Victor W. Nitti, MD
Urodynamics (UDS) is the term used to describe testing and measurements of the function of the lower urinary tract. The lower urinary tract has two essential functions: the storage of urine at low pressure and the voluntary evacuation of urine. Low-pressure storage is essential to protect kidneys and ensure continence, whereas voluntary evacuation allows for the elimination of urine in socially acceptable situations without fear of leakage or overdistention. It is clear that a number of conditions and diseases affect the lower urinary tract and disrupt the storage and/or evacuation of urine. This can lead to bothersome symptoms (e.g., urinary incontinence or pain from failure to empty) or, in some cases, potentially harmful sequela. In many cases, a precise assessment of storage and emptying is necessary to optimally treat patients. UDS is the dynamic study of the transport, storage, and evacuation of urine. It is composed of a number of tests that individually or collectively can be used to gain information about urine storage and evacuation. UDS involves the assessment of the function and dysfunction of the urinary tract and includes the actual tests that are performed (UDS studies) and the observations during the testing (UDS observations) (Abrams et al, 1988, 2002). In this chapter we discuss the use of urodynamics to help in the assessment and treatment of voiding dysfunction. We keep that discussion in practical terms and supplement it with evidence for the technical performance and clinical utility of UDS.
Role of Urodynamic Testing in Clinical Practice
Rather than refer to a list of “indications for UDS” that often are not evidence based at all, it is more useful for clinicians to think of how UDS should be used in a broader clinical perspective. In keeping with that theme, the role of UDS in clinical practice has been nicely summarized by Hosker and colleagues (2009) for the following situations:
Functional Classification of Voiding Dysfunction: Applicability to Urodynamic Testing
In order to formulate a set of questions to be answered by a urodynamic test, an understanding of the possible causes of symptoms and the possible urodynamic manifestations of a preexisting condition is necessary. In order to accomplish this, a practical classification of voiding dysfunction is invaluable. The system proposed and popularized by Wein (1981) is simple and allows one to classify voiding dysfunction according to urodynamic findings. Functional abnormalities of the lower urinary tract can be divided into the following:
The beauty of a functional classification system is that it helps to clarify treatment options for a given patient. Thus in practical terms, the UDS evaluation should help to determine if there is bladder or bladder outlet dysfunction (or both) and whether there is a storage and/or emptying problem. By providing answers to these simple questions, the urodynamic test can lead to a correct diagnosis and, equally as important, institution of appropriate treatment. Obviously, an understanding of the physiology of urine storage and voiding and the pathophysiology of voiding dysfunction (see Chapter 61) is required to formulate appropriate questions to be answered by a urodynamic study. However, all too often clinicians get caught up in the intricate neurophysiologic aspects of voiding and storage dysfunction and fail to think in practical terms. One should always focus on the possible urodynamic findings in a given case and how each of the findings may ultimately affect the patient and his or her treatment. Obviously, these potential findings will be determined by symptoms and/or underlying conditions or diseases.
Conducting A Urodynamic Study: Patient and Technical Factors
Preparing for Urodynamic Study: Clinician, Patient, and Facility
By following these simple rules, one can maximize the chance of obtaining useful information from a study. If a particular question is not answered, the study can be repeated in the same session. Most people who do urodynamics regularly would concur that a urodynamic test is not always perfect in answering all important questions, but by defining the information needed before starting the study, “unanswered questions” can be kept to a minimum. As mentioned earlier, one of the most important parts of UDS is its proper performance with careful attention to technical details so that accurate interpretation is possible. It is beyond the scope of this chapter to describe the proper performance of UDS in detail; however, the reader is referred to Schafer and colleagues (2002) for good urodynamic practices and Abrams and colleagues (2002) for terminology. The International Continence Society (ICS) has now defined the term “urodynamic observations” to denote those observations that occur during and are measured by the UDS test itself. In order to be consistent, it is recommended that all clinicians performing and interpreting UDS use the current ICS terminology (Abrams et al, 2002). A list of common UDS terms is provided in Table 62–1.
Table 62–1 Terminology for Common Urodynamic Terms and Observations According to the International Continence Society Standardization Subcommittee
From Abrams P, Cardozo L, Fall M, et al. The standardisation of terminology of lower urinary tract function: report from the Standardisation Subcommittee of the International Continence Society. Neurourol Urodyn 2002;21:167–78; and Schafer W, Abrams P, Liao L, et al. Good urodynamic practices: uroflowmetry, filling, cystometry, and pressure-flow studies. Neurourol Urodyn 2002;21:261–74.
The importance of a well-trained, attentive, and supportive staff involved with the UDS study cannot be overemphasized. With that said, in general, UDS is well tolerated. However, patients should be properly prepared and told why the test is being done, how the results may affect treatment, and what to expect during the actual UDS test. Scarpero and colleagues (2005) used a questionnaire-based study to assess patient expectations of anxiety, pain, embarrassment, and apprehension before UDS and compared it with the patient’s actual experience. They found that UDS was associated with minimal to moderate degrees of anxiety, discomfort, and embarrassment. After testing, most respondents (>90% per question) thought that the test was the same or better than expected and had an expected or less than expected level of pain and embarrassment. This did not vary between the sexes, but a higher number of younger individuals found that the test experience was worse than expected, whereas a higher number of older individuals found that it was better than expected. Therefore younger patients may require more reassurance and attention in preparation for the procedure.
Components of the Urodynamic Study
Uroflowmetry is measurement of the rate of urine flow over time. It is also an assessment of bladder emptying. Normal uroflow is a bell-shaped curve. When the flow rate is reduced or the pattern is altered, this could indicate bladder (underactive) or bladder outlet (obstruction) dysfunction (Fig. 62–1).
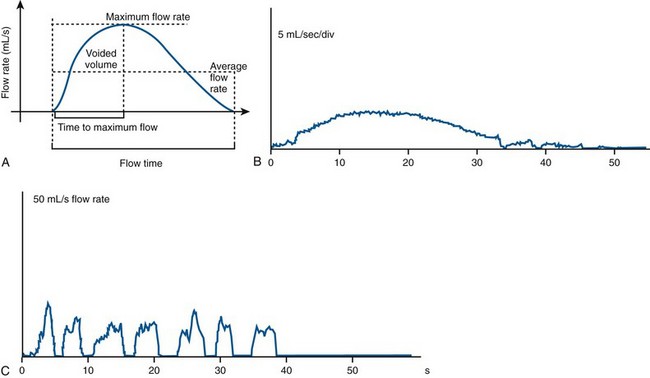
(A from Wein AJ, English WS, Whitemore KE. Office urodynamics. Urol Clin North Am 1988;15:609; B and C from Boone TB, Kim YH. Uroflowmetry. In: Nitti VW, editor. Practical urodynamics. Philadelphia: WB Saunders; 1998. p. 28–51.)
Cystometrogram (CMG): Cystometry or, more appropriately, “filling cystometry” is the method by which the pressure/volume relationship of the bladder is measured during bladder filling. The filling phase starts when filling commences and ends when the patient and urodynamicist decide that “permission to void” has been given. CMG can be performed by the single measurement of bladder pressure via a bladder catheter (urethral or suprapubic); however, changes in bladder pressure can represent a change in detrusor pressure (Pdet) or a change in abdominal pressure (Pabd) (see later). Therefore it is recommended that CMG be performed by measuring both the total vesical pressure (Pves) and Pabd (measured by a catheter placed in the rectum or vagina). To calculate Pdet, the following equation is used: Pdet = Pves − Pabd (Fig. 62–2).
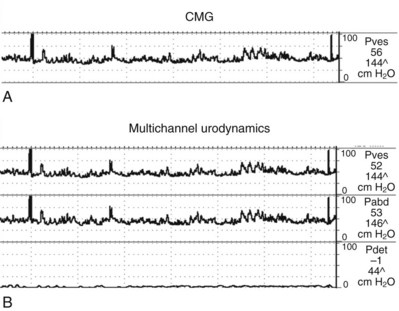
(From Nitti VW. Cystometry and abdominal pressure monitoring. In Nitti VW, editor. Practical urodynamics. Philadelphia: WB Saunders; 1998. p. 38–51.)
Urodynamic Equipment
Signal Transmission and Transducers
More recently, air-charged catheters have become popular for pressure measurement (T-Doc, Wenonah, NJ). This catheter uses a miniature, air-filled balloon placed circumferentially around a polyethylene catheter. External forces on the balloon of the catheter are transmitted to the air-filled catheter lumen and communicated to an external semiconductor transducer. The technology of the balloon system allows circumferential measurement readings. The catheters are disposable and single use. Air-charged catheters have several practical advantages over fluid-filled pressure lines because there is no fluid connection between the patient and the urodynamic equipment—just air. This means there is no hydrostatic pressure effect to account for so that there is no need to position anything at the level of the symphysis pubis and there is no need to flush the system through to exclude air (essential when using a fluid-filled system). Also, there are no artifactual fluctuations in pressure produced when the patients move. It must also be remembered that many UDS nomograms and other “standards of measurement” were determined using fluid-filled systems. Although there is comparative evidence for the use of air-charged catheters to measure urethral pressure (Pollak et al, 2004), no studies have shown definitively that air-charged catheters provide an acceptable alternative to fluid-filled lines for measuring intravesical and intra-abdominal pressure in UDS. Thus it is recommended that investigators planning to use air-charged catheters for intravesical and intra-abdominal pressure monitoring check for themselves that they have an equivalent performance to their current system for measuring pressure (Hosker et al, 2009).
Uroflowmeters
Urine flow rate or uroflow can be determined by a number of different types of devices or uroflowmeters. Modern uroflowmeters use weight, electrical capacitance, or a rotating disc to determine urinary flow rates. The two most common techniques used today are the weight transducer or load cell method and the rotating disc method. With the load cell the voided “weight” is measured and is then differentiated with respect to time to determine the flow rate. In the rotating disc method the urine stream is directed onto a rotating disc, and the power necessary to keep a disc rotating at a constant rate is measured. This power is proportional to the flow rate. The electronic dipstick flowmeter measures the electrical capacitance of a dipstick mounted in a collecting chamber. The output of the signal is proportional to the accumulated volume, and the volumetric flow rate is determined by differentiation. Each of these methods has advantages and disadvantages. The weight transducer method is simple, reliable, and accurate regardless of the site of stream impact but requires that the density of urine must be set. The rotating disc method is also reliable and accurate, and it provides a direct measurement without need for differentiation of volume with respect to time. Electronic flowmeters provide a range of electronically read flow parameters with graphical depiction of the uroflow and have sufficient precision for clinical use with error rates of 1% to 8% in voided volume and 4% to 15% in flow rate (Susset, 1983). Variations in specific gravity of the fluid voided (infusant when doing UDS studies) can affect the calculated flow rate. Most systems allow for calibrations for various fluids such as radiographic contract.
Electromyography
Muscle depolarization must be detected by an electrode placed in or near the muscle. Several different methods are available to do this including surface electrodes and needle electrodes (the two most common methods), as well as anal plug or urethral catheter–mounted electrodes (O’Donnell, 1998). Surface electrodes are self-adhesive skin patch electrodes that are applied over the skin of the anal sphincter. Except in some neurologic diseases, the external anal sphincter EMG will be the same as the external urethral sphincter EMG. Surface electrodes have a significant advantage compared with the needle electrode regarding patient convenience and comfort. However, the surface electrodes provide an inferior signal source and must be precisely placed to provide an adequate signal source. Most clinicians feel that the concentric needle electrode is the superior technique for obtaining a signal source of EMG activity of the striated external sphincter muscles. Compared with the surface electrode, placement of the needle electrode has the disadvantage of being uncomfortable for the patient, especially if more than one attempt at placement of the electrode is required to obtain an adequate signal. Also, the needle electrode is easily dislodged and may require replacement during the study. Patients typically have a low tolerance for replacement of the needle electrode during urodynamic studies. The performance of an EMG and the selection of the type of electrode to be used depend on the UDS question to be answered.
Filling and Storage Phase
The CMG assesses the bladder’s response to filling. The CMG can measure filling pressure, sensation, involuntary contractions, compliance, and capacity. Sensation is the part of cystometry that is truly subjective and therefore requires an alert and attentive patient and clinician. Several subjective parameters that can be recorded during filling are recognized by the ICS (see Table 62–1).
Normal Filling and Storage
Normally, the bladder should store urine at low pressure and not contract involuntarily. Once capacity is reached or voluntary voiding is desired, intravesical pressure will increase (voluntary detrusor contraction). In actuality this is preceded by a relaxation of the external sphincter. A normalized adult CMG is shown in Figure 62–3. Normally detrusor pressure should remain near zero during the entire filling cycle until voluntary voiding is initiated. That means baseline pressure stays constant (and low) and there are no involuntary contractions.
Abnormalities of Bladder Filling—Detrusor Overactivity and Impaired Compliance
During filling, involuntary detrusor contractions (IDCs) can occur. These are often associated with urgency and even urgency incontinence. Detrusor overactivity (DO) is a urodynamic observation characterized by IDCs during the filling phase, which may be spontaneous or provoked (Fig. 62–4). DO may be further characterized as neurogenic DO, which means it is associated with a relevant neurologic condition (e.g., spinal cord injury, multiple sclerosis) or idiopathic DO, which means there is “no defined cause” (non-neurogenic) (Abrams et al, 2002). The term idiopathic is a bit of a misnomer in that the cause of DO in a non-neurogenic patient may be readily apparent (e.g., bladder outlet obstruction, inflammatory process) or may be truly “unknown.” Thus from a practical standpoint, the terms neurogenic and non-neurogenic DO make sense but do not fit the ICS definitions. (It should be noted that the term neurogenic DO replaced the term detrusor hyperreflexia, and the term idiopathic DO replaced the term detrusor instability in previous ICS terminology). Neurogenic and idiopathic DO may look identical on CMG. These terms are strictly defined by the patient’s neurologic status and not the CMG appearance of the IDCs.
The presence of DO during UDS must be interpreted in the context of the patient’s symptoms and condition. Ideally, a patient’s symptoms should be reproduced during UDS, so we would expect DO to be accompanied by urgency or urgency incontinence, although it can occur and be significant without being symptomatic, particularly in neurogenic DO. However, DO can also be “test induced” or clinically insignificant. It has been reported in 14% to 18% of healthy asymptomatic volunteers undergoing UDS (van Waalwijk van Doorn et al, 1992; Robertson, 1999; Wyndaele et al, 2002). This is even more dramatic in ambulatory UDS studies where DO has been found in up to 69% of asymptomatic females (van Waalwijk van Doorn, et al, 1996). Conversely, failure to demonstrate DO on UDS does not rule out its existence. It is well known that up to 50% of women with urgency incontinence do not demonstrate DO on UDS. However, the ability to suppress DO during UDS test may in and of itself be significant. For example, Osman (2003) showed that patients with mixed incontinence and a normal CMG (no DO) not only had excellent cure rates for stress incontinence but also had an 87% cure rate for urgency incontinence (compared with only 43% cure for urgency incontinence for women randomized to receive antimuscarinic medication instead of stress incontinence surgery).
In addition to the presence of DO, its characteristics can be noted. DO can be observed as a single event or as multiple IDCs. It can be phasic (continuous), sporadic, or terminal (occurring at the end of filling near capacity). It can also be suppressed or nonabortable and may lead to leakage or precipitant micturition. Classifying DO in such a way can be valuable in certain circumstances. For example, overactivity bladder symptoms associated with obstruction have been shown to have a higher likelihood of resolving with intervention (e.g., transurethral resection of the prostate [TURP]) when DO occurs as a single terminal IDC rather than continuous or sporadic IDCs (Kageyama et al, 2000).
The viscoelastic properties of the bladder, on the basis of its composition of smooth muscle, collagen, and elastin, normally produce a highly compliant structure. Therefore as the bladder fills there is little change in pressure (see normalized CMG Fig. 62–3). Compliance is the relationship between change in bladder volume and change in detrusor pressure (Δ volume/Δ pressure) and is measured in mL/cm H2O. The ICS recommends two standard points, the Pdet at the start of bladder filling (usually zero) and the Pdet at cystometric capacity or before the start of any detrusor contraction that results in significant leakage (Abrams et al, 2002). Both points are measured excluding any detrusor contractions. It is difficult to define what “normal compliance” is in terms of mL/cm H2O. Several authors have shown that mean values for compliance in healthy subjects range from 46 to 124 mL/cm H2O (Sorensen et al, 1988; van Waalwijk van Doorn et al, 1992; Hosker, 2004). However, there is great variation. For example, van Waalwijk van Doorn and colleagues (1992) showed a variation of compliance from 11 to 150 mL/cm H2O (mean 46) in 17 healthy subjects. Some of the variation in “normal” is likely due to the fact that compliance per se is dependent on bladder capacity. Furthermore, various definitions of impaired compliance have been used (e.g., between 10 and 20 mL/cm H2O); however, there is no consistent definition based on mL/cm H2O. Stohrer and colleagues (1999) suggest that a value of less than 20 mL/cm H2O is consistent with impaired compliance and implies a poorly accommodating bladder. However, examples in which this may not be the case can be cited (e.g., small cystometric capacity). Therefore in practical terms, absolute pressure is probably more useful than a “compliance number” or value. For example, it has been shown that storage greater than 40 cm H2O is associated with harmful effects on the upper tracts (McGuire et al, 1981) (Fig. 62–5). Also, depending on the clinical scenario, a particular compliance in terms of mL/cm H2O can mean different things (Fig. 62–6). As a general rule, prolonged storage at high pressures can lead to upper tract deterioration. Elevated storage pressures and impaired compliance should be interpreted in the context of the clinical scenario. It appears that conventional cystometry may provoke filling pressures higher than natural filling in some cases. Robertson (1999) showed that for six patients with neuropathic bladder and severely impaired compliance on conventional cystometry, compliance was actually normal on ambulatory monitoring with natural filling.
Impaired compliance is seen in a variety of neurologic conditions (spinal cord injury/lesion, spina bifida) and usually results from increased outlet resistance (e.g., detrusor-external sphincter dyssynergia [DESD]) or decentralization in the case of lower motor neuron lesions. It can also result from long-term bladder outlet obstruction (e.g., BPH) (Leng and McGuire, 2003) or structural changes like radiation cystitis or tuberculosis.
Key Points: Abnormalities of Bladder Filling
Leak Point Pressures
ALPP is a measure of sphincteric strength or the ability of the sphincter to resist changes in abdominal pressure (McGuire et al, 1993). ALPP is defined as the intravesical pressure at which urine leakage occurs due to increased abdominal pressure in the absence of a detrusor contraction (Abrams et al, 2002). This measure of intrinsic urethral function is applicable to patients with stress incontinence. An ALPP can only be demonstrated in a patient with stress urinary incontinence (SUI). Conceptually, the lower the ALPP, the weaker the sphincter. There is no normal ALPP because patients without stress incontinence will not leak any physiologic abdominal pressure. ALPP should be measured as the total abdominal pressure required to cause leakage, not the change in pressure (McGuire et al, 1993). Therefore if ALPP is measured in the standing position, it should include the baseline Pabd (or Pves), which is usually about 20 to 40 cm H2O. Classically, the reading is taken from the Pves channel as long as there is no involuntary contraction (Fig. 62–7). In cases where patients do not leak with a urethral catheter in place, the ALPP can be measured from the abdominal pressure channel either rectally or vaginally (Stohrer et al, 1999). The original description of ALPP was done at an arbitrary bladder volume of 150 milliliters; however, in some cases it is necessary to fill the bladder more. The volume at which ALPP is determined should be noted as some investigators have found that it decreases at higher volumes (Faerber and Vashi, 1998). As a general rule, one should start testing at 150 mL and then every 50 mL thereafter until SUI is demonstrated. If there is no SUI demonstrated at capacity, the urethral catheter is removed and ALPP is measured via the rectal catheter (provided there is no increase in Pdet from DO or impaired compliance).
Current technology does not permit a method to distinguish between ISD in the face of urethral hypermobility in women. Therefore although these ALPP values are often used as guidelines, they should be interpreted with caution. For example, if there is no urethral hypermobility, SUI must be caused by ISD, regardless of the ALPP. Furthermore, Fleischmann and colleagues (2003) found that urethral hypermobility was equally common in women with lower versus higher ALPP. ISD and urethral hypermobility may coexist, and they do not define discrete classes of patients with stress urinary incontinence. Thus an isolated measure of ALPP without considering other factors such as cystometry and urethral mobility is of limited utility in predicting success for commonly performed female SUI procedures (Hosker et al, 2009).
The term ALPP has been used interchangeably with “Valsalva” leak point pressure (VLPP); however, this is not entirely correct. An ALPP can be measured during UDS testing by a voluntary Valsalva maneuver (VLPP) or by a cough (cough leak point pressure or CLPP). In the same person, VLPP tends to be significantly lower than CLPP. Therefore exact terminology and methods should be used when describing an ALPP. ALPP can also be influenced by the presence or the size of a urethral catheter (Bump et al, 1995; Huckabay et al, 2005; Türker et al, 2010). It has been shown in women with SUI that the larger the catheter, the lower the ALPP. ALPP can also be measured without a urethral catheter by assessing the abdominal pressure via a rectal (or vaginal) catheter. It has been shown that 15% of women with SUI (Türker et al, 2010) and 35% of men with postprostatectomy SUI (Huckabay et al, 2005) will demonstrate an ALPP only with the urethral catheter removed.
The second type of leak point pressure is the detrusor leak point pressure (DLPP), which is a measure of detrusor pressure in patients with decreased bladder compliance. It is defined as the lowest detrusor pressure at which urine leakage occurs in the absence of either a detrusor contraction or increased abdominal pressure (Abrams et al, 2002) (see Fig. 62–5). The higher the urethral resistance, the higher the DLPP. One can imagine that in a poorly compliant bladder, if outlet resistance is low, incontinence will occur at a relatively low or “safe” pressure. However, if outlet resistance is high, the pressure in the bladder will continue to increase as the bladder fills. There is potentially less incontinence, but eventually the pressure is transmitted to the upper tracts (Fig. 62–8).
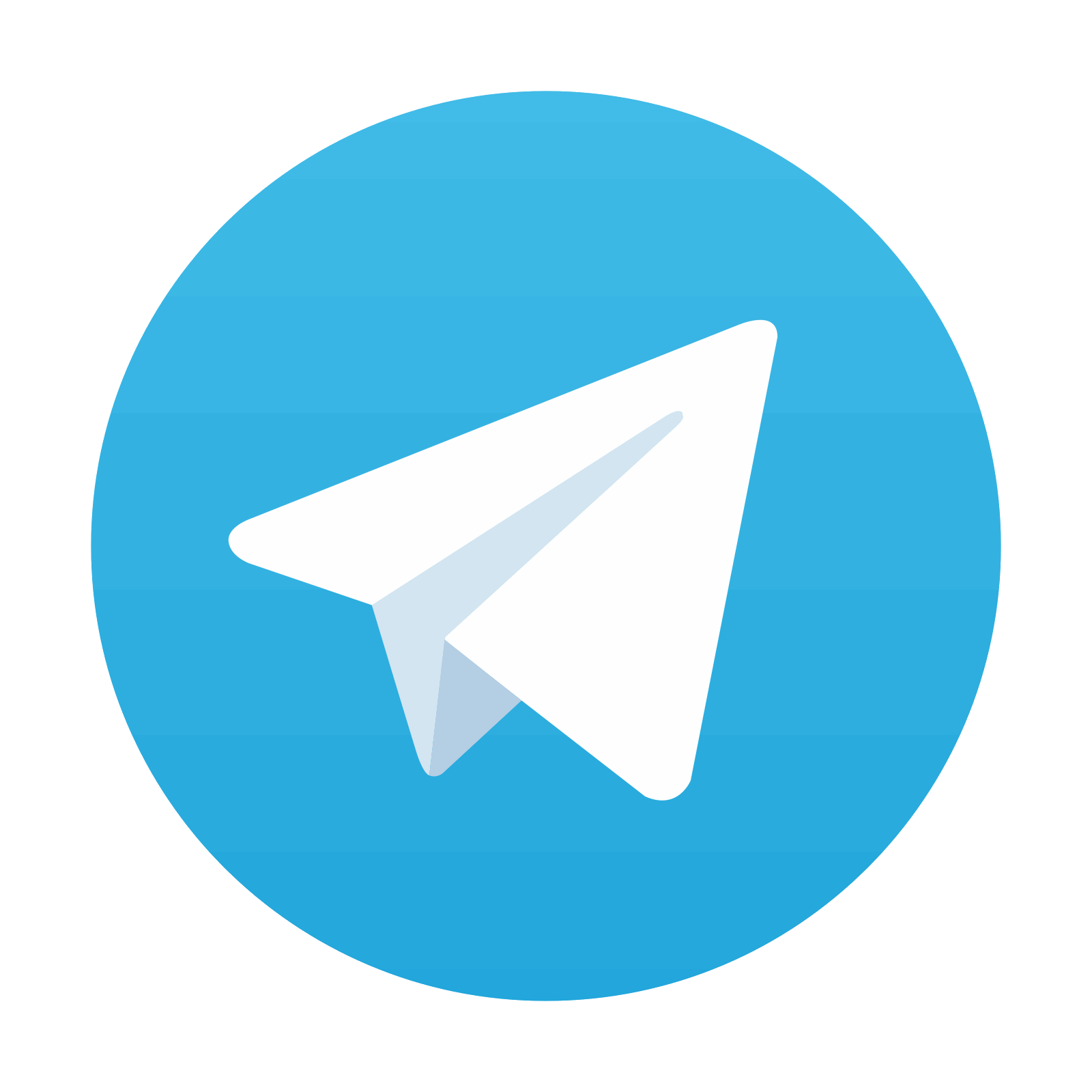
Stay updated, free articles. Join our Telegram channel

Full access? Get Clinical Tree
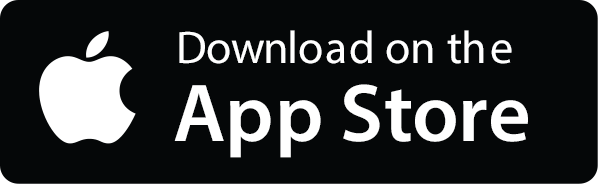
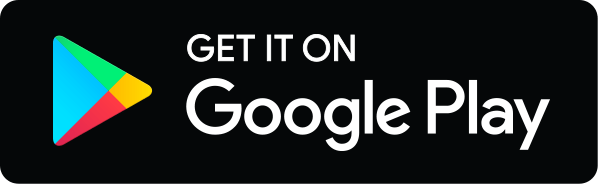