ANEMIA AND END-STAGE KIDNEY DISEASE: THE MAGNITUDE OF THE PROBLEM
Anemia, or reduced erythrocyte mass, is one of the most clinically significant complications of chronic kidney disease (CKD) and is associated with increased mortality and decreased quality of life. Although there is no universal definition of anemia, recent guidelines recommend diagnosing anemia in adult men with a hemoglobin (Hb) <13.0 g/dL and in adult females with an Hb <12.0 g/dL (1). Anemia is highly prevalent among patients with CKD and end-stage kidney disease (ESKD), with an increase in the rate and severity of anemia associated with a decrease in glomerular filtration rate (GFR) and the near universal prevalence of anemia in patients on chronic dialysis (2,3). Recent data from the National Health and Nutrition Examination Survey (NHANES) demonstrated a prevalence of anemia in predialysis patients with CKD stage 5 of 53.4%. The prevalence of anemia increases as patients initiate chronic dialysis therapy, with 85% of patients on chronic dialysis reported to have an Hb <12.0 g/dL by the 2014 United States Renal Data System (USRDS) annual report (4).
Numerous observational studies have shown that the presence and severity of anemia correlate with numerous adverse outcomes including increased mortality (5–7), left ventricular hypertrophy (LVH) (8,9), and decreased health-related quality of life (10,11). However, randomized control trials have not demonstrated a mortality benefit for the treatment of anemia with the use of erythropoiesis-stimulating agents (ESAs) in patients with CKD or ESKD, and some studies even suggest harm.
Despite the overwhelming prevalence of anemia in patients with ESKD and the clear association of anemia with significant morbidity and mortality, the degree to which anemia should be corrected and the optimal strategy for doing so is controversial. This chapter focuses on the treatment of anemia in patients with ESKD. We first review normal erythrocyte production and the normal response to anemia followed by a discussion of the various pathophysiological processes which lead to anemia in patients with ESKD before discussing treatment directly, as an understanding of the former topics are vital to successfully employing the latter.
NORMAL ERYTHROCYTE PRODUCTION
Under normal conditions, approximately 1% of circulating erythrocytes are replaced daily. Erythrocyte production occurs in the bone marrow and depends on erythropoietin (EPO), a hormone which is mainly produced in the kidneys, and regulates erythropoiesis via binding to EPO receptors on erythroid precursor cells located in the bone marrow. The proper functioning of this process requires additional cofactors including iron, vitamin B12, and folic acid.
EPO is the major regulatory hormone of erythropoiesis and is produced primarily in peritubular interstitial cells of the kidney with lesser production in the liver postnatally (12,13). EPO production is modulated by the delivery of oxygen from circulating erythrocytes; in the presence of tissue hypoxia, EPO production is increased. On a cellular level, this process occurs due to the presence of hypoxia-inducible factors (HIFs) in EPO-producing cells, specifically HIFα and HIFβ (14). Under normoxic conditions, HIFα is constitutively produced and subsequently degraded by the ubiquitin-proteasome system. This degradation is dependent on the hydroxylation of HIFα via specific hydroxylase enzymes, which allow for the binding of HIFα to the tumor suppressor protein von Hippel-Lindau (VHL). This leads to the HIFα molecule being tagged for proteasomal degradation. Under hypoxic conditions, HIFα degradation is inhibited, allowing for the successful production of EPO. EPO is then secreted into the systemic circulation where it binds with EPO receptors on erythroid precursor cells in the bone marrow, leading to increased production of erythrocytes by preventing erythroid cell apoptosis (15).
In order for successful erythropoiesis to occur, an adequate supply of iron, B12, and folate are necessary. Iron balance is a highly regulated process, and iron deficiency, either absolute or functional, significantly contributes to anemia of CKD. The successful production of erythrocytes depends on both adequate nutritional iron stores and the mobilization of these stores for use by the bone marrow. The master regulator of this process is hepcidin (16,17). Elevated hepcidin levels inhibit duodenal iron absorption and iron release from macrophages, whereas decreased hepcidin levels promote gastrointestinal (GI) absorption and mobilization from macrophages. Hepcidin production is stimulated by total body iron overload and infection (18).
UNDERLYING ETIOLOGIES OF ANEMIA OF CHRONIC KIDNEY DISEASE
Anemia in patients with CKD is, as a rule, a multifactorial process. While EPO deficiency is usually the primary pathophysiological process (19), iron deficiency anemia related to chronic blood loss and anemia of chronic disease related to comorbidity burden and the malnutrition–inflammation complex syndrome (20) both play significant contributory roles. Other causes for anemia found in the general population can also be superimposed on the above processes and should be considered when evaluating a patient with CKD and anemia.
In healthy patients, EPO levels increase in response to anemia. In patients with CKD, there is an inadequate response, and EPO levels are inappropriately low relative to the degree of anemia (21,22). While patients with CKD and ESKD (including anephric patients) continue to produce EPO and have been shown to increase EPO production (23), the response in EPO production is blunted and inadequate to sufficiently correct the anemia. The explanation for inadequate EPO production has historically implicated reduced nephron mass and loss of EPO-producing peritubular interstitial cells. However, recent evidence including clinical trials that have demonstrated that EPO production can be increased in patients with ESKD via pharmacological stabilization of HIF using prolyl hydroxylase inhibitors (24) raises the possibility that a disturbed oxygen-sensing mechanism rather than a lack of capacity for EPO production may be the primary cause of anemia.
ESKD and chronic hemodialysis (HD) inevitably lead to negative iron balance, primarily as a result of chronic blood loss through several different mechanisms. Patients with ESKD are more likely to have both overt and occult GI bleeding (25,26). Additionally, significant blood loss occurs both due to frequent phlebotomy as well as due to the HD procedure itself (27). In addition to absolute iron deficiency, patients with ESKD have a frequently impaired ability to absorb iron or utilize their existent iron stores due to ongoing inflammation (20).
There are several other processes that may contribute to anemia in patients with CKD that are worth mentioning. It has been known for some time that patients with CKD have a shortened erythrocyte survival time (28). Neither the precise pathophysiological cause of this phenomenon nor the degree to which this shortened survival contributes to anemia is entirely clear. However, it is unlikely that dialysis modality plays a significant role, as studies have shown no significant difference in the decrease in erythrocyte survival time between peritoneal dialysis, conventional thrice-weekly HD, nocturnal HD, or short daily HD (29,30). Furthermore, while the toxic uremic milieu is frequently cited as the probable cause for shortened erythrocyte survival, more frequent HD has shown no benefit in improving anemia (31).
Folate and vitamin B12 deficiency, while not common, are both readily reversible causes of anemia. These diagnoses should be tested for, particularly in the setting of macrocytosis. While there is loss of folate with dialysis, normal dietary intake should exceed such losses (32).
Historically, aluminum toxicity was a significant cause of anemia in patients with ESKD on dialysis, both due to the use of aluminum-containing phosphate binders and due to the presence of aluminum in water used for dialysis (33). Its incidence has waned significantly due to the now routine removal of aluminum from dialysis water and due to the wide availability of non–aluminum-containing phosphate binders. Aluminum toxicity–related anemia presents as a microcytic anemia in the presence of adequate iron stores (34).
Given the universal polypharmacy of patients with ESKD, it is important to consider medications and their potentially contributory role to anemia. The myelosuppressive effects of immunosuppressants, commonly used in the setting of solid organ transplants or systemic autoimmune conditions, are well known and well described. Angiotensin-converting enzyme (ACE) inhibitors and angiotensin receptor blockers (ARBs) have also been causally implicated in CKD-related anemia, although the evidence is primarily composed of case reports and small case series (35–37). Experimental data in rats demonstrates that angiotensin plays a role in EPO production (38), and it is therefore plausible that angiotensin blockade would impair EPO production and lead to anemia. While observational studies in patients on chronic dialysis have shown mixed results regarding the relationship between ACE inhibitor and ARB use with ESA dose (39,40), several uncontrolled studies did show the need for increased ESA dosing in patients receiving ACE inhibitors or ARBs (41,42).
TREATMENT OF ANEMIA OF CHRONIC KIDNEY DISEASE
The initial step in the treatment of anemia in patients with ESKD involves a basic diagnostic workup to identify the numerous processes that may be contributing to the anemia. Recent Kidney Disease Improving Global Outcomes (KDIGO) guidelines recommend that initial testing for patients with CKD and anemia includes complete blood count (CBC), absolute reticulocyte count, serum ferritin level, serum transferrin saturation (TSAT), and serum vitamin B12 and folate levels (1).
While tests for serum EPO levels are commercially available, these tests are not recommended since they are unlikely to affect clinical care given the lack of correlation between absolute EPO levels and the degree of anemia in patients with a creatinine clearance of less than 40 mL/min (43). As such, it can be assumed that all patients with advanced CKD and ESKD have EPO deficiency; however, it is recommended to first treat any correctable causes of anemia prior to initiating treatment with ESAs (1). This recommendation is predicated upon the fact that ESA responsiveness is unlikely in patients with additional causes of hypoproliferative anemia such as iron, vitamin B12, or folate deficiency. Furthermore, correction of these underlying issues can significantly reduce the dose of or need for ESA use. In particular, iron deficiency is exceedingly common in patients with CKD and ESKD, and numerous randomized studies have demonstrated that iron supplementation reduces ESA requirements (44–48).
DETERMINATION OF IRON STATUS
In healthy patients with an isolated finding of anemia, the diagnosis of iron deficiency anemia is generally clinically obvious and determined by the presence of numerous consonant factors including a low-reticulocyte index, low mean corpuscular volume (MCV), decreased iron stores based on decreased serum ferritin levels, and decreased iron availability based on decreased serum TSAT. In patients with anemia of CKD, straightforward determination of iron status is generally the exception rather than the rule. Iron stores may be present but not available for erythropoiesis, and serum ferritin levels are frequently normal or even increased without a concomitant increase in serum TSAT or even a decreased TSAT. Despite this laboratory discrepancy in iron status, studies have demonstrated that the administration of supplemental iron to patients with elevated ferritin levels can still improve the anemia (48,49), rendering serum ferritin a rather poor marker of ESA response. Given the complexity with accurately determining the iron status of patients with CKD, numerous additional serologic tests have been explored, including erythrocyte zinc protoporphyrin (50), percentage of hypochromic red blood cells (51), reticulocyte Hb content (52), and serum hepcidin (53). Thus far, none of these tests have been incorporated into routine clinical practice due to a lack of consistent results over numerous studies, lack of commercial availability, or lack of utility in meaningfully impacting current clinical practice. For now, guidelines recommend determining iron status by serum ferritin and TSAT alone; ultimately, when uncertainty still remains, iron status is determined by the administration of supplemental iron and the presence or absence of an erythropoietic response.
TREATMENT OF IRON DEFICIENCY
Therapeutic Targets
The treatment of anemia in patients with ESKD almost always involves both the use of intravenous (IV) iron and the use of ESAs, and an increased dose in the first of these agents generally allows for a reduced dose in the last. Safety concerns related to ESA use, shifting market pressures, and small studies demonstrating the ability to decrease ESA use by targeting higher levels of ferritin and TSAT have led to changing serum iron marker targets over time. The 2006 Kidney Disease Outcomes Quality Initiative (KDOQI) guidelines recommended administering IV iron to achieve a serum ferritin greater than 200 ng/mL and a TSAT greater 20% (54). The 2012 KDIGO guidelines recommend the administration of IV iron if serum ferritin is less than 500 ng/mL or TSAT is less than 30% and an increase in Hb or decrease in ESA dose is desired (1). Routine administration of iron to patients with serum ferritin levels greater than 500 ng/mL or TSAT greater than 30% is recommended against due to a lack of data demonstrating the safety of this approach, but this may be considered on an individual patient basis, particularly when a reduction in ESA dose is desired.
Two randomized control studies specifically evaluated the use of IV iron to achieve different iron targets and its effect on anemia parameters. A study by Besarab et al. (48) randomized 43 patients to receive maintenance IV iron dextran to maintain TSAT 20% to 30% versus increased doses of IV iron dextran to achieve TSAT 30% to 50% over 6 months and demonstrated comparable Hb levels in both groups with increased ferritin levels and a 40% decrease in ESA dosing in the increased IV iron group. The Dialysis Patients’ Response to IV Iron with Elevated Ferritin (DRIVE) study by Coyne et al. (49) randomized 134 patients with a serum ferritin of 500 to 1,200 ng/mL, and a TSAT <25% over a 6-week period to 1 g of IV ferric gluconate administered with HD over eight doses versus placebo while receiving EPO at a 25% increased dose from baseline with further titration prohibited over the duration of the study. Results demonstrated a 0.5 g/dL increase in Hb in the IV ferric gluconate group versus the placebo group.
While the above studies demonstrate that iron supplementation can improve anemia parameters even in patients with significantly elevated iron stores, there are no studies adequately powered with long enough follow-up to demonstrate long-term benefit or harm related to employing a strategy that favors the administration of iron over the use of ESAs (55).
Route of Administration
There is general agreement that IV iron is the preferred route of iron administration to patients with ESKD and that oral iron is inadequate to replete deficient iron stores. A recent meta-analysis of 28 studies involving 2,098 subjects demonstrated increased ferritin, increased TSAT, increased Hb, and decreased ESA dose with the use of IV versus oral iron (56). The accepted explanation for reduced effectiveness of oral iron has implicated elevated hepcidin levels from chronic inflammation leading to poor GI absorption. Interestingly, recent trials involving ferric citrate, an oral iron-containing phosphate binder, have demonstrated a decreased need for IV iron and ESA doses (57). It remains to be seen how the use of oral iron-containing phosphate binders will impact the overall management of anemia in patients with CKD.
Parenteral Iron Preparations
Sixty-two percent of patients with ESKD on HD regularly received IV iron in 2012 according to data from the USRDS (4). There are currently several parenteral iron preparations available for use in the United States: iron dextran, iron sucrose, ferric gluconate, ferric carboxymaltose, and ferumoxytol. Iron isomaltoside is available for use in Europe but is not currently U.S. Food and Drug Administration (FDA) approved. Data from Dialysis Outcomes and Practice Pattern Study (DOPPS) demonstrate that approximately 90% of IV iron administered in the United States in 2015 was an iron sucrose formulation, with the remainder consisting almost entirely of ferric gluconate (58).
Most IV iron preparations consist of iron associated with a carbohydrate shell. The infusion of IV iron and the release of free iron can lead to a range of anaphylactoid and other reactions including myalgias, arthralgias, facial flushing, and hypotension, although there is a relatively low risk for the occurrence of serious adverse events (59), with a predicted absolute risk of life-threatening events ranging from 0.6 to 11.3 events per million depending on the IV iron preparation used (60). Details of the various IV iron preparations are discussed below (TABLE 29.1).
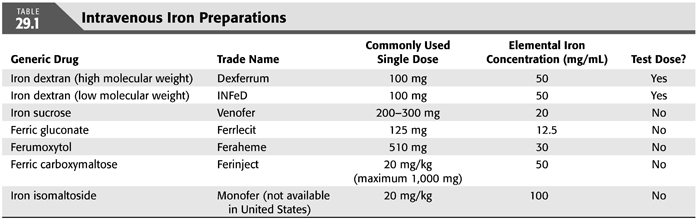
Iron Dextran
Iron dextran is available in the United States in both a high molecular weight formulation (Dexferrum) and a low molecular weight formulation (INFeD). While KDIGO guidelines strongly recommend close monitoring of all patients during their initial dose of iron dextran (1) due to an increased risk of severe anaphylactic reactions, several studies suggest that the increased risk of severe anaphylactic reactions is limited largely to the use of high molecular weight formulations (61,62). The use of low molecular weight iron dextran appears to have an equivalent risk of adverse reactions to other commonly used IV iron preparations (63). Iron dextran is available in 1- or 2-mL solutions of 50 mg/mL. The package insert recommends administration of a test dose of 25 mg prior to the administration of a full dose. Furthermore, KDIGO guidelines recommend that patients be monitored for 60 minutes after their initial infusion of iron dextran and that resuscitative personnel be available at the time of infusion. While the package insert recommends a maximum dose of 100 mg daily, the administration of 1,000 mg of iron dextran over 1 hour has been shown to be safe and effective (64).
Iron Sucrose
Iron sucrose (Venofer) is widely used in the United States and worldwide for the treatment of CKD-associated anemia. It appears to have a superior safety profile to iron dextran and an equivalent safety and efficacy profile to other IV iron preparations (65,66). It is available in 2.5-, 5-, or 10-mL solutions of 20 mg/mL. The package insert states that there is no need for a test dose in product-naive patients. KDIGO guidelines suggest monitoring patients for 60 minutes after the initial infusion of iron sucrose but mention that the data to support this suggestion is not strong. The package insert states that a dose of up to 200 mg can be given over a 2- to 5-minute IV infusion. Doses of 300 mg should be given over 1.5 hours. It is not recommended to administer doses above 300 mg, as this has been associated with a significant rate of adverse reactions including dizziness, nausea, and hypotension (67).
Ferric Gluconate
Ferric gluconate (Ferrlecit) was available for use in Europe for many decades before being FDA approved in 1999. It is safer than iron dextran with comparable safety and efficacy to other IV iron preparations (68,69). It is available in 5-mL solutions of 12.5 mg/mL. The package insert states that prior labeling recommended a test dose of 2 mL, but a test dose is no longer recommended. KDIGO guidelines suggest monitoring patients for 60 minutes after the initial infusion of ferric gluconate but mention that the data to support this suggestion is not strong. The package insert recommends a maximum dose of 125 mg which can be infused over 1 hour, although the infusion of 250 mg over 1 hour appears to be safe (70). There is little data available to support the safety of administering larger one-time doses.
Ferumoxytol
Ferumoxytol (Feraheme) was FDA approved for the treatment of anemia in patients with CKD in 2009. It possess supermagnetic properties and can affect magnetic resonance imaging (MRI) for days to months after its administration (71). Randomized trials have demonstrated ferumoxytol to have comparable efficacy and safety to other IV iron preparations (65,72). It is available in 17-mL solutions of 510 mg/17 mL. The package insert does not indicate the need for a test dose but recommends patient monitoring for at least 30 minutes after infusion in a setting where proper personnel and therapies are immediately available. While ferumoxytol was initially recommended to be administered as a rapid injection of 510 mg over 17 to 60 seconds, this was suggested to lead to an increased rate of anaphylactic reactions (73); subsequent recommendations suggest administering ferumoxytol in a diluted form over a slow infusion of at least 15 minutes. Although the maximum recommended dose is 510 mg, administering 1,020 mg as a one-time dose has been shown to be safe and efficacious (74).
Ferric Carboxymaltose
Ferric carboxymaltose (Injectafer) was FDA approved for anemia in non–dialysis-dependent CKD in 2013. Several randomized trials demonstrated superior efficacy with comparable safety of ferric carboxymaltose versus oral iron in patients with anemia not related to CKD (75–77). Additionally, one randomized trial demonstrated superior efficacy with comparable safety of ferric carboxymaltose versus oral iron in patients with non–dialysis-dependent CKD (78), and one randomized trial demonstrated comparable efficacy and safety between two injections of 750 mg of ferric carboxymaltose given over 1 week versus five injections of 200 mg of iron sucrose given over 2 weeks in patients with non–dialysis-dependent CKD (79). There is very little published data available at this time evaluating the safety and efficacy of ferric carboxymaltose in patients with ESKD. Ferric carboxymaltose is available in 15-mL solutions of 750 mg/15 mL. There is no mention of requiring a test dose in the package insert, and there is no specific recommendation by the FDA to monitor patients after their initial infusion. Of note, ferric carboxymaltose infusion was associated with transient hypertensive episodes (79).
Iron Isomaltoside
Iron isomaltoside (Monofer) is currently not FDA approved and is only in use outside of the United States. It can be administered as a 15-minute infusion with doses of >1,000 mg given at one time.
Iron Loading and Maintenance Therapy
There are generally two phases in the treatment of iron deficiency in anemic patients with ESKD: iron loading and maintenance therapy. Iron loading is the use of IV iron to correct iron deficiency. Maintenance therapy is the ongoing administration of IV iron in iron-replete patients who are usually receiving concurrent ESA therapy. As discussed earlier, current guidelines (1) recommend administering IV iron to patients with a TSAT <30% and a ferritin <500 ng/dL when an increase in Hb is desired. Most iron loading regimens recommend the intensive administration of approximately 1,000 mg of IV iron over a relatively short time period. This can be done over relatively few treatments with the use of iron dextran, ferumoxytol, or ferric carboxymaltose but would require more treatments with the use of iron sucrose and ferric gluconate. During the iron loading phase, it is recommended to check the CBC, TSAT, and ferritin frequently to ensure that the treatment is achieving its goals. It is recommended to ensure that iron stores are replete prior to initiating ESA therapy, as the administration of an ESA to an iron deficient patient has a high probability of failure. Once iron stores are replete and Hb and iron indices are stable, laboratory test results can be checked every 3 months (1).
Once iron stores are replete, there are two possible strategies regarding maintenance therapy: intermittent dosing of IV iron when iron indices fall below target or continuous dosing of IV iron to maintain iron indices above target. Theoretically, continuous dosing of IV iron is more likely to ensure that iron stores remain adequate, allowing for the possibility of continued, effective erythropoiesis (80). Several randomized trials evaluated the use of continuous IV iron versus intermittent administration in iron-replete patients and demonstrated superiority of a continuous dosing regimen with either a higher Hb achieved or an equivalent Hb with a reduced ESA dose (81,82).
Maintenance Therapy via Iron-Containing Dialysate
A newly tested method of maintenance administration of IV iron to patients with ESKD who are iron replete involves the addition of ferric pyrophosphate citrate (FPC) (Triferic), a water-soluble iron salt, to the dialysis solution, allowing for routine administration of low doses of IV iron with each dialysis treatment. Two identical randomized trials, Continuous Replacement Using Iron Soluble Equivalents (CRUISE) 1 and 2 (83), evaluated the effects of the use of dialysate containing 2 μmol/L of FPC iron versus standard dialysate in 599 patients on a fixed dose of ESA with prohibited supplemental iron use and demonstrated that FPC-containing dialysate maintained steady Hb levels with less of a decrease in ferritin levels versus the placebo group. An additional randomized trial, Physiological Replenishment Iron Maintenance Equivalency (PRIME) (84), evaluated the use of dialysate containing 2 μmol/L FPC iron versus standard dialysate in 103 patients on adjustable doses of ESAs and IV iron to be administered when serum ferritin levels decreased below 200 μg/L and demonstrated a decrease in ESA dose and decrease in IV iron need versus placebo. FPC (Triferic) was FDA approved as an iron replacement product to maintain Hb in adult patients with HD-dependent CKD in January 2015.
Adverse Effects of Intravenous Iron
As discussed earlier, all IV iron preparations are associated with anaphylactic-type reactions which at times can be life threatening. Excluding high molecular weight iron dextran which carries an increased risk, the absolute risk of a life-threatening serious adverse event has been estimated to be approximately 1 in 200,000 with commonly used IV iron preparations (60).
Theoretically, an iron-rich environment can promote bacterial growth, and the administration of IV iron to patients with active infection is contraindicated (1). Despite this recommendation, the evidence linking the administration of IV iron to an increased infection risk is mixed and of low quality. A retrospective study of 32,566 patients (85) showed no association between IV iron dose and infection-related mortality; a prospective, observational study of 985 patients (86) showed no association between IV iron use and infection risk; a retrospective study of 117,050 patients (87) demonstrated an increased risk of infection-related hospitalization in patients receiving higher dose intermittent versus lower dose maintenance IV iron therapy; a meta-analysis of 72 randomized controlled trials involving 10,605 patients (88) demonstrated an increased risk of infection with the use of IV versus oral or no iron; a retrospective study of 14,078 patients (89) showed no significant increased risk of infection-related mortality with IV iron dose but did demonstrate a nonsignificant trend of increased infection-related mortality in patients receiving >1,050 mg of IV iron over 3 months or >2,100 mg IV iron over 6 months; and a retrospective study of 22,820 patients on chronic HD (90) demonstrated that IV iron administration in the setting of hospitalization for infection was not associated with an increased risk of readmission for infection, increased length of stay, or mortality. A recent trial by Agarwal et al. (91) randomized 136 patients with CKD stage 3 or CKD stage 4 to IV versus oral iron and demonstrated no significant difference in serious adverse events between the two groups. However, there was incomplete randomization, and adjustment after randomization did demonstrate a significant increase in the adjusted risk of serious adverse events including infection-related adverse events in patients receiving IV iron versus oral iron. Given the incomplete randomization, the findings of this study are difficult to interpret.
The relationship between IV iron use and overall mortality is not entirely clear at this time. A retrospective study of 32,566 patients (85) showed no association between IV iron dose and overall mortality; a retrospective study of 32,435 patients (92) demonstrated an increased risk of mortality in those receiving >300 mg/mo of IV iron; a prospective study of 58,058 patients (93) demonstrated a decreased risk of overall mortality with the use of up to 400 mg/mo of IV iron versus no iron but an increased risk of mortality with a dose of >400 mg/mo of IV iron; and a retrospective study of 14,078 patients (89) showed a decreased risk of overall mortality with the use of 150 to 350 mg/mo or >350 mg/mo of IV iron compared to 0 to150 mg/mo.
A paucity of adequately powered randomized trials of sufficient duration makes it difficult to evaluate the long-term risks and benefits of IV iron use at this time.
ERYTHROPOIESIS-STIMULATING AGENTS
Historically, anemia was an inevitable consequence of ESKD and was only treatable by regular blood transfusions, presenting a significant source of morbidity in patients with ESKD. With the advent of recombinant human erythropoietin (rHuEPO) in the 1980s, anemia became a treatable problem, with the potential of improving Hb to normal levels. Over time, additional drugs have been developed which are either derivatives of the EPO molecule or are different molecules which can directly or indirectly stimulate the EPO receptor. These medications are all grouped under the class of drugs commonly referred to as ESAs.
Epoetin
Epoetin is a term generally applied to rHuEPO preparations produced using recombinant technology via the overexpression of the human EPO gene in mammalian cell lines. Epoetin-α and epoetin-β were the first two of these compounds developed, and both are produced in Chinese hamster ovary cells. Epoetin-α is the only epoetin currently available in the United States. Several nonrandomized trials in the late 1980s demonstrated the ability of rHuEPO to increase Hb levels in patients with ESKD (94–97), leading to FDA approval of epoetin-α (Epogen; Procrit).
A number of additional epoetin preparations have been developed and are available for use outside of the United States. In particular, epoetin biosimilars, which are drugs designed as copies of either epoetin-α or epoetin-β, have been available in Europe since 2007 (98). Due to the high molecular complexity of biological medications, small changes can have a significant impact on clinical outcomes. Therefore, the FDA and other international drug-related authorities have a rigorous approval process for biosimilar medications. The potential effects of changing the formulation process of epoetins was demonstrated by the global increase in cases of pure red cell aplasia (PRCA) that occurred after subcutaneous (SC) Eprex, an epoetin-α product, was changed to an albumin-free formulation (99). There are currently no biosimilar epoetins available for use in the United States, but these drugs are expected to become available within the next few years. Details of the various epoetins are discussed below (TABLE 29.2).
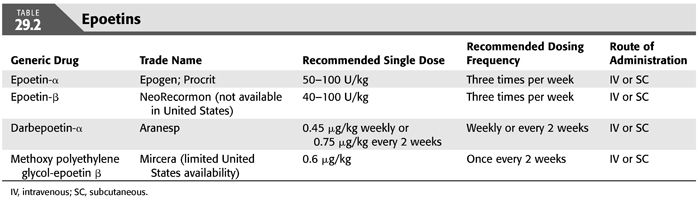
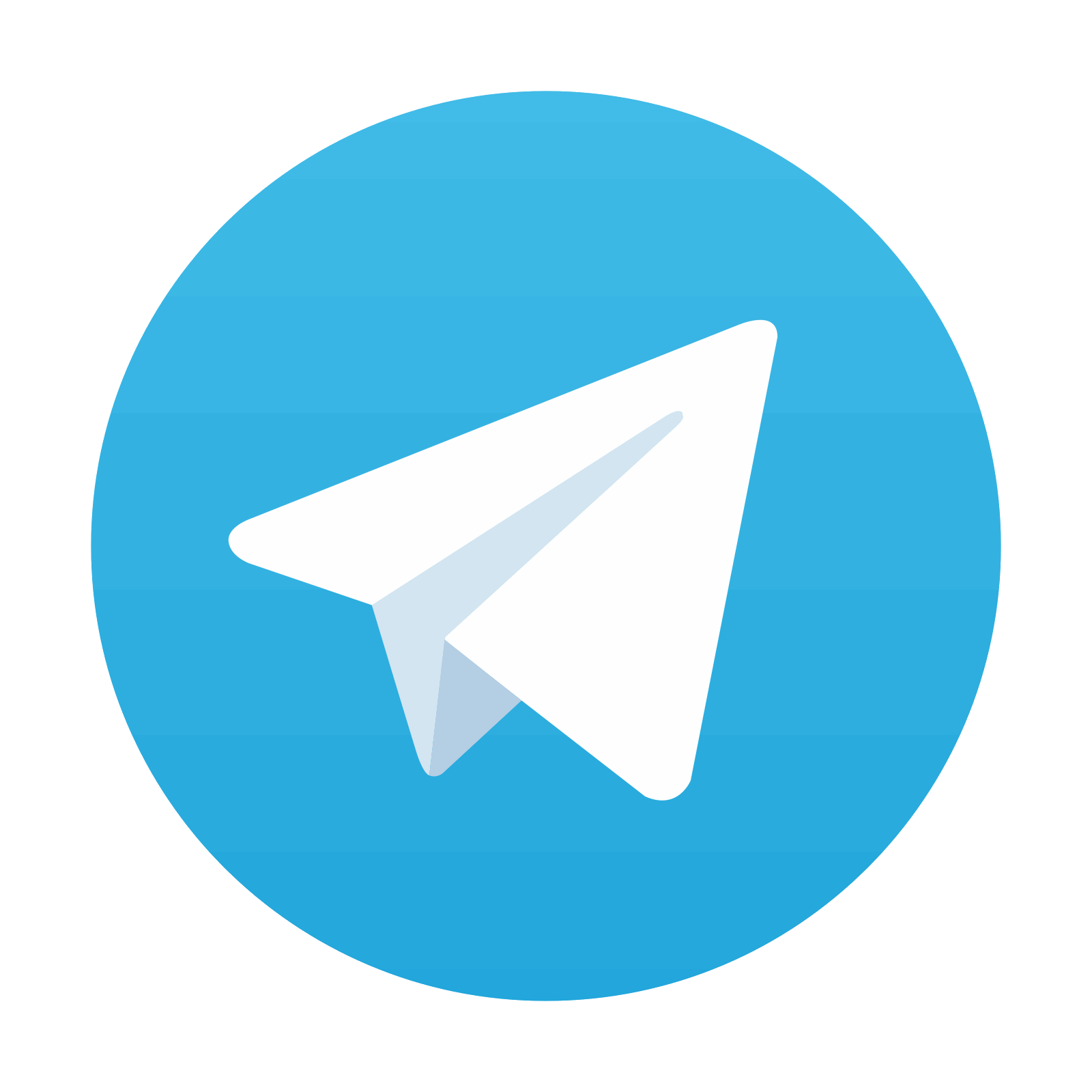
Stay updated, free articles. Join our Telegram channel

Full access? Get Clinical Tree
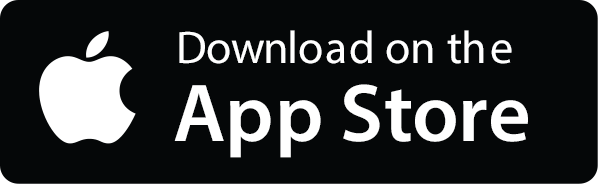
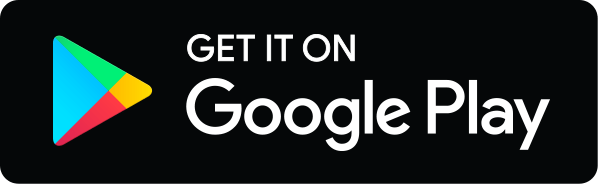