, André Smout1 and Jan Tack2
(1)
Gastroenterology and Hepatology, Academic Medical Centre, Amsterdam, The Netherlands
(2)
Gastroenterology and Hepatology, UZ Leuven, Leuven, Belgium
5.1 Normal Gastric Function
5.1.1 Control of Gastric Motility
After its passage through the esophagus, the food bolus reaches the stomach where it is temporarily stored until grinding and further aboral transport occurs.
The functions of the proximal and the distal stomach differ considerably. The proximal part comprises the fundus and the proximal half of the gastric corpus, where smooth muscle cells have a stable membrane resting potential and where contractions are slow and tonic in nature. These properties allow the proximal stomach to serve as a reservoir for the meal. In the distal part of the stomach, which comprises the antrum and the distal half of the corpus, smooth muscle cells display spontaneous oscillations of the membrane potential at a frequency of 3 cycles/min (the so-called slow waves). Slow waves are generated in the corpus near the greater curvature, in the pacemaker region of the stomach. Slow waves arise in the interstitial cells of Cajal, and they determine the rhythm at which phasic contractions in the distal stomach can occur (Fig. 5.1). Slow waves are continuously present, even when the distal stomach is not contracting. Contractions occur during the most depolarized phase of the slow waves and are triggered by the release of excitatory neurotransmitters from myenteric nerve endings.
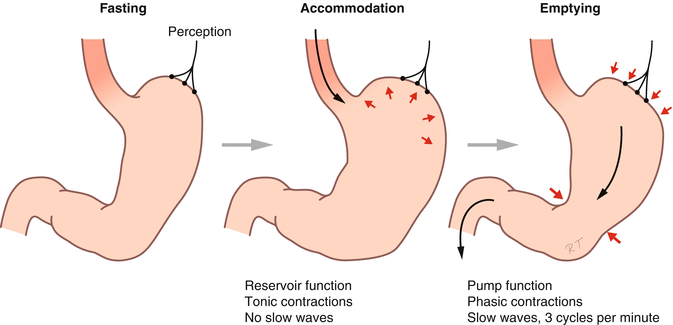
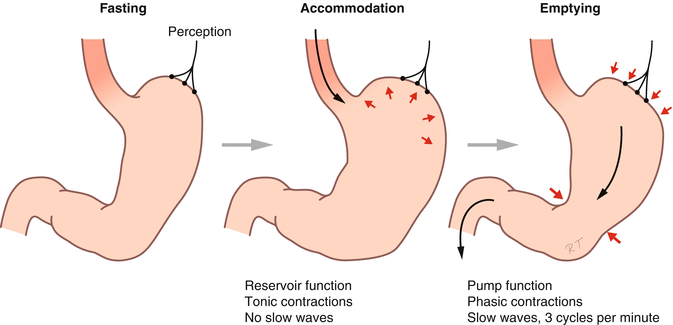
Fig. 5.1
Gastric motility during fasting, during food ingestion (accommodation), and during gastric emptying (Published with kind permission of © Rogier Trompert Medical Art 2015)
In the stomach, similar to the rest of the gastrointestinal tract, the myenteric plexus is located between the circular and the longitudinal muscle layer. Although myenteric neurons receive input from the central nervous system through parasympathetic (vagus nerve) and sympathetic autonomic nerves, the enteric nervous system is able to function independently. The vagus nerve plays mainly an afferent or sensory role, as 80–90 % of vagal nerves convey information from the gastrointestinal tract to the brain.
Afferent information from the gastrointestinal tract is integrated in the brain stem (especially the tractus solitarius) and influences myenteric plexus activity through efferent pathways. These so-called vago-vagal reflexes play a major role in the control of gastric motility, both during the fed and the fasting state. The sympathetic innervation mainly inhibits the release of acetylcholine from the myenteric plexus.
Gastric function is also controlled by a variety of gastrointestinal hormones. They mainly originate from the small intestine, where the release of many different gut peptides is controlled by the presence or absence of nutrients. These hormones signal to the brain for the control of hunger and satiety, while at the same time adapting gastric function to the presence or anticipated presence of food.
5.1.2 Fasting or Interdigestive Motility
During fasting, the stomach and small intestine exhibit a recurrent pattern of contractions and quiescence, which is called the migrating motor complex (MMC). A cycle of the MMC lasts 90–120 min and consists of 3 distinct phases (see also Chaps. 1 and 6). Phase I is a period of absent contractile activity; during phase II contractions occur at highly variable frequency and without coordination. Phase III is characterized by a period of approximately 5 min of maximal contractile frequency (3/min in the stomach), which is again followed by phase I. This contraction pattern starts proximally, in the stomach or duodenum, and migrates distally over the small intestine to start again proximally. During the passage of phase III in the gastric antrum, non-digested particles are cleared from the stomach. Phase III in the gastric antrum is also associated with an intense hunger sensation and may play a role in the control of food intake.
5.1.3 Postprandial Motility
The MMC is interrupted by food intake. Driven by the extrinsic innervation, the gastrointestinal tract switches to the so-called postprandial motility pattern. In the stomach, this is characterized by relaxation of the proximal stomach and coordinated contractions in the distal stomach (Fig. 5.1).
Following each deglutition, the lower esophageal sphincter and the proximal stomach relax to facilitate passage and arrival of the food bolus in the gastric corpus. The deglutitive relaxation of the proximal stomach, which is associated with inhibition of antral contractile activity, is called receptive relaxation. Gastric filling with a meal is not associated with a rise in intragastric pressure owing to a second type of relaxation of the proximal stomach, which is called adaptive relaxation. Both types of relaxation generate gastric accommodation, which represents the food reservoir function of the stomach, which is largely coordinated through a vago-vagal reflex pathway. As the meal becomes increasingly fragmented by gastric grinding (see below), a rise in tone of the proximal stomach occurs, which will help to promote the process of gastric emptying.
Shortly after ingestion of the meal, peristaltic contractions occur in the distal stomach, propagating from mid-corpus to the pylorus. Upon arrival of this contraction wave at the pylorus, the latter closes briefly, resulting in the food bolus being thrown back to a more proximal part of the stomach. This process helps to grind the food to small particles. As soon as these fragments are small enough, passage through the pylorus to the duodenum occurs. Hence, the pylorus functions as a filter for larger particles and thus plays a key role in the control of gastric emptying.
5.1.4 Control of Gastric Emptying
A large number of physiological mechanisms are involved in the control of gastric emptying, resulting in a different handling of liquids and solids. Liquids empty with an exponential curve, with increasing caloric and osmotic density slowing down empting. It is the pressure gradient between the stomach and the duodenum which drives the liquid emptying rate, with tone of the proximal stomach and resistance at the pylorus as the most important variables (Fig. 5.2).
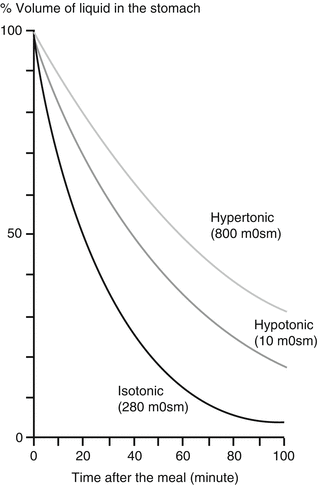
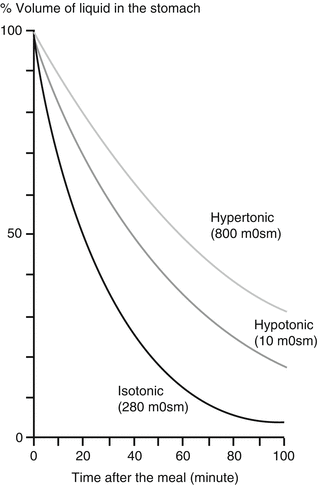
Fig. 5.2
The effect of the osmotic density of a liquid meal on the rate of gastric emptying
Emptying of solids follows a different pattern. In an initial phase, the antral peristaltic pump grinds the food, and no emptying occurs. This phase is called the “lag phase.” As soon as particles of 1 mm diameter or less are generated, passage through the pylorus occurs, and the emptying follows an almost linear curve (Fig. 5.3).
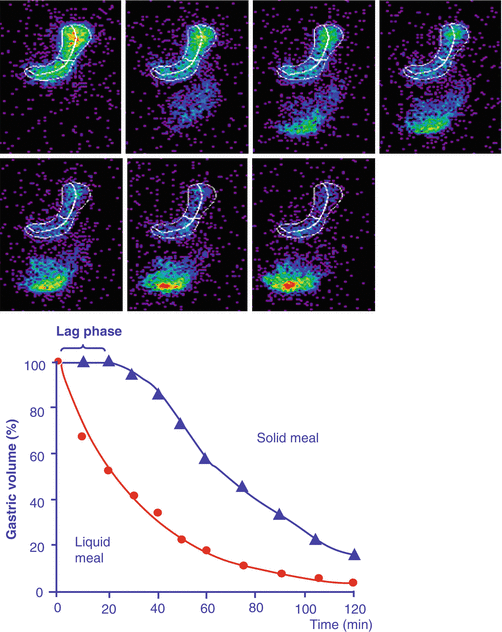
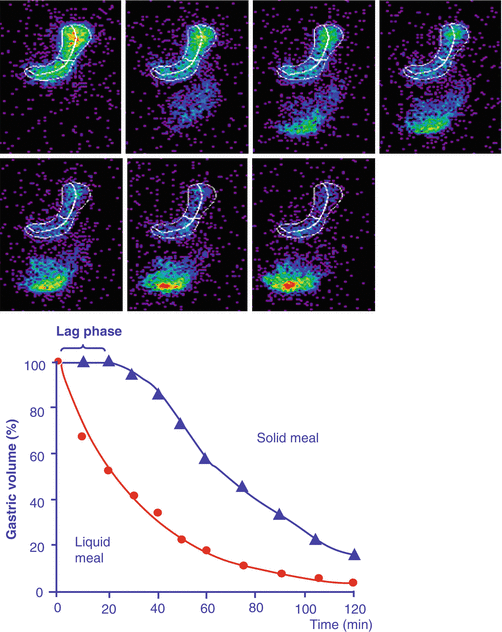
Fig. 5.3
Radioisotope gastric emptying scintigraphy, with quantification of the amount of tracer present in the stomach region over time
Pyloric passage of food occurs in a pulsatile fashion and is controlled by hormonal and neural feedback from the duodenum. Small intestinal receptors sensitive to pH, osmolarity, fatty acid chain length and concentrations of glucose, and L-tryptophan will slow gastric emptying through neural (enteric nerves) or hormonal (glucagon-like peptide 1 (Figs. 5.4 and 5.5), cholecystokinin, peptide YY, and others) signaling. In addition, there is also some colonic negative feedback, as colonic filling slows down gastric emptying.
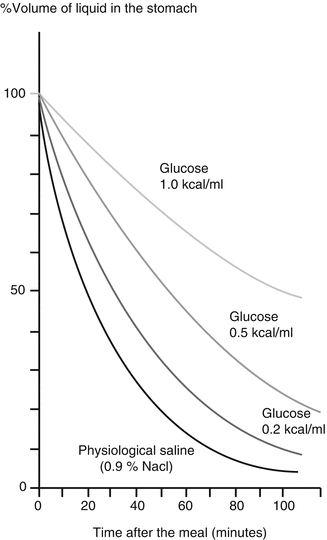
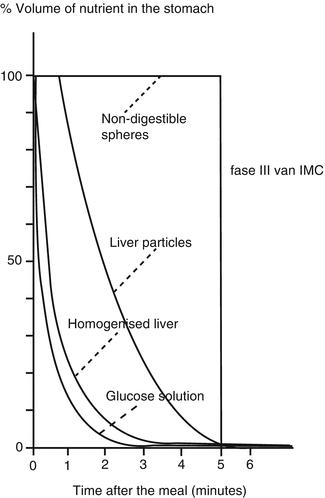
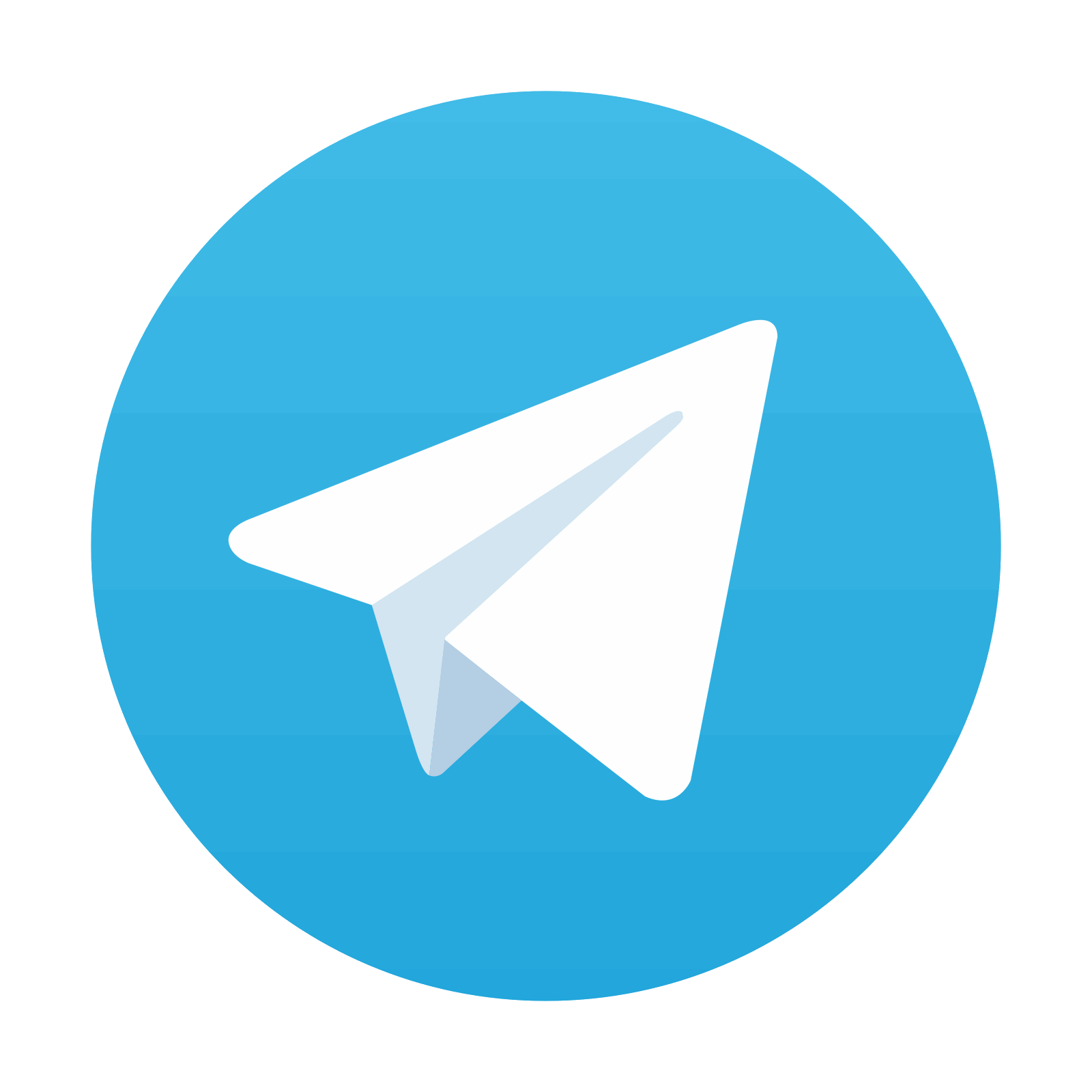
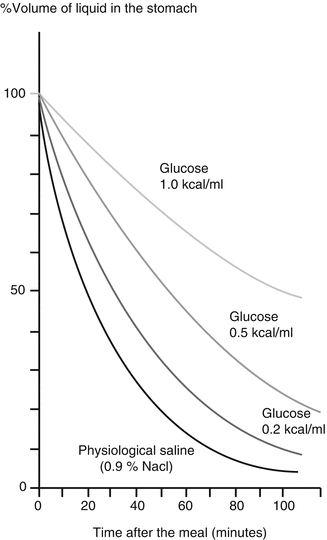
Fig. 5.4
The effect of the caloric content of a liquid meal on the rate of gastric emptying
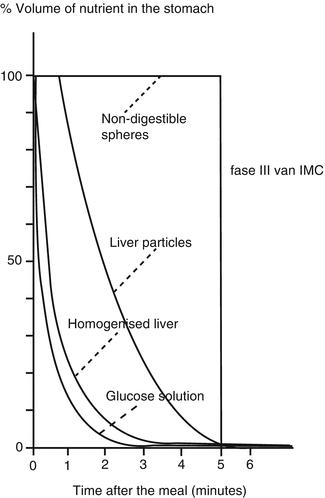
Fig. 5.5
The effect of the particle size of a meal on the rate of gastric emptying. Large particles needed to be reduced in size before they can pass the pylorus. Nondigestible particles are the last ones to exit the stomach
< div class='tao-gold-member'>
Only gold members can continue reading. Log In or Register a > to continue
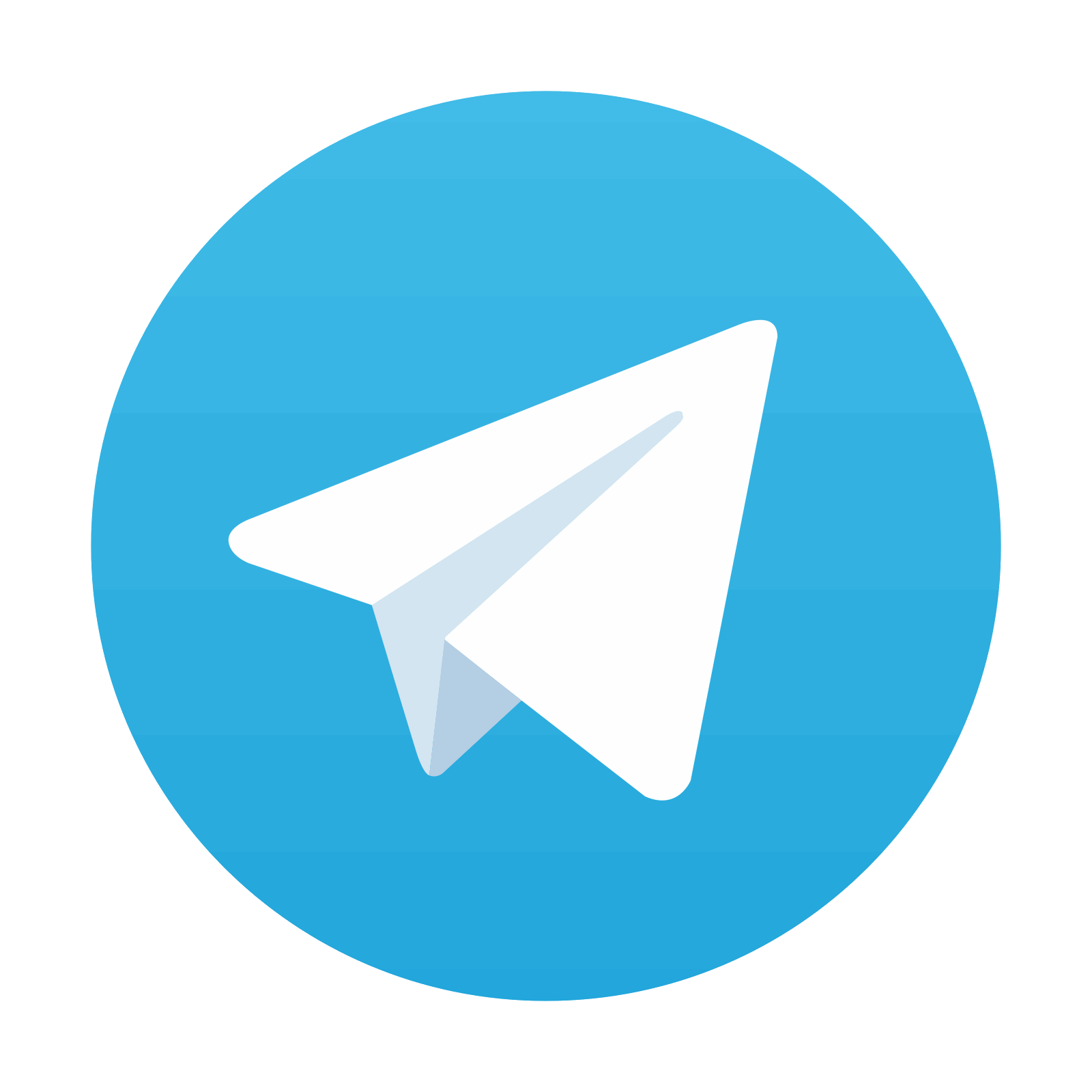
Stay updated, free articles. Join our Telegram channel

Full access? Get Clinical Tree
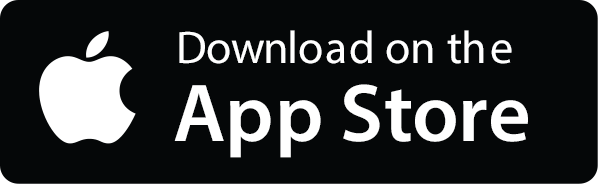
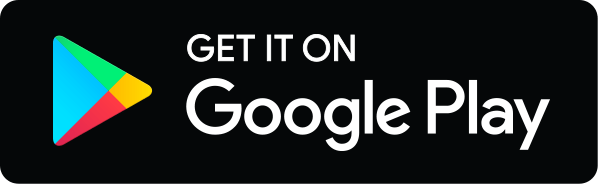
