal denervation has been shown to be effective in controlling hypertension in the general population [101]. Whether catheter-based renal denervation of the native kidneys in transplant recipients will have an effect on arterial blood pressure comparable to the effect of bilateral nephrectomy warrants further study.
Effect of Hypertension on Transplant Outcomes and Cardiovascular Events
Both systolic and diastolic hypertension after kidney transplantation predict reduced patient and allograft survival. Each 10 mmHg increment of SBP above 140 mmHg is associated with an increased relative risk for graft failure (RR: 1.12, CI 1.08–1.15; P < 0.0001), death-censored graft failure (RR 1.17, CI 1.12–1.22; P < 0.0001), and patient death (RR 1.18, CI 1.12–1.23; P < 0.0001) [10]. These risks persist after adjusting for allograft function and rejection episodes [10]. Importantly, uncontrolled hypertension precedes graft failure by several years and the negative impact of post-transplant hypertension on transplant outcomes is evident even in recipients with functioning grafts 5 years from transplantation [10]. Results from a registry study that included 29,751 patients followed for up to 7 years demonstrate that uncontrolled hypertension at 1, 3, and 5 years from transplantation is associated with graded increase in subsequent allograft failure and death-censored graft failure irrespective of rejection episodes and antihypertensive medication use (P < 0.0001 for all time points) [3]. Single-center studies that included both deceased and living donor kidney transplant recipients confirm the independent, negative impact of uncontrolled hypertension on long-term transplant outcomes [1, 2].
Poor allograft function and uncontrolled hypertension are closely linked, and establishing a cause and an effect relationship of hypertension on poor allograft outcome isdifficult in many of the abovementioned studies. However, achieving lower SBP is associated with improved graft and patient survival 7 years after transplantation. In a study that included 24,404 primary deceased donor kidney transplant recipients, patients with uncontrolled hypertension at 1 year who were able to achieve adequate BP control at 3 years had lower risk of subsequent allograft failure than those with sustained hypertension at 3 years (RR 0.79; CI 0.73–0.86; P < 0.001) [102]. Likewise, better BP control after year 3 was associated with improved 10-year graft survival (RR 0.83; CI 0.72–0.96; P = 0.01), whereas even a temporary increase in SBP at 3 years was associated with worse graft survival (RR 1.37; CI 1.19–1.58; P < 0.001) [102].
Uncontrolled hypertension is a potent risk factor for de novo congestive heart failure (CHF) and ischemic heart disease (IHD) in transplant recipients. In a study that included 638 kidney transplant recipients followed for up to 7 years, each 10 mmHg increase in SBP above 128 mmHg was associated with adjusted RR of 1.29 for new IHD (CI 1.1–1.5; P = 001) and RR of 1.25 for de novo CHF (CI 1.08–1.44; P < 0.001) [5]. Results from an observational study predict a 2 % increase risk of fatal or nonfatal cardiovascular events for every 1 mmHg increase in SBP [4].
Management of Post-transplant Hypertension: Non-pharmacological Management of Post-transplant Hypertension
Lifestyle modifications including Dietary Approaches to Stop Hypertension (DASH) diet, exercise, moderate alcohol use, weight regulation, and smoking cessation are recommended as a first step in the management of hypertension in the general population [42]. No study yet has tested the efficacy of lifestyle modifications specifically on controlling post-transplant hypertension. Nonetheless, efforts to avoid weight gain, discontinue tobacco use, and increase exercise warrant emphasis for all kidney transplant recipients. Obstructive sleep apnea (OSA) is an underdiagnosed contributor to hypertension in the kidney transplant population. Kidney transplant recipients with OSA have higher levels of SBP despite taking more antihypertensive medications compared to those without OSA (147 ± 21 vs. 139 ± 18 mmHg, P = 0.05) [103]. Treatment of OSA has been associated with better BP control in the general population but has not been widely studied in kidney transplant recipients [104]. Considering the ubiquitous preexistent risk factors and risks of post-transplant hypertension, lifestyle changes including vigilance in diagnosing and treating OSA ought to be started simultaneously with pharmacological management.
Immunosuppressive Medications Dose Modification
Selection and dosage of immunosuppression after kidney transplantation are rarely determined primarily by BP effects but altering the immunosuppressive regimen can improve arterial blood pressure control. For many reasons, steroid-free protocols are gaining popularity and 23 % of first renal transplants were discharged after transplantation without steroids in 2004 as opposed to 5 % in 2000 [105]. This percentage further increased to 26 % in 2007 (source: www.ustransplant.org). Steroid reduction or withdrawal protocols can be associated with improvement in post-transplant blood pressure control. The results of a meta-analysis that included 34 studies and 5,637 kidney transplant recipients demonstrate that steroid avoidance and steroid withdrawal protocols are associated with somewhat lower risks for hypertension (RR:0.9, CI: 0.85–0.94, P < 0.0001) compared to patients receiving long-term steroids [106]. This salutary effect of steroid withdrawal on blood pressure control is evident irrespective of race, age, and interval from transplantation to steroid withdrawal with the largest effect on BP observed in those taking the lowest doses of cyclosporin [107–112]. For example, Hocker and colleagues reported lower prevalence of hypertension (50 % vs. 87 %, P < 0.05) in 23 pediatric kidney transplant recipients following steroid discontinuation 1 year from transplantation compared to 19 well-matched controls [112]. Another study randomized 130 pediatric kidney transplant recipients to complete steroid avoidance versus standard steroid use demonstrated lower SBP over the 3-year follow-up period in the steroid avoidance group (P = 0.017) [113]. Other studies, however, identify little beneficial effect of steroid withdrawal on post-transplant blood pressure control [114–117] especially with regimens based upon tacrolimus and mycophenolate. Results from a prospective, randomized, double-blind, placebo-controlled study by Woodle et al. that included 386 renal transplant recipients randomized to early corticosteroid elimination versus chronic corticosteroid maintenance (5 mg/day at 6 months) indicate that both systolic and diastolic BP are similar in patient with or without maintenance steroids [114]. The discrepancy in the effect of steroid withdrawal on post-transplant hypertension may be explained by the difference in study designs, lack of adjustment for antihypertensive medication use, variability in kidney function, and the different doses of maintenance steroid used in each study. For example, Savarman and colleagues report a retrospective study of 58 patients followed for 7 years that when prednisone dosage is tapered from 10 mg daily to 10 mg every other day, clinically significant improvements are seen in systolic and diastolic blood pressure and the number of blood pressure medications used but no further benefit is obtained with complete steroid withdrawal [115]. Overall, it appears that a low dose of daily steroids (5 mg/day) has little impact on long-term blood pressure control.
Johnson et al. report the effect of cyclosporine discontinuation on post-transplant hypertension in a multicenter study that included 525 kidney transplant patients on sirolimus and steroids randomized to either continue or withdraw CNI 3 months after transplantation. Blood pressures did not differ between groups at randomization. At 12 months, both systolic (139.8 vs. 133.5 mmHg, P = 0.004) and diastolic (81.3 vs. 78.6 mmHg, P = 0.027) blood pressures were lower in the cyclosporine withdrawal arm despite lower doses of antihypertensive medication (P = 0.013) [118]. Similar effects on BP reduction were evident when CNIs were withdrawn from a mycophenolate mofetil- and steroid-based regimen in patients with stable and dysfunctional allografts [119, 120]. Even 50 % cyclosporine dose reduction led to improvement in BP control and reduction in the number of blood pressure medications in stable kidney transplant recipients [121]. Newer classes of non-CNI immunosuppressive medications such as belatacept are associated with lower number and dose of antihypertensive medications when compared to cyclosporine-based regimens [122]. These studies indicate that CNI withdrawal and minimization protocols are associated with meaningful improvement in blood pressure control. These benefits must be weighed carefully, however, against the increased risk of rejection episodes [123].
Pharmacological Antihypertensive Therapy
Current guidelines do not recommend a particular class of antihypertensive medication to control post-transplant hypertension. Calcium channel blockers (CCBs), diuretics, beta-blockers (BBs), α1-blockers, angiotensin-converting enzyme inhibitors (ACE-I), and angiotensin receptor blockers (ARBs) have all been used to reduce blood pressure after renal transplantation effectively [124, 125]. Recent studies indicate that the utilization of all classes of antihypertensive medications has increased by 2–7-fold over the last decade, reflecting increased commitment by the transplant community to treat hypertension (Fig. 20.4) [126]. Choice of the appropriate antihypertensive medication should therefore be guided by patients’ comorbidities, volume status, CNI use, level of kidney function, and the presence of proteinuria. The majority of kidney transplant patients will require 2 or more antihypertensive medications to achieve adequate BP control, and therefore it will be more practical to start with a single antihypertensive medication irrespective of its class and additional medications can be added according to response of therapy and side effects from the initial medication [18, 124]. Clinicians caring for transplant recipients must be particularly aware of potential interactions between antihypertensive agents and immunosuppressives as well as other drugs used in this setting. Table 20.4 summarizes common antihypertensive medications used in the management of post-transplant hypertension with reference to potential beneficial and adverse effects.
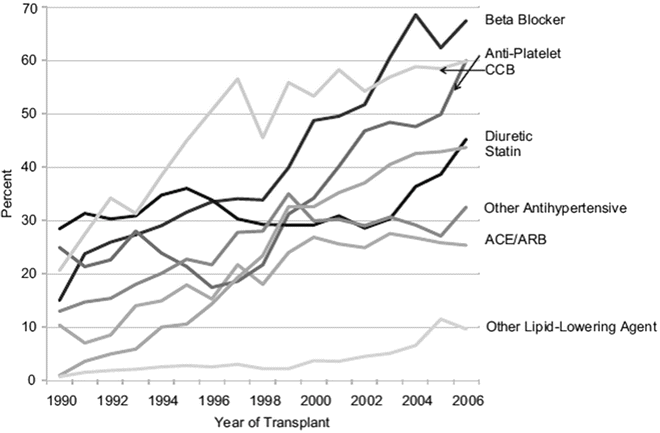
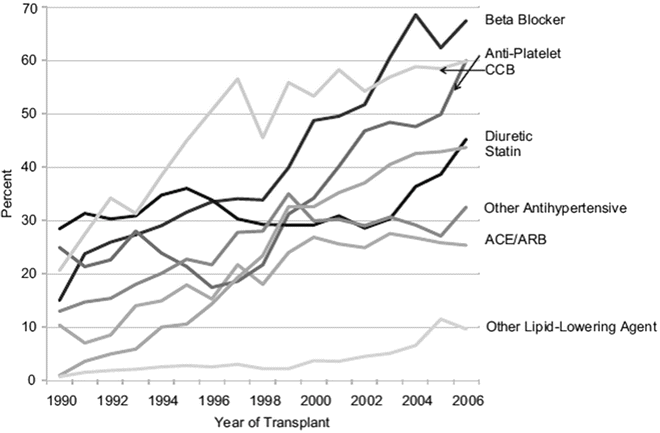
Fig. 20.4
Trend in the utilization of antihypertensive and other cardiovascular medications in the first 4 months from transplantation spanning the period from 1990 to 2006. Utilization of different antihypertensive medications has increased by 2–7-folds over this period. Reprinted with permission [126]
Table 20.4
Antihypertensive medications commonly used in kidney transplant recipients, their potential side effects and their interaction with CNIs
Class of drug | Features | Adverse effects | Effect of renal function on drug excretion | Interaction with CNI and sirolimus |
---|---|---|---|---|
Calcium channel blockers (CCBs) | ||||
Dihydropyridines | Potent vasodilators, antagonizes CNI-mediated vasoconstriction | Edema, headache, gingival hyperplasia, tachycardia | ||
Nifedipine XL | Rapid onset of action | − | − | |
Amlodipine | Steady state reached 7–8 days from drug initiation. Long 1/2 life (35 h) | − | + | |
Non-dihydropyridines | ||||
Diltiazem | Moderate potency | Bradycardia, edema | − | ++ |
Verapamil | Least potent | Constipation, bradycardia | − | ++ |
β-blockers (BBs) | ||||
Atenolol, metoprolol | Less potent antihypertensives, may diminish CNI-related headache | Bradycardia, bronchospasm, potential for rebound tachycardia | − | − |
α–β Blockers | ||||
Labetalol | Effective antihypertensives, rapid onset and short duration of action. Multiple daily dosing is required | Bradycardia, postural hypotension | + | − |
Central agents | ||||
Clonidine | Rapid onset, effective, short duration of action | Dry mouth, bradycardia, dizziness, rebound hypertension | − | − |
Enalapril, lisinopril, ramipril | Limited efficacy and short duration with enalapril. No clear benefit from starting early after transplantation. Especially useful in proteinuric patients | |||
Angiotensin receptor blockers (ARBs) Valsartan, losartan | Hyperkalemia, non-anion gap metabolic acidosis, azotemia | ++ | − | |
Diuretics | ||||
Thiazides | Potentiate other antihypertensive medications. Effective in volume-dependent hypertension. Spironolactone might have renal protective effects | Prerenal azotemia, electrolyte abnormalities | + | − |
Loop | ||||
Spironolactone |
Calcium Channel Blockers
Dihydropyridine CCBs are the most frequently prescribed antihypertensive class in kidney transplant recipients [124, 126]. As a class, CCBs effectively reduce systemic vascular resistance by acting on the vascular smooth muscle and often control post-transplant hypertension even when prescribed as monotherapy [53]. CCBs are also effective in controlling BP in nonrenal solid organ transplant patients. In a study of 37 hypertensive liver transplant recipients maintained on cyclosporine, 64 % reached goal blood pressure with long-acting nifedipine monotherapy [53]. Importantly, the fall in BP paralleled the fall in the systemic vascular resistance [53].
CCBs in general are well tolerated but commonly magnify CNI-induced gingival hyperplasia and cause lower extremity edema. Drug interactions between CNI and both non-dihydropyridine CCBs (verapamil, diltiazem) and dihydropyridine CCBs (nifedipine, amlodipine, isradipine) are well recognized and require careful monitoring of CNI levels. Non-dihydropyridine CCBs are potent inhibitors of the cytochrome P450 3A4 isoenzyme and co-administration with CNI will increase CNI trough levels [127]. Dihydropyridine CCBs produce modestly an increase in CNI levels and are considered safer than non-dihydropyridine CCBs from a drug interaction prospective [128].
CCBs counteract the systemic and afferent arteriolar vasoconstriction induced by CNI, increase renal blood flow, and increase GFR through an increase in the glomerular hydrostatic pressure and filtration fraction [129–131]. Because of these favorable hemodynamics effects, CCBs have been associated with lower risk of DGF and hyperuricemia which are commonly observed with CNI [132–135].
The effect of CCBs on kidney function and allograft survival has been extensively studied. Midtvedt et al. conducted a randomized, double-blind controlled study with 154 patients randomized to receive either lisinopril or controlled-release nifedipine. BP control was similar in both groups but patients treated with nifedipine had better allograft function that persisted during the 2-year follow-up period [136]. Similarly, results from a retrospective study that included more than 1,600 patients followed for 4 years indicate that CCB use is associated with the lowest risk of graft loss when compared to all other antihypertensive medications [124]. When patients were randomized to receive either the long-acting CCB lacidipine or placebo, lacidipine-treated patients had lower serum creatinine and higher iohexol GFR 1 and 2 years from transplantation despite having similar BP and acute rejection rate compared to the placebo arm [137]. Other randomized studies with isradipine or felodipine showed similar salutary effect of CCBs on kidney function 3–12 months after drug initiation [131, 138]. In a meta-analysis of randomized trials that included 29 studies with more than 2,200 patients randomized to either CCBs or placebo or no treatment, Cross and colleagues demonstrated that CCBs are associated with reduced graft loss (RR: 0.75, CI 0.57–0.99; P = 0.04) and higher GFR (mean GFR difference 4.5 mL/min, CI 2.2–6.7 mL/min, P < 0.0001) [139]. Taken together, results of these randomized studies suggest that CCBs may have a salutary effect on post-transplant kidney function and allograft survival as compared to placebo.
Studies demonstrating an increased GFR in cyclosporine-treated patients with CCB use generally reflect vasodilation from dihydropyridine CCB that increased intra-glomerular pressure. The increased pressure is known to increase proteinuria [130]. Whether such afferent vasodilation is beneficial for proteinuric patients over the long term is questionable. Indeed dihydropyridine CCBs accelerate proteinuria and the progression of renal disease in diabetic and nondiabetic proteinuric nephropathies [140]. Therefore caution should be used when dihydropyridine CCBs are prescribed for proteinuric patients and monitoring of urinary protein excretion is recommended in these patients.
In contrast to clear benefits in controlling post-transplant hypertension, observational single-center analyses demonstrate increased risk for ischemic cardiac events occurring beyond the first post-transplant year in kidney recipients maintained on dihydropyridine CCBs (RR, 2.26; CI, 1.24–4.12; P = 0.008), an association that persisted despite adjusting for other antihypertensive agents and known cardiac risk factors [6]. Prospective trials in other high-risk populations have not confirmed this association, although extrapolation to kidney transplant patients must be done cautiously.
Overall due to their unique properties, proven benefit, and salutary effect on graft function and survival, CCBs are considered by many including transplant work groups as the first-line antihypertensive to be used in kidney transplant recipients [141]. Caution, however, should be implemented for proteinuric patients receiving CCBs.
β-Blockers
BBs are routinely used in kidney transplant recipients with 70 % of patients transplanted in 2006 taking BBs as part of their antihypertensive regimen [126]. Many patients with preexisting cardiovascular disease who were taking BBs prior to transplantation continue taking these medications in the post-transplant period. BBs are also commonly started in the peri-transplant period to reduce the risk of perioperative cardiovascular events. BBs use in the early transplant period has more than quadrupled in recent years [9, 126].
BBs are equally effective to ACE-I in reducing blood pressure but have little impact on albuminuria [142, 143]. The antihypertensive efficacy of BBs may however be limited to patients with preserved native kidneys and was low in nephrectomized patients, attributed to low activity of the renin–angiotensin–aldosterone system in this group [144]. As a class, BBs are associated with bradycardia, fatigue, sexual dysfunction, and hyperlipidemia which are already prevalent in the kidney transplant recipients. Third-generation BBs that combine alpha- and beta-blocking properties such as carvedilol or labetalol are more potent antihypertensive agents and have limited or even beneficial effects on the lipid profile [145]. BBs use may increase the risk for development of post-transplant diabetes and new-onset type 2 diabetes in the general population [146, 147].
Renin–Angiotensin–Aldosterone System Blockers: ACE-I and ARB
Renin–angiotensin–aldosterone system (RAAS) blockers are equally effective to other antihypertensive medications in controlling post-transplant hypertension [148]. The specific potential benefits of ACE-I and ARBs in preserving allograft function in kidney transplant recipients have been the subject of multiple publications. This interest stems from the known beneficial effects of RAAS blockers on delaying the progression of kidney disease in the CKD population [149, 150]. Whether the renoprotective effects of RAAS extend to the kidney transplant population is unclear [142, 143, 151, 152]. A meta-analysis that included 14 studies suggested that use of RAAS blockers is associated with decline in GFR, an effect that persisted even after adjusting for confounding factors and including only studies that utilized RAAS blockers for more than 12 months [148]. This drop in GFR likely is explained by the hemodynamic changes that follow RAAS blockade. However the important question is whether RAAS blockers are associated with long-term protective effect on allograft function and histology. Results of a recently published double-blind randomized placebo-controlled trial that examined the effect of losartan on change in interstitial fibrosis or allograft function over a 5-year period demonstrate that neither the degree of interstitial fibrosis nor GFR was not different at any time point in the ARB compared to the placebo-treated arms (Fig. 20.5) [153]. By contrast, beneficial effects of RAAS blockers regarding limiting proteinuria are well established. Proteinuria declined with RAAS blockers by a mean of −0.47 g/day (95 % CI −0.86 to −0.08, P = 0.02). The effect of ACE-I and ARB on post-transplant proteinuria has been confirmed in other studies including a recent meta-analysis [124, 139, 142, 154]. Even in recipients without clinically significant proteinuria, ACE-I or ARB use has consistently been associated with lower urinary protein excretion rate compared to patients with similar blood pressure levels who are not taking these medications [155, 156]. Considering the negative impact of proteinuria on post-transplant outcomes, these data suggest that RAAS blockers are particularly beneficial in proteinuric kidney transplant recipients [148, 157, 158].
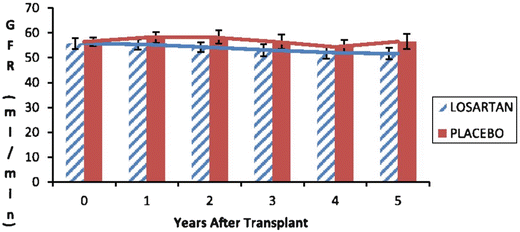
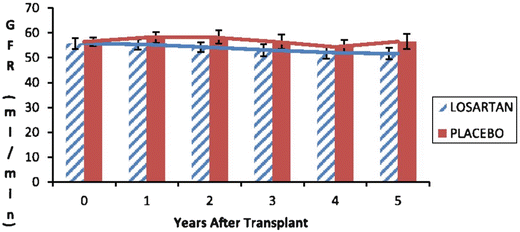
Fig. 20.5
Glomerular filtration rate (GFR) as measured by iothalamate clearance 153 kidney transplant recipients randomized to receive either losartan (n = 77) or placebo (n = 76). There was no difference in GFR at any time point between the two groups up to 5 years from transplantation. Reprinted with permission [153]
Whether RAAS blockers have specific benefits regarding patient and graft survivals has also been a matter of debate. In a retrospective study that included more than 2,000 kidney transplant recipients, RAAS blockers at any time point after the transplant were associated with improvement in both 10-year patient and death-censored graft survivals [159]. Other studies that included smaller number of patients but with higher cardiovascular risk profile showed that RAAS blocker used for a median of 14 months was associated with lower mortality but no significant advantage on allograft survival [160]. Data from the collaborative study group that included more than 17,000 patients failed to confirm a beneficial effect of ACE-I or ARB on patient or allograft survivals even when a separate analysis that included patients with increased cardiovascular risk was performed [161]. Importantly, ACE-I or ARB use does not abrogate the negative effect of post-transplant hypertension on allograft survival when graft survival was assessed at different levels of BP readings (Fig. 20.6) [161]. Comparison of RAAS blockers with CCBs in a retrospective study that included 1,662 patients identified no difference in the overall study population, but revealed improved allograft survival with RAAS blockers 4 years after transplantation in recipients with the highest tertiles of both systolic and diastolic blood pressures [124]. In patients with allograft dysfunction, some studies suggest a beneficial effect of RAAS blockers on improving graft in patients with either chronic allograft nephropathy (now identified as interstitial fibrosis/tubular atrophy, IF/TA) or post-transplant glomerular disease [162, 163]. The results of an ongoing randomized study aiming at addressing the effect of RAAS blockers on lowering patients’ mortality and prolonging allograft survival in kidney transplant recipients are currently awaited [164].