cells from bone marrow, peripheral blood, or umbilical cord blood. By 2007, 80% of allogeneic and nearly 100% of autologous transplants involved the use of peripheral blood cells.6,7 AKI has been found to be more common in recipients of marrow transplant as opposed to peripheral stem cells in some studies11 but not in others.13 Conversely, chronic GVHD (cGVHD) may be more prevalent in recipients of peripheral stem cell transplantation due to the greater dose of delivered T cells.13,15,16,17 No association has been established between the number of infused stem cells per body weight and AKI.18
TABLE 38.1 Common Indications for Hematopoietic Cell Transplantation | |||||||||||||||||||||||||||||
---|---|---|---|---|---|---|---|---|---|---|---|---|---|---|---|---|---|---|---|---|---|---|---|---|---|---|---|---|---|
|
immunosuppressive drugs, typically cyclosporine or tacrolimus plus methotrexate, although mycophenolate mofetil and sirolimus can also be used.30 Prophylactic drugs may be withdrawn earlier in RIC to facilitate graft-versus-tumor effect. Prophylaxis for infection includes acyclovir for patients seropositive for herpes simplex virus (HSV), trimethoprim/sulfamethoxazole to prevent Pneumocystis jiroveci infection, oral fluconazole for fungal prevention, and preemptive ganciclovir or foscarnet for cytomegalovirus (CMV) infection in viremic recipients.31,32 Amphotericin, voriconazole, or micafungin are used for patients at risk for aspergillus infection.33 Comparing outcomes in allogeneic recipients in the periods between 1993 and 1997 and 2003 and 2007, there has been a significant decrease in bacterial, fungal, and CMV infections.12 In addition to improvements in the use of prophylactic antimicrobials, the increased use of peripheral blood donor cells has resulted in significantly faster neutrophil engraftment and earlier reconstitution of immunity.
TABLE 38.2 Distinguishing Features of the Three Modalities of Hematopoietic Cell Transplant | ||||||||||||||||||||||||||||||||||||||||||||||||||||||||||||||||||||||||||||||||
---|---|---|---|---|---|---|---|---|---|---|---|---|---|---|---|---|---|---|---|---|---|---|---|---|---|---|---|---|---|---|---|---|---|---|---|---|---|---|---|---|---|---|---|---|---|---|---|---|---|---|---|---|---|---|---|---|---|---|---|---|---|---|---|---|---|---|---|---|---|---|---|---|---|---|---|---|---|---|---|---|
|
conditioning regimens, immunosuppressants, prophylactic medications, and length of follow-up. In addition, the lack of a standardized definition for AKI has been vexing to those seeking to understand the epidemiology of the disease. Two recently proposed sets of diagnostic criteria, RIFLE (risk, injury, failure, loss of kidney function, end-stage kidney disease)35 and AKIN (Acute Kidney Injury Network),36 have facilitated diagnostic standardization and allowed for significant advances in understanding the epidemiology and outcomes of AKI at large.37 The definitions employed by three common classification systems for AKI following HCT are shown in Table 38.3. Although many studies of post-HCT AKI have used a variation of the classification system utilized by Parikh et al.,38 the sensitivity of the RIFLE and AKIN criteria has recently been evaluated for diagnosis and prediction of long-term, all-cause mortality associated with post-HCT AKI.39 AKIN identified the smallest number of patients as having AKI across all three modalities due to a reduced sensitivity for identifying the lowest category of AKI. For severe AKI, denoted as RIFLE ≥ injury, AKIN ≥ stage 2, or Parikh ≥ grade 2, all three systems performed identically. As seen in multiple other settings,40,41 RIFLE and AKIN stages, along with those of the Parikh classification, were associated in a stepwise manner with mortality. The HCT-Comorbidity (HCT-CI) index is composed of cardiac, pulmonary, hepatic, gastrointestinal, and renal function tests that are sensitive for the detection of subclinical organ impairment and has been shown to predict nonrelapse mortality.42,43 Both intermediate, hazard ratio (HR) 2.42, and high risk, HR 4.69, HCT-CI scores were independently associated with the development of RIFLE “I” and “F” in a combined cohort of myeloablative and RIC allogeneic recipients.43
TABLE 38.3 Commonly Utilized Definitions of Acute Kidney Injury in Hematopoietic Cell Transplant Studies | ||||||||||||||||||||||||||||||||||||||||||||||||
---|---|---|---|---|---|---|---|---|---|---|---|---|---|---|---|---|---|---|---|---|---|---|---|---|---|---|---|---|---|---|---|---|---|---|---|---|---|---|---|---|---|---|---|---|---|---|---|---|
|
Befitting the discrepant patient populations, conditioning regimens, and prophylactic medications seen in these studies, myriad risk factors have been found for AKI. Conflicting results have been reported for multiple factors. Additionally, many studies have not utilized adjusted analysis, calling the true influence of postulated risk factors with potential colinearity into question. Those that have been found significant after adjustment in multiple studies include sinusoidal obstruction syndrome (SOS),28,48,50,51,52,53,63,67,68 cyclosporine use/toxicity,45,53,64 hyperbilirubinemia/jaundice,46,48 amphotericin,28,50 aGVHD,48,51 and chronic kidney disease (CKD).46,55 A full listing of risk factors for AKI in myeloablative allogeneic as well as autologous and RIC HCT are provided in Table 38.6.
TABLE 38.4 Comparison of Acute Kidney Injury in Single Modality Hematopoietic Cell Transplant Studies | ||||||||||||||||||||||||||||||||||||||||||||||||||||||||||||||||||||||||||||||||||||||||||||||||||||||||||||||||||||||||||||||||||||||||||||||||||||||||||||||||||||||||||||||||||||||||||||||||||||||||||||||||||||||||||||||||||||||||||||||||||||||||||||||||||||||||||||||||||||||||||||||||||||||||||||||||||||||||||||||||||||||||||
---|---|---|---|---|---|---|---|---|---|---|---|---|---|---|---|---|---|---|---|---|---|---|---|---|---|---|---|---|---|---|---|---|---|---|---|---|---|---|---|---|---|---|---|---|---|---|---|---|---|---|---|---|---|---|---|---|---|---|---|---|---|---|---|---|---|---|---|---|---|---|---|---|---|---|---|---|---|---|---|---|---|---|---|---|---|---|---|---|---|---|---|---|---|---|---|---|---|---|---|---|---|---|---|---|---|---|---|---|---|---|---|---|---|---|---|---|---|---|---|---|---|---|---|---|---|---|---|---|---|---|---|---|---|---|---|---|---|---|---|---|---|---|---|---|---|---|---|---|---|---|---|---|---|---|---|---|---|---|---|---|---|---|---|---|---|---|---|---|---|---|---|---|---|---|---|---|---|---|---|---|---|---|---|---|---|---|---|---|---|---|---|---|---|---|---|---|---|---|---|---|---|---|---|---|---|---|---|---|---|---|---|---|---|---|---|---|---|---|---|---|---|---|---|---|---|---|---|---|---|---|---|---|---|---|---|---|---|---|---|---|---|---|---|---|---|---|---|---|---|---|---|---|---|---|---|---|---|---|---|---|---|---|---|---|---|---|---|---|---|---|---|---|---|---|---|---|---|---|---|---|---|---|---|---|---|---|---|---|---|---|---|---|---|---|---|---|---|---|---|---|---|---|---|---|---|---|---|---|---|---|---|---|---|---|---|---|---|---|---|---|---|---|---|---|---|---|---|---|---|---|
|
TABLE 38.5 Studies Comparing Multiple Hematopoietic Cell Transplant Modalities | ||||||||||||||||||||||||||||||||||||||||||||||||||||||||||||||||||||||||||||||||||||||||||||||||||||||||||||||||||||||||||||||||||||||||||||||||||||||||||||||||||||||||||||||||||||||||||||
---|---|---|---|---|---|---|---|---|---|---|---|---|---|---|---|---|---|---|---|---|---|---|---|---|---|---|---|---|---|---|---|---|---|---|---|---|---|---|---|---|---|---|---|---|---|---|---|---|---|---|---|---|---|---|---|---|---|---|---|---|---|---|---|---|---|---|---|---|---|---|---|---|---|---|---|---|---|---|---|---|---|---|---|---|---|---|---|---|---|---|---|---|---|---|---|---|---|---|---|---|---|---|---|---|---|---|---|---|---|---|---|---|---|---|---|---|---|---|---|---|---|---|---|---|---|---|---|---|---|---|---|---|---|---|---|---|---|---|---|---|---|---|---|---|---|---|---|---|---|---|---|---|---|---|---|---|---|---|---|---|---|---|---|---|---|---|---|---|---|---|---|---|---|---|---|---|---|---|---|---|---|---|---|---|---|---|---|---|
|
absolute change required for doubling of serum creatinine is less in patients with lower baselines, with the same following for GFR, and thus those patients with preserved baseline function require smaller changes to meet AKI criteria.
TABLE 38.6 Risk Factors Independently Associated with Acute Kidney Injury Following Hematopoietic Cell Transplant | ||||||||||||||||||||||||||||||||||||||||||||||||||||||||||||||||||||||||||||||||||||||||||||||||||||
---|---|---|---|---|---|---|---|---|---|---|---|---|---|---|---|---|---|---|---|---|---|---|---|---|---|---|---|---|---|---|---|---|---|---|---|---|---|---|---|---|---|---|---|---|---|---|---|---|---|---|---|---|---|---|---|---|---|---|---|---|---|---|---|---|---|---|---|---|---|---|---|---|---|---|---|---|---|---|---|---|---|---|---|---|---|---|---|---|---|---|---|---|---|---|---|---|---|---|---|---|
|
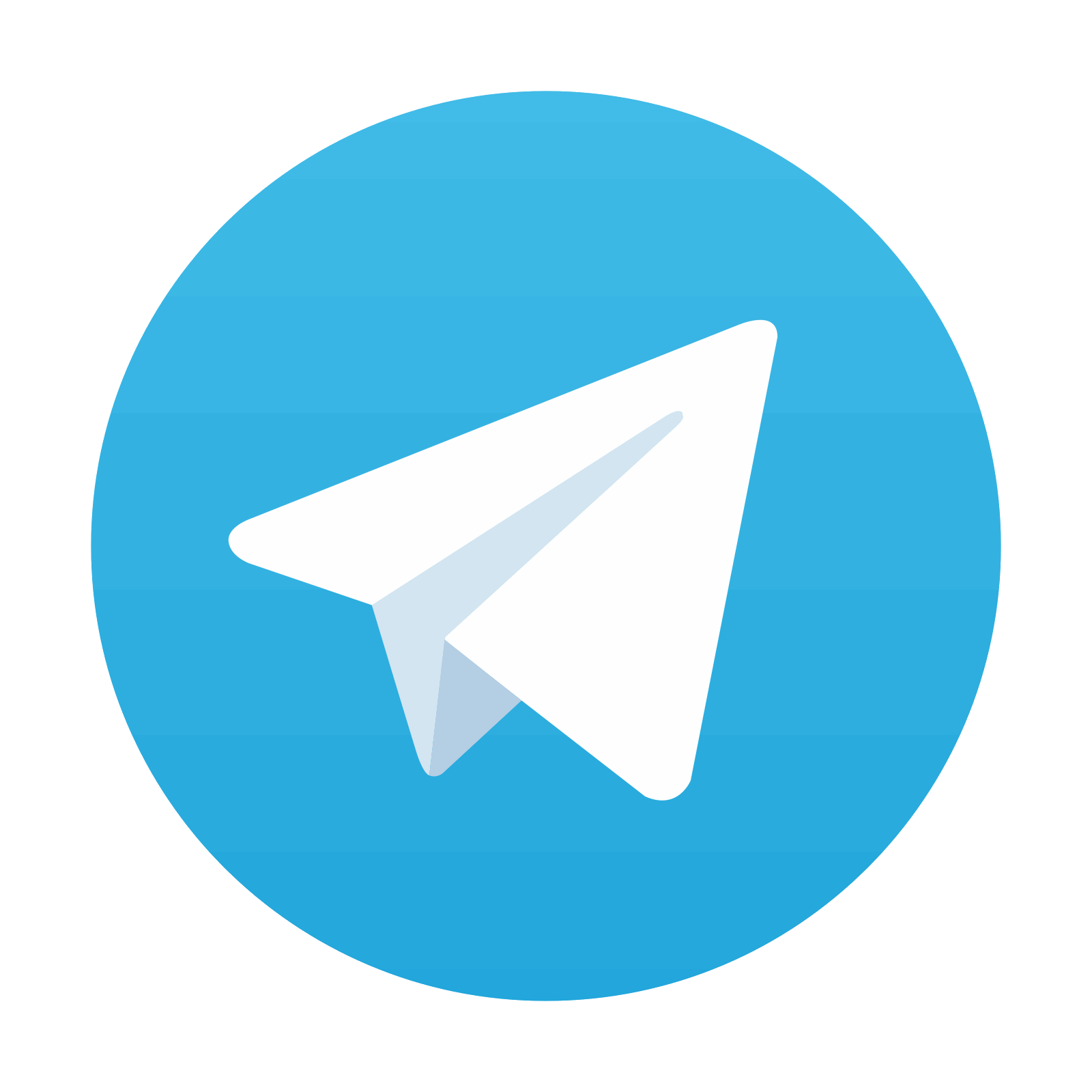
Stay updated, free articles. Join our Telegram channel

Full access? Get Clinical Tree
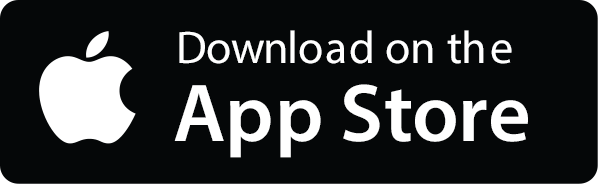
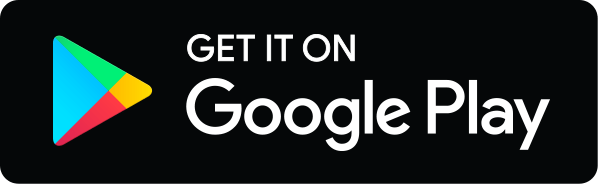