DIALYSATE COMPOSITION IN HEMODIALYSIS
In most outpatient settings, patients receive hemodialysis using dialysate prepared in bulk and delivered via a central delivery system so that the composition of the dialysate is the same for all patients. Although most patients tolerate the procedure when administered in this fashion, many patients suffer from hemodynamic instability or symptoms of dialysis disequilibrium. One strategy to improve the clinical tolerance to dialysis is to adjust the dialysate composition according to the individual characteristics of the patient.
Dialysate Sodium
As dialysis has evolved, there has been continued interest in adjusting the dialysate sodium (Na) concentration in an attempt to improve the tolerability of the procedure. In the early days of dialysis, fluid removal was accomplished by a process of osmotic ultrafiltration. High concentrations of glucose were placed in the dialysate in order to create an osmotic driving force for fluid removal, as the coil dialyzers utilized at the time were unable to withstand high transmembrane hydrostatic pressures. In order to prevent the development of hypernatremia as water moved from plasma to dialysate, the Na concentration in dialysate was purposely set low, usually in the range of 125 to 130 mEq/L. With the development of more resilient membranes capable of withstanding high transmembrane pressures, osmotic ultrafiltration was replaced by hydrostatic ultrafiltration. Initially, a low dialysate Na concentration continued to be used in order to avoid the problems of chronic volume overload such as hypertension and heart failure. With the institution of shorter treatment times, volume removal became more rapid and symptomatic hypotension emerged as a common and often disabling problem during dialysis. It soon became apparent that changes in the serum Na concentration, and more specifically changes in serum osmolality, were playing a role in the development of this hemodynamic instability. Subsequent studies demonstrated that raising dialysate Na to between 139 and 144 mEq/L was associated with improved hemodynamic stability and general tolerance to the procedure (1,2).
The importance of a stable plasma osmolality in maintaining hemodynamic stability was first suggested when the hemodynamic profiles of ultrafiltration were compared to diffusional solute clearance. Ultrafiltration alone (the removal of iso-osmolar fluid by exerting a transmembrane pressure across the dialyzer) decreases cardiac output primarily due to a reduction in the stroke volume but is accompanied by an increase in peripheral vascular resistance such that arterial pressure is maintained. By contrast, diffusional dialysis results in a fall in arterial pressure while peripheral vascular resistance remains the same. With conventional dialysis (ultrafiltration and dialysis), less volume removal can be achieved before hypotension occurs as compared to ultrafiltration alone. The primary and characteristic difference between these two processes with respect to plasma osmolality is that plasma osmolality remains relatively constant during ultrafiltration but declines during dialysis due to the rapid intradialytic fall in plasma urea.
The decline in plasma osmolality during regular hemodialysis favors a fluid shift from the extracellular space to the intracellular space, thereby exacerbating the volume-depleting effects of dialysis. In animal studies, both conventional dialysis and sequential ultrafiltration dialysis result in an ultrafiltrate volume which is less than the decrease in extracellular fluid volume consistent with a shift of fluid into the intracellular compartment (3). In addition, during sequential ultrafiltration hemodialysis, this shift only occurs during the diffusive phase. With the advent of high clearance dialyzers and more efficient dialysis techniques, this decline in plasma osmolality becomes more apparent as solute is more rapidly removed.
Use of a low dialysate sodium concentration would tend to further augment the intracellular shift of fluid, as plasma tends to become even more hyposmolar consequent to the movement of sodium from plasma to dialysate. Older studies that employed maneuvers designed to minimize the decline in plasma osmolality such as infusing hypertonic mannitol, placement of high concentrations of dextrose in the dialysate, or placement of glycerol in the dialysate found that hemodynamic stability became comparable to that seen with ultrafiltration. While maneuvers to minimize the decline in plasma osmolarity using mannitol or glycerol can optimize hemodynamic stability during hemodialysis (4,5), the main and, perhaps the most physiologic, approach adopted to reduce the extent of change in the osmolality of body fluids during dialysis has been to increase the sodium concentration of the dialysate. Indeed, numerous investigators have now demonstrated that hemodynamic tolerance to dialysis is improved when the dialysate sodium concentration is increased to at least 135 mEq/L.
The mechanism by which raising the dialysate Na concentration improves hemodynamic stability is depicted in FIGURE 2.1. As discussed previously, a high dialysate Na concentration has been shown effective in maintaining a relatively constant plasma osmolality, thereby minimizing intracellular water movement during dialysis. In this regard, Van Stone et al. (6) measured changes in body water compartments as a function of low or high dialysate sodium concentrations in a group of dialysis patients in which 2-kg volumes were removed. With a low dialysate sodium (7% lower than serum sodium concentration), there was a dramatic decrease in extracellular water and a 21% decline in plasma volume while intracellular water increased. Use of a high dialysate sodium (7% greater than the serum sodium concentration) resulted in proportional decreases in intra- and extracellular water, and plasma volume only fell by 13%. By preventing a decrease in plasma osmolality, the higher sodium dialysate leads to mobilization of fluid from the intracellular space, resulting in better preservation of plasma volume. These findings have been confirmed by more recent studies utilizing bioimpedance measurements to study changes in body fluid compartments (7). As the dialysate-to-plasma Na gradient increases, there is a greater contraction of the intracellular fluid compartment. The shift of fluid into the extracellular fluid space increases the hydrostatic pressure of the interstitium, thus increasing the capacity for vascular refilling during volume removal resulting in a reduced frequency of hypotension and cramps.
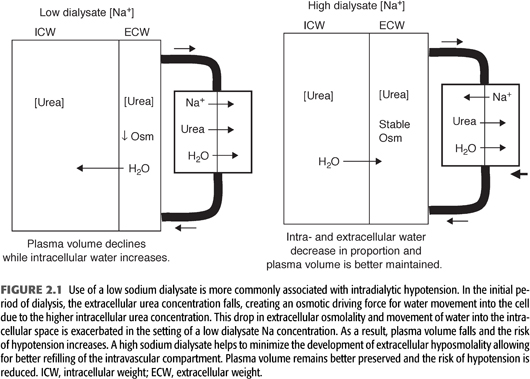
A high Na dialysate may also more favorably influence compensatory mechanisms involved in maintaining peripheral vascular resistance during volume reduction. Decreased plasma osmolality has been associated with impaired peripheral vasoconstriction during volume removal possibly due to an inhibitory effect of hyposmolality on afferent sensing mechanisms. This effect would compound the tendency toward hemodynamic instability, as many chronic dialysis patients have baseline abnormalities in autonomic function. In addition, central nervous system abnormalities due to cellular swelling are more likely to result during low osmolality dialysis, potentially further impairing autonomic function. A low Na dialysis solution has also been shown to increase prostaglandin E2 levels. This prostaglandin has venodilatory properties that could lead to venous pooling, thus contributing to hemodynamic instability (8).
The primary concern with use of a higher dialysate Na concentration is the potential to stimulate thirst and cause increased weight gain and poor blood pressure control in the interdialytic period. Studies addressing this issue confirmed that a higher dialysate Na modestly increased interdialytic weight gain. However, this excess weight was found to be readily removed with improved tolerance to ultrafiltration (1).
More recently, there has been interest in varying the concentration of Na in the dialysate during the procedure to minimize the potential complications of a high Na solution while retaining the beneficial hemodynamic effects. A high dialysate Na concentration is used initially with a progressive reduction toward isotonic or hypotonic levels by the end of the procedure. This method allows for a diffusive Na influx early in the session to prevent the rapid decline in plasma osmolality resulting from the efflux of urea and other small molecular weight solutes. During the remainder of the procedure, when the reduction in osmolality accompanying urea removal is less abrupt, the lower dialysate Na level minimizes the development of hypertonicity and any resultant excessive thirst, fluid gain, and hypertension in the interdialytic period.
Several studies have compared the hemodynamic and symptomatic effects of a dialysate in which the Na concentration is varied during the procedure to that in which the Na concentration is fixed. Dumler et al. (9) used a dialysate Na of 150 mEq/L during the initial 3 hours of dialysis at the time of ultrafiltration; the dialysate Na was decreased to 130 mEq/L for the last hour. The control group was dialyzed against Na concentration fixed at 140 mEq/L. Use of the high/low Na hemodialysis was associated with a smaller decline in systolic pressure as well as fewer symptomatic hypotensive episodes.
Other investigators have varied dialysate Na according to a Na gradient protocol in which the Na is set to decrease from a high to a low level over the course of a dialysis session. Raja et al. (10) and Daugirdas et al. (11) found no measurable benefit. Acchiardo and Hayden (12) found a reduction in hypotensive episodes, and Sadowski et al. (13) had similar results in young patients. The linear and step Na modeling programs have been found to be better in lowering the risk of intradialytic headache as compared with the exponential program. The linear program was the only individual program that alleviated interdialytic cramps; the most striking reduction in the risk for posttreatment hypotension occurred with the step program.
Differences in the incidence of symptomatic hypotension during dialysis or in the degree of interdialytic weight gain between the fixed or variable Na protocols have been difficult to demonstrate. Levin and Goldstein (14) studied a group of patients who were specifically selected because of the frequent occurrence of symptoms on dialysis such as headaches, cramps, and light-headedness. In a crossover trial, these patients were assigned either to a fixed Na dialysate and a constant rate of ultrafiltration or to a gradient protocol in which the initial Na concentration and ramping pattern were individually adjusted to minimize thirst. Use of the patient-specific Na gradient profiles was associated with improvement in all patients with headache and in 70% of patients with light-headedness. Most patients reported an increase in thirst, but there were no differences in interdialytic weight gain or in predialysis and postdialysis mean arterial pressure. Using a more general dialysis population, Sang et al. (15) compared a linear or step Na gradient (155 to 140 mEq/L) protocol with a fixed Na dialysate (140 mEq/L). In this study, Na modeling was associated with a significant reduction in cramps and symptomatic hypotension. However, these benefits were followed by increasing thirst, fatigue, and weight gain between dialysis sessions as well as a higher predialysis blood pressure. The authors concluded that only 22% of patients had a significant benefit from the modeling programs. Finally, a study by Movilli et al. (16) found improved blood volume preservation by using a pattern of high to low Na change (160 to 133 mEq/L); the changes in blood pressure were similar between this high to low variation and conventional dialysis.
In summary, the available data suggest that in most chronic dialysis patients, changing the dialysate Na during the course of the treatment offers little advantage over a constant dialysate Na of between 140 and 145 mEq/L. The inability to clearly demonstrate a superiority of Na modeling may be due to the fact that the time-averaged concentration of Na was similar in many of the comparative studies. For example, a linear decline in dialysate Na from 150 to 140 mEq/L will produce approximately the same postdialysis serum Na as occurs when a dialysate Na of 145 mEq/L is used throughout the procedure. In addition, the optimal time-averaged Na concentration whether administered in a modeling protocol or with a fixed dialysate concentration is likely to vary from patient to patient as well as in the same patient during different treatment times (17). This variability is supported by studies demonstrating wide differences in the month-to-month predialysis Na concentration in otherwise stable dialysis patients (18).
Nevertheless, in selected patients, Na modeling may be of benefit (TABLE 2.1). Patients initiating dialysis with marked azotemia are often deliberately dialyzed to decrease the urea concentration slowly over the course of several days to avoid the development of the dialysis disequilibrium syndrome. The use of a high/low Na dialysate in these patients may minimize fluid shifts into the intracellular compartment and decrease the tendency for neurologic complications. Na modeling may also be beneficial in patients suffering frequent intradialytic hypotension, cramping, nausea, vomiting, fatigue, or headache. In such patients, the modeling protocol can be individually tailored to minimize increased thirst, weight gain, and hypertension. Combining dialysate Na profiling with a varying rate of ultrafiltration may provide additional benefit in particularly symptomatic patients (19). Use of this combined approach may be of particular benefit in ensuring hemodynamic stability in patients with acute kidney injury in the intensive care unit (20).
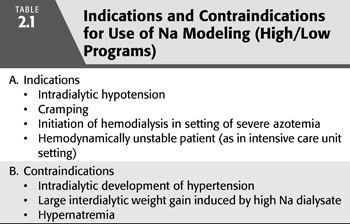
When prescribing a Na gradient protocol, it is important to monitor the patient for evidence of a progressive increase in total body Na. In some patients, a high/low Na protocol can lead to large interdialytic weight gain or cause intradialytic hypertension (TABLE 2.1). Such adverse effects are more likely to occur when the time-averaged Na concentration is greater than the patient’s predialysis serum Na concentration (21). In this setting, use of a low dialysate Na during the terminal phase of the procedure does not guarantee negative Na balance. In hypertensive patients, adjusting the protocol to achieve negative Na balance may be of therapeutic benefit in the long-term control of blood pressure. In this regard, Flanigan et al. (22) recently compared a fixed dialysate Na of 140 mEq/L to a gradient protocol in which the dialysate Na was lowered in an exponential fashion from 155 to 135 mEq/L and then held constant at 135 mEq/L for the final half hour of the procedure. Ultrafiltration was discontinued during the final half hour of the session. Use of the variable Na dialysate permitted a 50% reduction in the dose of antihypertensive medications without significant changes in predialysis blood pressure or interdialytic weight gain. Although not specifically measured, use of the terminal low Na period may have caused a decrease in the total body exchangeable Na, thus accounting for improved blood pressure control in Na-sensitive patients. A recent report from a single dialysis facility also described a beneficial effect on blood pressure when the dialysate Na was simply lowered from a set value of 141 to 138 mmol/L (23). As in the study by Flanigan et al. (22), this effect occurred in the absence of a change in interdialytic weight again consistent with a volume independent effect.
Profiled hemodialysis is a recent attempt to make the dialysate Na even more patient specific. This technique is based on a mathematical model in which baseline patient characteristics are used to construct a patient-specific Na profile dialysate before each treatment. Initial experience with this procedure has shown improved cardiovascular hemodynamics when compared with a fixed dialysate Na concentration despite the same total mass of Na being removed (24).
In dialysis patients, interdialytic Na and water loads vary from one patient to another and from treatment to treatment. Water balance can be achieved by making total ultrafiltrate volume equal to interdialytic weight gain. Current research is focusing on ways in which the dialysate Na concentration can be adjusted to more accurately match intradialytic Na removal with interdialytic Na intake. The ability to achieve zero Na balance would enhance the ability to control hypertension in the interdialytic intervals and minimize the risk of hypotension during the dialysis procedure.
The ability of dialysis to accurately regulate fluid balance has become highly refined over the years (although choosing the target dry weight and reaching it are more problematic). A similar accuracy in the maintenance of Na balance requires that the dialysate Na concentration be individualized such that with each treatment, a constant end-session plasma Na concentration is reached. If over time end-dialysis weight and plasma Na concentration are kept constant (assuming no change in Na distribution volume), one can assume that the patient will be in Na balance. As currently practiced, the dialysate Na concentrations whether fixed or varied are not chosen with the primary aim of achieving Na balance. This approach risks a pathologic excess in the total Na mass, which over time can lead to clinical manifestations of volume overload such as hypertension or congestive heart failure.
To properly calculate the dialysate Na concentration required to maintain Na balance, measurement of the patient’s plasma water Na concentration at the beginning of the treatment is required. Given the linear correlation between the conductivity of dialysate and its Na content, conductivity measurements can be used as a surrogate of Na concentration. Locatelli et al. (25,26) have recently described the use of a biofeedback system that allows for the automatic determination of plasma water and dialysate conductivity such that blood sampling can be avoided. With these measurements, along with session time, desired weight loss, and expected end-treatment plasma water conductivity, the dialysate conductivity is automatically adjusted to achieve the prescribed final plasma water conductivity. Application of this conductivity kinetic model to patients treated with a variant of hemodiafiltration has allowed for near zero hydro-sodium balance to be achieved. At the same time, cardiovascular stability was improved (25). Newer technologies capable of repetitive measurements of plasma water and dialysate conductivity will allow for the dialysate Na to be adjusted throughout the procedure (27).
With increased ability to individualize the dialysate Na concentration, one can envision a scenario in which a patient initiated on hemodialysis is initially treated with a dialysate Na concentration designed to achieve negative Na balance. Once the patient becomes normotensive or requires minimal amounts of antihypertensive medications, the dialysate Na can be adjusted on a continual basis to ensure that Na balance is maintained. However, a universal approach to prescribing the dialysate sodium relative to the serum remains controversial. Recent epidemiologic data show that, similar to the nondialysis population, lower serum sodium levels (measured predialysis) are associated with increased mortality (28). Despite the known effects on weight gain from high dialysate sodium, large dialysis cohort data show that use of a lower dialysate sodium is actually associated with further increase in mortality compared to higher dialysate sodium (29) among those patients with the lower tertile of serum sodium (<137 mEq/L). Identifying the appropriate dialysate sodium requires consideration of numerous factors including intradialytic symptoms and interdialytic habits. Achieving the optimal total body Na content will likely become just as important as determining an accurate dry weight.
Dialysate Potassium
In most chronic outpatient dialysis centers, there is little individualization of the dialysate potassium concentration. Rather, most patients are dialyzed with a potassium bath that is prepared centrally and delivered with a concentration fixed at 1 or 2 mEq/L. When using a fixed dialysate potassium, it is difficult to predict the exact amount of potassium that will be removed in a given dialysis session. Typically, one should not expect more than about 80 to 100 mEq of potassium removal even with the use of a potassium-free dialysate. In addition, there will be marked variability in the amount of potassium removed from patient to patient despite similar predialysis potassium levels and dialysis regimens. This variability can be explained by the fact that potassium movement from the intracellular to the extracellular space and ultimately into the dialysate is influenced by several patient-specific factors.
The removal of excess potassium by dialysis is achieved by the use of a dialysate with a potassium concentration lower than that of plasma creating a gradient favoring potassium removal; its rate is largely a function of this gradient. Plasma potassium concentration falls rapidly in the early stages of dialysis, but as the plasma concentration falls, potassium removal becomes less efficient. Because potassium is freely permeable across the dialysis membrane, movement of potassium from the intracellular space to the extracellular space appears to be the limiting factor in potassium removal. Factors that importantly dictate the distribution of potassium between these two spaces include changes in acid–base status, tonicity, glucose and insulin concentration, and catecholamine activity (TABLE 2.2).
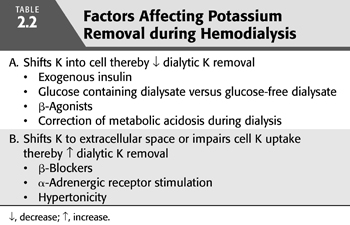
The movement of potassium between the intracellular and extracellular space is influenced by changes in acid–base balance that occur during the dialysis procedure (30). Extracellular alkalosis causes a shift of potassium into cells, whereas acidosis results in potassium efflux from cells. During a typical dialysis, there is net addition of base to the extracellular space, which promotes cellular uptake of potassium and therefore attenuates the removal of potassium during dialysis. The degree to which potassium shifts into the cell is directly correlated with the bicarbonate concentration of the dialysate (31). However, with routine dialysis, the change in blood pH is of small magnitude and the effect on potassium removal is not profound. By contrast, dialysis in patients who are acidotic will result in less potassium removal because potassium is shifted into cells as the serum bicarbonate rises. Wiegand et al. (32) described five patients in whom the serum potassium concentration decreased during dialysis even though the dialysate potassium concentration was higher than the original serum potassium concentration. The decline in potassium concentration occurred in association with a marked rise in the pH. In one patient, the decline in potassium concentration was of such a magnitude that she became quadriplegic and developed respiratory failure. There appears to be no difference in potassium removal whether acetate or bicarbonate is chosen as the dialysate buffer.
Insulin is known to stimulate the cellular uptake of potassium and can therefore influence the amount of potassium removal during dialysis. This effect of insulin was demonstrated in studies comparing potassium removal using glucose-containing and glucose-free dialysates (33). The use of a glucose-free dialysate was found to result in greater amounts of potassium removal when compared with the use of a glucose-containing bath. The use of a glucose-free dialysate would be expected to result in lower levels of insulin. However, another study showed little difference in intradialytic or postdialysis serum potassium levels between high and low glucose baths in diabetic and nondiabetic patients, suggesting that neither increased serum glucose (seen in both groups) or increased insulin (seen in nondiabetics) were sufficient to make a clinically significant change potassium removal (34).
Changes in plasma tonicity can affect the distribution of potassium between the intracellular and extracellular space. Administration of hypertonic saline or mannitol is sometimes used in the treatment of hypotension during dialysis. These agents would be expected to favor potassium removal during dialysis because the resultant increased tonicity would favor potassium movement into the extracellular space. There are no studies addressing whether there is any significant clinical benefit with this approach.
β-Adrenergic stimulation is known to shift potassium into cells and lower the extracellular concentration. Inhaled β stimulants have been reported to be effective in the acute treatment of hyperkalemia, so such therapy before dialysis may lower the total amount of potassium removed during the dialytic procedure. Allon and Shanklin (35) found that the cumulative dialytic potassium removal was significantly lower in patients who were treated with nebulized albuterol 30 minutes before the procedure as compared with patients in whom the albuterol treatment was omitted.
Alterations in serum potassium concentration during dialysis can conceivably have important effects on systemic hemodynamics. A decrease in serum potassium concentration during hemodialysis would be predicted to increase systemic vascular resistance. Hypokalemia has been shown to increase resistance in skeletal muscle, skin, and coronary vascular beds, possibly through effects on the electrogenic Na-K pump in the sarcolemmal membranes of vascular smooth muscle cells. In addition, decreased serum potassium concentration may enhance the sensitivity of the vasculature to endogenous pressor hormones (36).
Despite the potential for hypokalemia to increase systemic vascular resistance, Pogglitsch et al. (37) found the incidence of hypotensive episodes were, in fact, reduced when supplemental potassium was administered during the final 30 minutes of dialysis. One explanation for this seemingly paradoxical finding rests on the known interaction between hypokalemia and the autonomic nervous system. For example, hypokalemia has been found to be associated with dysautonomia in patients with hyperaldosteronism (38). It is reasonable to speculate that in patients with advanced kidney disease, who already have a propensity for autonomic insufficiency, a fall in plasma potassium may uncover or cause impairment in sympathetic responses (39).
In support of this suggestion, Henrich et al. (40) found that hypokalemic dialysis was accompanied by a fall in plasma catecholamine concentration as compared with dialysis in which serum potassium concentration was held constant. Moreover, despite similar reductions in blood pressure, the isokalemic dialysis group had a significant increase in heart rate after dialysis, whereas the hypokalemic group demonstrated no significant change. Further studies are needed to investigate the effects of fluctuations in serum potassium concentration during dialysis on the autonomic nervous system.
Changes in serum potassium concentration during dialysis may also influence systemic hemodynamics through effects on myocardial performance. Dialysis is associated with an increase in contractility, which can be attributed to an increase in ionized serum calcium. Increased ionized calcium is most closely related to improved ventricular contractility, but modifying effects of concomitant decreases in potassium may also be important. Haddy et al. (41) have demonstrated that the inotropic effect of increased serum calcium concentration is enhanced by simultaneous decreases in plasma potassium concentration. In this regard, Wizemann et al. (42) found that improvement in myocardial contractility during a series of isovolemic dialysis maneuvers was related to a simultaneous increase in plasma calcium and decrease in plasma potassium concentration. In the presence of an elevated plasma potassium concentration, a high plasma calcium concentration failed to exert a significant inotropic effect.
An increase in peripheral vascular resistance secondary to the development of hypokalemia could have potential detrimental effects on dialysis efficiency. This decrease in efficiency would result from decreased blood flow to urea-rich tissues such as skeletal muscle and in effect increase the amount of body wide recirculation. In support of this possibility, Dolson and Androgue (43) found that a dialysate potassium concentration of 1.0 mmol/L as compared with 3.0 mmol/L resulted in lower values for both the urea reduction ratio and Kt/V in 14 patients with ESKD. By contrast, Zehnder et al. (44) found no effect of dialysate potassium on dialysis adequacy. Although more studies are needed in this area, it is likely that any effect of a low dialysate potassium concentration to decrease dialysis adequacy is small in magnitude. In addition, increasing the dialysate potassium concentration to improve dialysis adequacy will increase the risk of hyperkalemia during the interdialytic period.
Most patients dialyzed with a fixed potassium dialysate tolerate the procedure well and do not suffer from complications of hypokalemia or hyperkalemia. Nevertheless, there are clinical conditions in which an individualized dialysate potassium concentration may be useful. Patients with underlying heart disease, particularly in the setting of digoxin therapy, are prone to arrhythmias, as hypokalemia develops toward the end of a typical treatment. Additionally, low dialysate potassium has been shown to unfavorably alter the QTc interval (a marker of risk of ventricular arrhythmias) (45).
Setting the dialysate potassium concentration to a higher value in any patient at risk for arrhythmias is not without risk. In an analysis of more than 80,000 maintenance hemodialysis patients, predialysis serum potassium values between 4.6 and 5.3 mEq/L were associated with the greatest survival, whereas values <4.0 or ≥5.6 mEq/L were associated with increased mortality. Use of a higher dialysate potassium concentration was associated with increased mortality in patients with predialysis potassium values ≥5.0 mEq/L (46). A separate case-control study found the use of a low potassium dialysate (<2.0 mEq/L) as an independent risk factor for the specific occurrence of in-center sudden cardiac arrest. Patients with cardiac arrest who were on low potassium dialysate were noted to have lower predialysis serum potassium than the control patients on low potassium dialysate (47). Altogether, when considering the risk for cardiac death or mortality, predialysis serum potassium should help guide the decision of the dialysate potassium with caution to avoid excessively high or low serum potassium levels. The use of variable dialysate potassium levels during a single treatment results in less QTc abnormalities compared to fixed levels, but it is uncertain if this would translate into overall better outcomes (48).
With these considerations in mind, Redaelli et al. (49) have studied the effects of modeling the dialysate potassium concentration in such a way as to minimize the initial rapid decline in the plasma potassium concentration (FIGURE 2.2). Patients with frequent intradialytic premature ventricular complexes were dialyzed using a dialysate with a fixed (2.5 mEq/L) potassium or one with an exponentially declining potassium (from 3.9 to 2.5 mEq/L), which maintained a constant blood to dialysate potassium gradient of 1.5 mEq/L throughout the procedure. In the fixed dialysate group, the blood to dialysate potassium gradient decreased over the treatment from 3.0 to 1.4 mEq/L. The variable potassium dialysate decreased premature ventricular complexes, a finding most evident during the first hour of the procedure. The total drop in the serum potassium concentration was no different between the fixed and variable potassium dialysates.
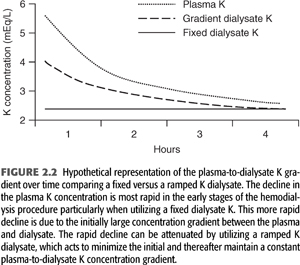
In summary, because of kinetics of potassium movement from the intracellular to the extracellular space, one can expect only up to 70 to 90 mEq of potassium to be removed during a typical dialysis session. As a result, one should not overestimate the effectiveness of the dialytic procedure in the treatment of severe hyperkalemia. The total amount removed will exhibit considerable variability and will be influenced by changes in acid–base status, changes in tonicity, changes in glucose and insulin concentration, and catecholamine activity. Given the tendency for the plasma potassium to rise in the immediate postdialysis time period, the most efficient way to remove excess potassium stores would be to prescribe 2- to 3-hour periods of dialysis separated by several hours. Studies examining the hemodynamic effect of potassium fluxes during hemodialysis are limited. More importantly, deliberate alterations in dialysate potassium concentration to affect hemodynamic stability would not be without risk. Use of low potassium dialysate concentration may contribute to arrhythmias, especially in those patients with underlying coronary artery disease or those taking digoxin. On the other hand, use of dialysate with high potassium concentration may predispose patients to predialysis hyperkalemia. In patients who are at high risk for arrhythmias on dialysis, modeling the dialysate potassium concentration to maintain a constant blood to dialysate potassium gradient throughout the procedure may be of clinical benefit (FIGURE 2.2).
Dialysate Buffer
Acetate Buffer
The early use of bicarbonate as the base in dialysis solutions required a cumbersome system in which CO2 was continuously bubbled through the dialysate to lower pH to prevent the precipitation of calcium and magnesium salts. As a result, in the early 1960s, acetate became the standard dialysate buffer used to correct uremic acidosis and to offset the diffusive losses of bicarbonate during hemodialysis. Over the next several years, reports began to accumulate linking routine use of acetate with cardiovascular instability and hypotension during dialysis. In particular, critically ill patients undergoing acute hemodialysis, especially with the use of large surface area dialyzers, were found to exhibit vascular instability when exposed to acetate in dialysis fluid. Given these observations, bicarbonate-containing dialysate began to reemerge as the principal dialysate buffer especially as advances in biotechnology made the use of bicarbonate dialysate less expensive and less cumbersome to use. Because acetate is still used as the principal dialysate buffer in some centers, the following paragraphs compare the clinical effects of acetate and bicarbonate dialysate.
During a routine dialysis in which acetate is used as the buffer, there are large fluxes of both acetate and bicarbonate across the dialyzer with little overall net gain in base. Because the acetate dialysate lacks bicarbonate, there is diffusion of actual bicarbonate from the blood into the dialysate. In addition, potential bicarbonate is lost from the blood in the form of organic anions such as citrate, lactate, pyruvate, BETA-hydroxy-butyrate, and acetoacetate because these anions diffuse across the dialyzer and into the dialysate (50). The pH of blood exiting the dialysis cartridge does not fall because CO2 diffuses from the blood as well. Despite this large efflux of potential and actual bicarbonate from the body, there is a net gain of alkali because of the greater influx of acetate as it diffuses from the dialysate into the blood. Acetate is normally metabolized in muscle to acetyl coenzyme A (CoA). Acetyl CoA is then metabolized in the Krebs cycle to CO2 and water with formation of one bicarbonate molecule for each molecule of acetate metabolized. The metabolism of acetate to carbon dioxide can contribute up to 40% of the total energy expenditure during dialysis (51). In addition, acetate-containing dialysate is associated with significant increases in the plasma concentration of ketone bodies. The accelerated ketogenesis and the extra caloric burden seen with acetate dialysate does not appear to be associated with an impairment in glucose utilization or change in plasma insulin during the procedure.
Chronic hemodialysis patients metabolize acetate at a reduced rate as compared with control patients (39). Decreased rates of metabolism of acetate to bicarbonate can potentially result in accumulation of acetate. This accumulation of acetate is magnified with use of dialyzers with large surface areas that allow for transfer of acetate to the plasma compartment at rates greater than a patient’s ability to metabolize it. The consequent increase in blood concentration of acetate has been associated with nausea, vomiting, fatigue, and, more importantly, hemodynamic instability.
There are a number of mechanisms by which acetate might predispose to vascular instability (TABLE 2.3). Acetate, possibly by its conversion to adenosine, has vasodilatory properties that can directly decrease peripheral vascular resistance. In addition, acetate might increase venous capacity leading to a decrease in cardiac filling (52). These vasodilatory effects of acetate are further augmented through the release of interleukin-1. Interleukin-1 has vasodilatory properties and its activity is increased from 8 to 12 times normal by standard acetate hemodialysis (53).
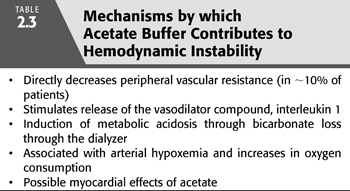
In addition to direct vasodilatory properties, incomplete acetate oxidation can lead to acid–base changes that can adversely affect hemodynamics. Metabolism of acetate to bicarbonate in an amount less than the diffusive losses of bicarbonate through the dialyzer will result in a decreased concentration of serum bicarbonate. A decrease in serum bicarbonate concentration can result in mild metabolic acidosis that may take 2 to 3 hours after dialysis to correct. Such loss of bicarbonate through the dialyzer contributes to vascular instability in addition to any direct vascular effects of acetate.
Arterial hypoxemia occurs with the use of acetate dialysate and may also contribute to vascular instability. During acetate dialysis, there is diffusive loss of soluble carbon dioxide from blood to dialysate. In an attempt to maintain normal blood carbon dioxide concentration, pulmonary hypoventilation occurs resulting in hypoxemia. In addition, metabolism of acetate leads to an increase in oxygen consumption further exacerbating any decrease in oxygenation. In susceptible individuals, acetate may provoke subendocardial ischemia by deleteriously affecting myocardial oxygen balance (54).
Despite the association of acetate with a higher frequency of symptoms in some studies, there is a poor correlation between symptoms and signs and blood acetate concentration. In an attempt to reconcile this discrepancy, Vinay et al. (55) studied a large population of dialysis patients and concluded that true acetate intolerance only occurred in about 10% of the dialysis population. The patients found intolerant were unable to metabolize acetate optimally and were predominately female. Because muscle is the primary site of metabolism of acetate, a reduced muscle mass often found in females might account for the reduced metabolism of acetate. Similarly, malnourished and elderly patients may be more predisposed to acetate intolerance by such a mechanism.
During conventional hemodialysis with acetate, the vasoconstrictive response to hypovolemia is masked by the vasodilatory tendency induced by the diffusive nature of the therapy. It is unclear, however, whether inability to vasoconstrict during conventional hemodialysis is related to use of acetate or rather a fall in serum osmolality. In this regard, several studies have found that vascular instability with acetate dialysis is generally improved with use of a higher Na dialysate concentration. In addition, substitution of bicarbonate for acetate as the base constituent in the dialysate has been purported to markedly improve symptoms on dialysis. It has been difficult to prove, however, whether bicarbonate dialysate can independently further improve hemodynamic and symptomatic tolerance to hemodialysis especially when a higher dialysate osmolality is used. Wehle et al. (56) found no added benefit of bicarbonate over acetate particularly if dialysate Na was increased to 145 mEq/L. Henrich et al. (57) in a comparative study of acetate and bicarbonate dialysis using a dialysate Na concentration of 140 mEq/L demonstrated strikingly similar hemodynamic responses. In a later study, Velez et al. (58) noted that with high Na dialysates (141 mEq/L), no detectable differences between bicarbonate and acetate were found with respect to cardiac output, mean blood pressure, or orthostatic tolerance to standing after dialysis. With use of a dialysate Na of 130 mEq/L, however, bicarbonate dialysate was associated with greater stability of blood pressure on standing after dialysis.
Controversy exists as to the overall effects of acetate and bicarbonate buffers on ventricular performance. Administration of sodium acetate as a bolus injection in dogs results in marked decreases in myocardial contractile force and blood pressure (59). However, if given as a continuous infusion, no definite depression of myocardial function is observed. Aizawa et al. (60) compared the effects of acetate and bicarbonate hemodialysis on cardiac function using phonocardiography. Left ventricular function was depressed to a greater extent with acetate than after bicarbonate dialysis.
The negative inotropic effect attributed to acetate has been contested by other reports in which sodium acetate was found to increase ventricular performance. Nitenberg et al. (61) using angiographic techniques studied left ventricular function in seven patients with plasma acetate concentrations comparable to that found during sodium acetate hemodialysis. An increase in cardiac index, ejection fraction, and maximum velocity of circumferential fiber (VCF) shortening not attributable to alterations in heart rate, preload, or afterload was demonstrated after sodium acetate infusion. Mansell et al. (62) noted that patients who developed hyperacetatemia during regular dialysis were still able to maintain an increased cardiac output. Schick et al. (63) studied nine patients in a double-blind, crossover manner and demonstrated improvement of left ventricular mean VCF of equal magnitude with both acetate and bicarbonate hemodialysis. Ruder et al. (64) performed a double-blind, crossover study of 36 patients comparing acetate and bicarbonate hemodialysis with respect to baseline left ventricular function. In patients with depressed VCF, hemodialysis with either buffer resulted in improvement of ventricular function with mean VCF significantly higher after bicarbonate dialysis as compared with acetate. In patients with normal VCF, only bicarbonate dialysis produced significantly better ventricular function. In contrast, Leunissen et al. (65,66) found increases in VCF in patients with normal left ventricular function with either acetate or bicarbonate dialysis. In patients with compromised left ventricular function, only bicarbonate dialysis resulted in significant increase in VCF.
The disparity of results in studies of effects of dialysate composition on cardiac function may in part results from failure to distinguish effects of volume removal. In this regard, Nixon et al. (67) demonstrated that dialysis with acetate with or without volume removal produced an increase in contractility. Mehta et al. (68) performed isovolemic dialysis and found that mean VCF improved to the same extent with both acetate and bicarbonate dialysis. Anderson et al. (69) studied left ventricular performance with two-dimensional echocardiography in five patients under three different cardiac filling volumes before and after a standard isovolemic hemodialysis. Cardiac performance as assessed by VCF improved comparably with acetate or bicarbonate under conditions of increased or decreased preloads.
In summary, many years of experience with acetate as the principal buffer in dialysate has not borne out concerns that acetate would be associated with long-term adverse consequences. In most chronic stable dialysis patients, cardiac function is improved with acetate or bicarbonate-containing dialysate. Under conditions in which a dialysate Na of more than 140 mEq/L is used, bicarbonate offers no apparent hemodynamic advantage over acetate. By contrast, use of a bicarbonate bath may provide more hemodynamic stability than acetate under conditions of a low Na dialysate concentration (less than 135 mEq/L) or in patients who are truly acetate intolerant. In addition, bicarbonate-containing dialysate improves platelet function to a greater extent than acetate and may be less arrhythmogenic in susceptible patients. In either case, it should be pointed out that as high-efficiency and especially high-flux dialysis becomes more widely used, acetate-containing dialysate will become a thing of the past. The increased clearance seen with these procedures would allow the rate of influx of acetate to exceed the maximal rate of metabolism such that acid–base balance could not be maintained.
Bicarbonate Buffer
Bicarbonate is now the principal buffer used in dialysate. Producing bicarbonate dialysate requires a specifically designed system that mixes a bicarbonate concentrate and an acid concentrate with purified water. The acid concentrate contains a small amount of either lactic or acetic acid and all the calcium and magnesium. The exclusion of these cations from the bicarbonate concentrate prevents the precipitation of magnesium and calcium carbonate that would otherwise occur in the setting of a high bicarbonate concentration. During the mixing procedure, the acid in the acid concentrate will react with an equimolar amount of bicarbonate to generate carbonic acid and carbon dioxide. The generation of carbon dioxide causes the pH of the final solution to fall to approximately 7.0 to 7.4. This more acidic pH as well as the lower concentrations of calcium and magnesium in the final mixture allow for these ions to remain in solution. The final concentration of bicarbonate in the dialysate is generally fixed in the range of 33 to 38 mmol/L (FIGURE 2.3).
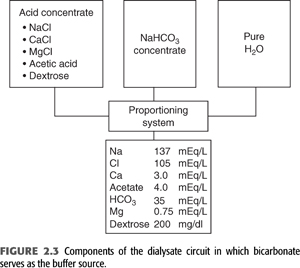
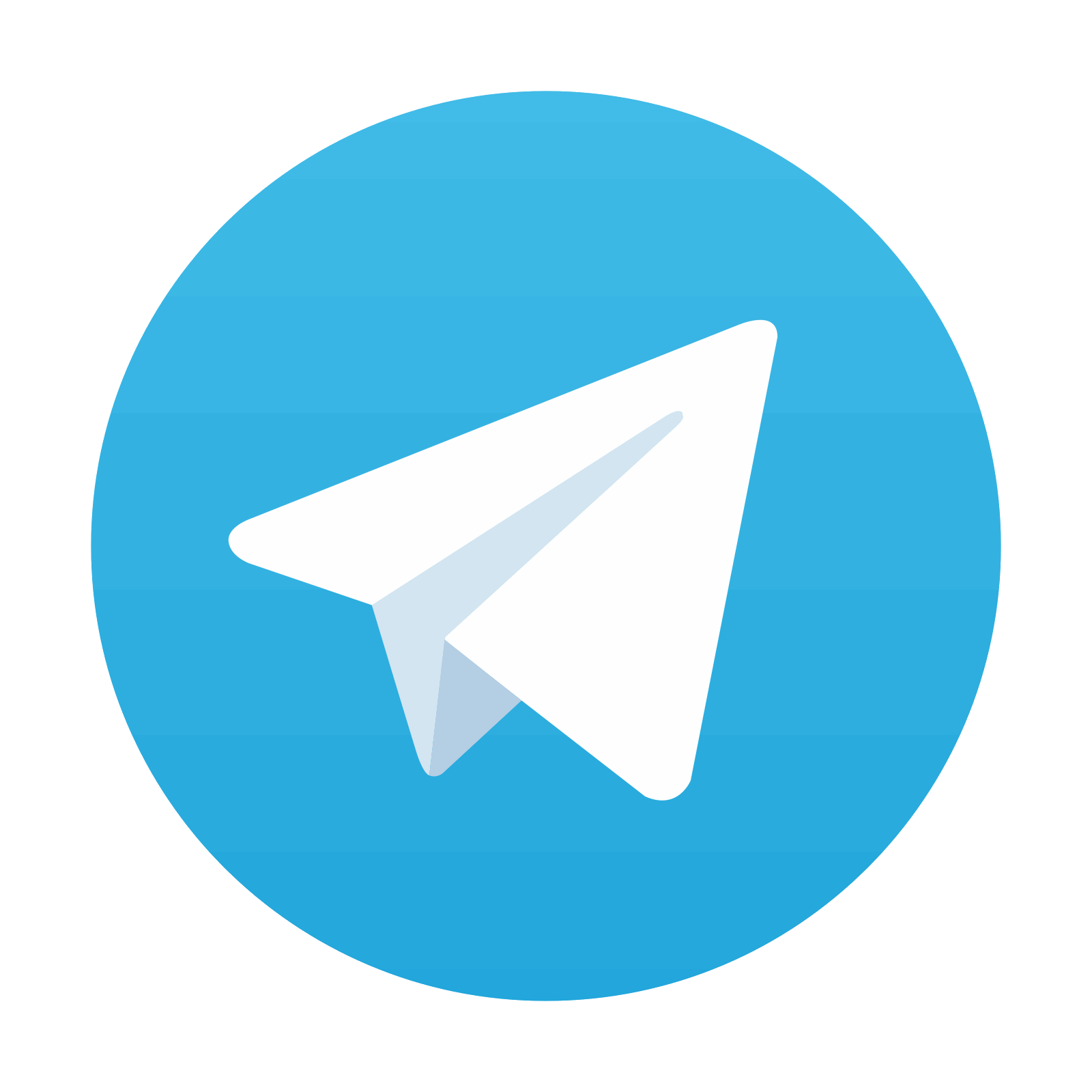
Stay updated, free articles. Join our Telegram channel

Full access? Get Clinical Tree
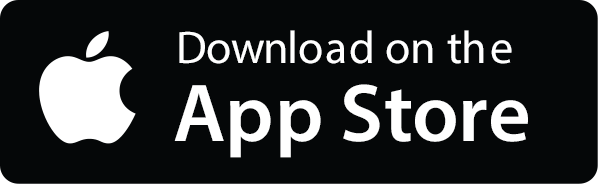
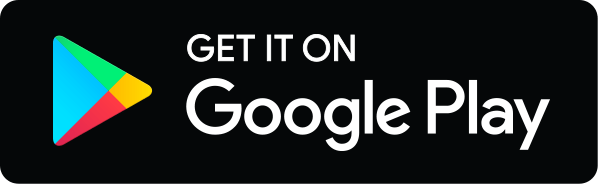