Abstract
In a term human gestation, the amount of water in the fetal compartments, including the fetus, placenta, and amniotic fluid (AF), may exceed 5 L; in pathologic states, the amount may be much more because of excessive AF or fetal hydrops. Water largely flows from the maternal circulation to the fetus via the placenta, and the rate of fetal water acquisition depends on placental water permeability characteristics. Within the gestational compartment, water is circulated between the fetus and the AF. In the latter part of pregnancy, an important facet of this circulation is water flux from the AF to the fetal circulation across the amnion. Normal AF water dynamics are critical because insufficient (oligohydramnios) or excessive (polyhydramnios) amounts of AF are associated with impaired fetal outcome, even in the absence of structural fetal abnormalities. This chapter reviews data regarding the placental transfer of water and examines the circulation of water within the gestation, specifically the water flux across the amnion, as factors influencing AF volume. Finally, some controversies regarding the mechanics of these events are discussed.
Keywords
amniotic, placenta, fluid, aquaporins
- •
Clinical Scenarios
- •
Fetal Water
- •
Mechanisms of Water Flow
- •
Aquaporins
- •
Conclusion
In a term human gestation, the amount of water in the fetal compartments, including the fetus, placenta, and amniotic fluid (AF), may exceed 5 L; in pathologic states, the amount may be much more because of excessive AF or fetal hydrops. Water largely flows from the maternal circulation to the fetus via the placenta, and the rate of fetal water acquisition depends on placental water permeability characteristics. Within the gestational compartment, water is circulated between the fetus and the AF. In the latter part of pregnancy, an important facet of this circulation is water flux from the AF to the fetal circulation across the amnion. Normal AF water dynamics are critical because insufficient (oligohydramnios) or excessive (polyhydramnios) amounts of AF are associated with impaired fetal outcome, even in the absence of structural fetal abnormalities. This chapter reviews data regarding the placental transfer of water and examines the circulation of water within the gestation, specifically the water flux across the amnion, as factors influencing AF volume. Finally, some controversies regarding the mechanics of these events are discussed.
Clinical Scenarios
Water flux in the placenta and chorioamnion is a matter of more than theoretical interest. Clinical experience in humans suggests that altered placental water flow may occur and can cause deleterious fetal effects in association with excessive or reduced AF volume. Similarly, primary abnormalities in fetal fluid exchange with the amniotic cavity may result in markedly abnormal AF volume.
Maternal Dehydration
Maternal dehydration has been associated with reduced fetal compartment water and oligohydramnios. As an example, the following case has been reported: a 14-year-old girl was admitted at 33 weeks’ gestation with cramping and vaginal spotting. A sonogram indicated oligohydramnios and an AF index (AFI) of 2.6 cm (reference range, 5–25 cm) with normal fetal kidneys and bladder. On hospital day 2, the AFI was 0 cm. Recorded maternal fluid balance was 8 L in and 13.6 L out. Serum sodium was 153 mEq/L. Diabetes insipidus was diagnosed and treated with intranasal desmopressin acetate. With the normalization of maternal fluid and serum osmolality status, the oligohydramnios resolved rapidly. The patient ultimately delivered a healthy 2700 g male infant at 38 weeks’ gestation.
Reduced Maternal Plasma Oncotic Pressure
Maternal malnutrition may predispose patients to increased fetal water transfer and polyhydramnios, a result of reduced maternal plasma oncotic pressure. We recently encountered a patient who illustrated this condition: a 35-year-old gravida 4, para 3 presented at 32 to 33 weeks of gestation complaining of premature labor. On admission, the maternal hematocrit was 18.9% and hemoglobin was 5.6 g/dL with a mean corpuscular volume of 57.9 fL. Blood chemistries were normal except that the patient’s serum albumin was 1.9 g/dL (reference range, 3.3–4.9 g/dL). A diagnosis of severe maternal malnutrition was made. On ultrasound examination, the AFI was 24.5 cm, and the fetal bladder was noted to be significantly enlarged, which is consistent with increased urine output. Premature labor was thought to be attributable to uterine overdistension. Subsequently, the patient delivered a 1784-g male infant with Apgar scores of 3 at 1 minute and 7 at 5 minutes. The infant was transferred to the neonatal intensive care unit for significant respiratory distress.
As described later, although the forces driving normal maternal-to-fetal water flux are uncertain, changes in the osmotic–oncotic difference between the maternal and fetal sera can affect the volume of water flowing from the mother to the fetus. In the first case of maternal dehydration, presumably because of an environment of increased maternal osmolality, less water crossed the placenta to the fetus. Similarly, maternal dehydration caused by water restriction (in a sheep model) or caused by hot weather (in humans) has been associated with reduced AF volumes. Conversely, reduced maternal oncotic pressure likely contributed to increased maternal-to-fetal water transfer in the second patient. Fetal renal homeostatic mechanisms then led to increased fetal urine output and increased AF volume. Similarly, studies administering DDAVP (desmopressin) to both humans and sheep have demonstrated that a pharmacologic reduction in maternal serum osmolality can lead to an increase in AF. As these examples illustrate, maternal-fetal water flow is a carefully balanced system that can be perturbed with clinically significant effect. The material presented below will detail the mechanisms regulating fetal–maternal–AF fluid homeostasis.
Fetal Water
Placental Water Flux
Net water flux across the placenta is relatively small. In sheep, a bulk water flow to the fetus of 0.5 mL/min is sufficient for fetal needs at term. By contrast, tracer studies suggest that the total water exchanged (i.e., diffusionary flow) between the ovine fetus and the mother is dramatically larger, up to 70 mL/min. Most of this diffusionary flow is bidirectional, resulting in no net accumulation of water. Although the mechanisms regulating the maternal–fetal flux of water are speculative, the permeability of the placenta to water changes with gestation, suggesting that placental water permeability may be a factor in regulating the water available to the fetus.
Although fetal water may derive from sources other than transplacental flux, these other sources appear to be of minor importance. Water could, theoretically, pass from the maternal circulation to the AF across the fetal membranes (i.e., transmembrane flow), although this flow rate is thought to be small, partly because the AF is hypotonic compared with maternal serum. The driving force (AF to maternal direction) resulting from osmotic and oncotic gradients between hypotonic, low-protein AF and isotonic maternal serum is far greater than that induced by maternal vascular versus AF hydrostatic pressure (maternal to AF direction). Any direct water flux between maternal serum and AF should therefore be from the fetus to the mother. In addition, a small amount of water is produced as a byproduct of fetal metabolic processes. Because these alternative routes contribute only a minor proportion of the fetal water, it is apparent that the fetus is dependent on placental flux for the bulk of water requirements.
Fetal Water Compartments
In gestation, water is partitioned between the fetus, placenta and membranes, and AF. Although term human fetuses may vary considerably in size, an average fetus contains 3000 mL of water, of which about 350 mL is in the vascular compartment. In addition, the placenta contains another 500 mL of water. As the amount of water in infants averages 75% of body weight, the volume of fetal and placental water is proportionate to the fetal weight. AF volume is less correlated with fetal weight, though reduced AF volume is associated with growth-restricted fetuses and increased AF volume is associated with macrosomic fetuses. The AF is a fetal water depot, and in normal human gestations at term, the AF volume may vary from 500 mL to more than 1200 mL. In pathologic states, the AF volume may vary more widely. Next, we present what is known regarding the formation of AF, the circulation of AF water, and the mechanisms controlling this circulation.
Amniotic Fluid Volume and Composition
During the first trimester, AF is isotonic with maternal plasma but contains minimal protein. It is thought that the fluid arises either from a transudate of fetal plasma through nonkeratinized fetal skin or maternal plasma across the uterine decidua or placental surface. With advancing gestation, AF osmolality and sodium concentration decrease, a result of the mixture of a larger volume of dilute fetal urine with a smaller volume of isotonic fetal lung liquid production. In comparison with the first half of pregnancy, AF osmolality decreases by 20 to 30 mOsm/kg H 2 O with advancing gestation to levels approximately 85% to 90% of maternal serum osmolality in humans, although there is no osmolality decrease in the AF near term in rats. AF urea, creatinine, and uric acid increase during the second half of pregnancy, a consequence of increased renal excretion, resulting in AF concentrations of these urinary byproducts two to three times higher than those of fetal plasma.
Concordant with the changes in AF content, AF volume changes dramatically during human pregnancy ( Fig. 1.1 ). The average AF volume increases progressively from 20 mL at 10 weeks to 630 mL at 22 weeks, and to 770 mL at 28 weeks’ gestation. Between 29 and 37 weeks’ gestation, there is little change in volume. Beyond 39 weeks, AF volume decreases sharply, averaging 515 mL at 41 weeks. When the pregnancy becomes postdate, there is a 33% decline in AF volume per week, consistent with the increased incidence of oligohydramnios in postterm gestations.

Fetal Water Circulation
AF is produced and resorbed in a dynamic process with large volumes of water circulated between the AF and fetal compartments ( Fig. 1.2 ). During the latter half of gestation, the primary sources of AF include fetal urine excretion and fluid secreted by the fetal lung. The primary pathways for water exit from the AF include removal by fetal swallowing and the intramembranous (IM) absorption of water into fetal vasculature. Although some data on these processes in the human fetus are available, the bulk of the information about fetal AF circulation derives from animal models, especially sheep.

Urine Production
In humans, fetal urine production changes with increasing gestation. The amount of urine produced by the human fetus has been estimated by the use of ultrasound assessment of fetal bladder volume, although the accuracy of these measurements has been called into question. Exact human fetal urine production rates across gestation are not established but appear to be in the range of 25% of body weight per day or nearly 1000 mL/day near term.
In near-term ovine fetuses, 500 to 1200 mL/day of urine is distributed to the AF and allantoic cavities. During the last third of gestation, the fetal glomerular filtration rate (GFR) increases in parallel to fetal weight, with a similar but variable increase in the reabsorption of sodium, chloride, and free water. Fetal urine output can be modulated, as numerous endocrine factors, including arginine vasopressin, atrial natriuretic factor, aldosterone, and prostaglandins, have been demonstrated to alter fetal renal blood flow, GFR, or urine flow rates. Importantly, physiologic increases in fetal plasma arginine vasopressin significantly increase fetal urine osmolality and reduce urine flow rates.
Lung Fluid Production
It appears that all mammalian fetuses normally secrete fluid from their lungs, contributing to the expansion and development of the lung. The absolute rate of fluid production by human fetal lungs has not been estimated; the fluid production rate has been extensively studied in the ovine fetus only. During the last third of gestation, the fetal lamb secretes an average of 100 mL/day per kilogram fetal weight. Under physiologic conditions, approximately half of the fluid exiting the lungs enters the AF and half is swallowed. Therefore, an average of approximately 165 mL/day of lung liquid enters the AF near term. Fetal lung fluid production is affected by physiologic and endocrine factors, but nearly all stimuli tested have been demonstrated to reduce fetal lung liquid production, with no evidence of stimulated production and nominal changes in fluid composition. Increased arginine vasopressin, catecholamines, and cortisol, even acute intravascular volume expansion, decrease lung fluid production. Given this lack of evidence of bidirectional regulation, it appears that, unlike the kidneys, the fetal lungs may not play an important role in the maintenance of AF volume homeostasis though they do provide fluid to the amniotic cavity. Current opinion is that fetal lung fluid secretion is likely most important in providing pulmonary expansion, which promotes airway and alveolar development.
Fetal Swallowing
Studies of near-term pregnancies suggest that the human fetus swallows an average of 210 to 760 mL/day of AF, which is considerably less than the volume of urine produced each day. However, fetal swallowing may be reduced beginning a few days before delivery, so the rate of human fetal swallowing is probably underestimated. Little other data on human fetal swallowed volumes are available, although investigators have quantified human fetal gastric emptying as a surrogate measure of volume swallowed. In fetal sheep, there is a steady increase in the volume of fluid swallowed over the last third of gestation. In contrast to a relatively constant daily urine production/kg body weight, the daily volume swallowed increases from approximately 130 mL/kg per day at 0.75 term to more than 400 mL/kg per day near term. A series of studies have measured ovine fetal swallowing activity with esophageal electromyograms and swallowed volume using a flow probe placed around the fetal esophagus. These studies demonstrate that fetal swallowing increases in response to dipsogenic (e.g., central or systemic hypertonicity or central angiotensin II ) or orexigenic (central neuropeptide Y ) stimulation and decreases with acute arterial hypotension or hypoxia. Thus, near-term fetal swallowed volume is subject to periodic alterations as mechanisms for “thirst” and “appetite” develop functionality, although decreases in swallowed volume appear to be more reflective of deteriorating fetal condition.
Intramembranous Flow
The amount of fluid swallowed by the fetus does not equal the amount of fluid produced by both the kidneys and the lungs in either human or ovine gestation. As the volume of AF does not greatly increase during the last half of pregnancy, another route of fluid absorption is needed. This route is the IM pathway.
The IM pathway refers to the route of absorption between the fetal circulation and the amniotic cavity directly across the amnion. Although the contribution of the IM pathway to the overall regulation and maintenance of AF volume and composition has yet to be completely understood, results from in vivo and in vitro studies of ovine membrane permeability suggest that the permeability of the fetal chorioamnion is important for determining AF composition and volume. This IM flow, recirculating AF water to the fetal vascular compartment, is thought to be driven by the significant osmotic gradient between the hypotonic AF and isotonic fetal plasma. In addition, electrolytes (e.g., Na + ) may diffuse down a concentration gradient from fetal plasma into the AF, and intraamniotic peptides (e.g., arginine vasopressin ) and other electrolytes (e.g., Cl − ) may be recirculated to the fetal plasma.
Although it has never been directly measured in humans, indirect evidence supports the presence of IM flow. Studies of intraamniotic 51 Cr injection demonstrated the appearance of the tracer in the circulation of fetuses with impaired swallowing. Additionally, alterations in IM flow may contribute to AF clinical abnormalities because membrane ultrastructure changes are noted with polyhydramnios or oligohydramnios.
Experimental estimates of the net IM flow averages 200 to 250 mL/day in fetal sheep and likely balances the flow of urine and lung liquid with fetal swallowing under homeostatic conditions. Filtration coefficients have been calculated, although IM flow rates under control conditions have not been directly measured. Mathematical models of human AF dynamics also suggest significant IM water and electrolyte fluxes, but trans membranous flow (AF to maternal) is extremely small compared with IM flow.
This detailed understanding of fetal fluid production and resorption provides little explanation as to how AF volume homeostasis is maintained throughout gestation and does not account for gestational alterations in AF volume or postterm or acute-onset oligohydramnios. As an example, the acute reduction in fetal swallowing in response to hypotension or hypoxia seen in the ovine model would not produce the reduced AF volume noted in stressed human fetuses. For this reason, recent research has addressed the regulation of water flow in the placenta and fetal membranes. We will discuss the possible mechanisms for the regulation of fetal water flow, beginning with a review of the general principles of membrane water flow.
Mechanisms of Water Flow
Biologic membranes exist, in part, to regulate water flux. Flow may occur through cells (i.e., transcellular) or between cells (i.e., paracellular), and the type of flow affects the composition of the fluid crossing the membrane. In addition, transcellular flow may occur across the lipid bilayer or through membrane channels or pores (i.e., aquaporins [AQPs]); the latter route is more efficient because the water permeability of the lipid membrane is low. Because the AQPs allow the passage of water only (and sometimes other small nonpolar molecules), transcellular flow is predominantly free water. Paracellular flow occurs through relatively wide spaces between cells and consists of both water and solutes in the proportions present in the extracellular space; large molecules may be excluded. Although water molecules can randomly cross the membrane by diffusion without net water flow, net flow occurs only in response to concentration (osmotic) or pressure (hydrostatic) differences.
Osmotic and hydrostatic forces are created when there is a difference in osmotic or hydrostatic pressure on either side of the membrane. Osmotic differences arise when there is a difference in solute concentration across the membrane. For this difference to be maintained, the membrane permeability of the solute must be low (i.e., a high reflection coefficient). Commonly, osmotic differences are maintained by charged ions such as sodium, or large molecules such as proteins (also called oncotic pressure). These solutes do not cross the cell membrane readily. Osmotic differences can be created locally by the active transport of sodium across the membrane with water following because of the osmotic force created by the sodium imbalance. It should be noted that although the transport of sodium is active, water flux is always a passive, nonenergy-dependent process. Hydrostatic differences occur when the pressure of fluid is greater on one side of the membrane. The most obvious example is the difference between the inside of a blood vessel and the interstitial space. Hydrostatic differences may also be created locally by controlling the relative direction of two flows. Even with equal initial pressures, a hydrostatic difference will exist if venous outflow is matched with arterial inflow (countercurrent flow). The actual movement of water in response to these gradients may be more complex as a result of additional physical properties, including unstirred layer effects and solvent drag.
Net membrane water flux is a function of the membrane properties and the osmotic and hydrostatic forces. Formally, this is expressed as the Starling equation:
Jv = LpS ( Δ P − σ RT ( c 1 − c 2 ) )
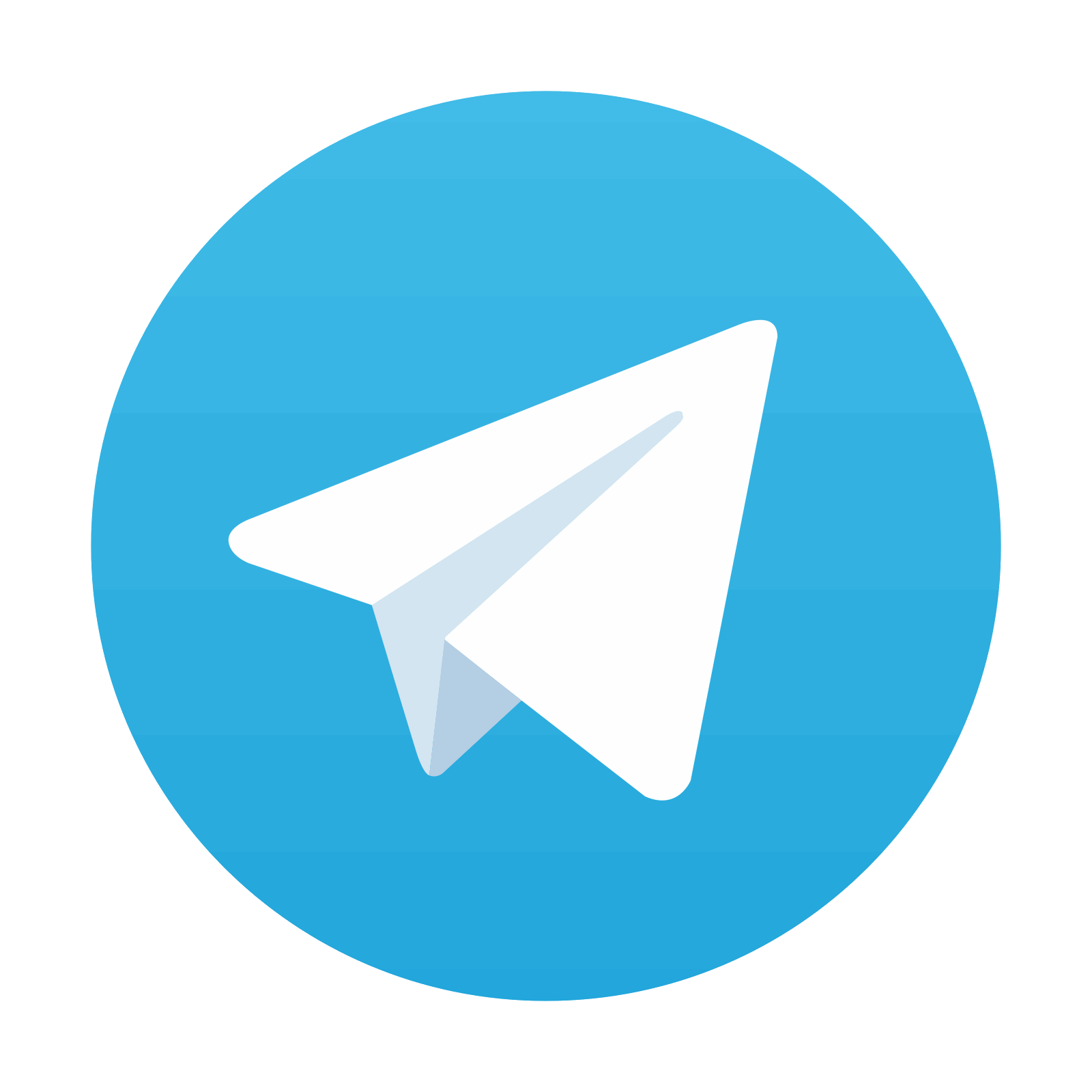
Stay updated, free articles. Join our Telegram channel

Full access? Get Clinical Tree
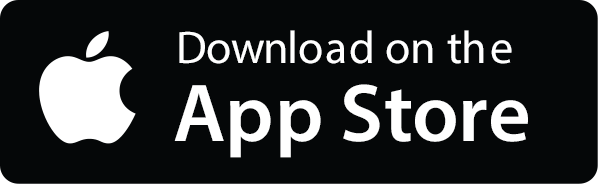
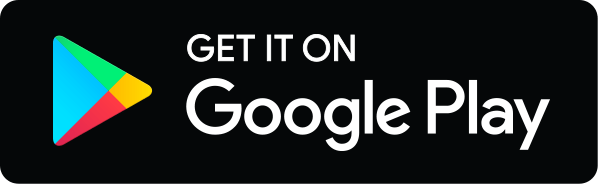