PHYSIOLOGY
The lungs and the kidneys interact closely to maintain an acid–base balance. HL Mencken, a noted American satirist, wrote in the Baltimore Sun, “Life is a battle against hydrogen ions.” Any perturbation in the hydrogen ion balance whether by addition of mineral (nonvolatile) acid such as lactic acid, formic acid, or hydrochloric/sulfuric acid; by a reduction in serum HCO3; or by an increase in carbon dioxide (volatile acid: carbonic acid) in an organism stimulates a compensatory response that attempts to revert the organism closer to a steady state. In the first two scenarios (metabolic acidosis, as defined by a reduction in serum HCO3) (1), an immediate respiratory compensation develops that can drive the PaCO2 to ≤10 mm Hg in an attempt to maintain a life-conserving pH. This extreme compensation is illustrated dramatically in presentations of diabetic ketoacidosis (DKA). An increased secretion of hydrogen ions eventually occurs in the kidneys with an attempt to further normalize pH if the metabolic acidosis persists.
In the third scenario above (respiratory acidosis, defined by an increase in PaCO2) (2), the kidneys retain HCO3 to allow for a more normal pH. Respiratory acidosis is typically seen in patients with chronic obstructive pulmonary disease (COPD) and obesity hypoventilation syndrome (OHS) in addition to respiratory failure due to neuromuscular disease (e.g., myasthenia gravis and acute inflammatory demyelinating polyneuropathy).
Conversely, the emergence of a primary metabolic alkalotic state (defined by an increase in serum HCO3) (3) produces a drop in alveolar ventilation mediated by medullary chemoreceptors to allow carbon dioxide levels (and thus hydrogen ion concentration borne of carbonic acid) to rise to allow reversion closer to steady state. Compensatory hypercapnia in this setting is usually modest (expected PaCO2 48 to 50 mm Hg with arterial pH not infrequently in excess of 7.60). Primary metabolic alkalosis is most frequently seen in the setting of vomiting and diuretic use.
A reduction in carbon dioxide tension in the organism due to primary respiratory alkalosis triggers an “alkaliuresis,” again with the goal to revert pH to a more normal state. Primary respiratory alkalosis may be seen in patients presenting with stroke, head injury, or salicylate intoxication, in addition to asthma and pulmonary embolism (PE), for example.
Respiratory responses to primary acid–base perturbations occur almost instantly (minutes), while kidney compensation is slower, taking days to reach a peak response, and therefore, the ability of an organism to respond to a major insult to the acid–base balance is limited by the intensity of response that may be required and coexistent morbidities affecting either organ system. For example, a limitation in ventilation due to airway disease may prevent an adequate response to a severe metabolic acidosis in the setting of sepsis or poisoning, similarly, presence of end-stage kidney disease (ESKD) and a concomitant new respiratory acidosis may result in a lower pH than if the kidneys were functional. Given the rapidity of respiratory perturbations in acid–base disturbances, more extreme changes in arterial pH can be expected compared to the relatively slower (and weaker) perturbations in metabolic alterations.
There are three major methods by which physiologic interactions between the lung and kidney may be described: using HCO3 to quantify the metabolic component, the strong ion difference (SID), and the standard base excess (SBE). All three are essentially clinically indistinguishable (4,5) with regard to predicting secondary directional changes in metabolic parameters.
Pulmonary kidney interactions are defined mathematically by the Henderson-Hasselbalch equation (5) encapsulating the essential and intimate relationship between the lungs and the kidney to maintain life (EQUATION 26.1):

Detailed acid–base disturbance reviews have recently been published and are out of the scope of this basic review (6).
The kidneys also play a major role in the regulation of volume status; major perturbations in kidney function will necessarily impact pulmonary function due to changes in total lung water. This is discussed further below.
Key Points
• Respiratory perturbations in acid–base disturbances result in more extreme changes in arterial pH compared to the relatively slower (and weaker) perturbations in metabolic alterations.
• There are three major methods to describe secondary directional changes in acid–base disturbances: using HCO3 to quantify the metabolic component, the strong ion difference (SID), and the standard base excess (SBE). All three are essentially clinically indistinguishable and may be used interchangeably.
CHRONIC KIDNEY DISEASE AND LUNG FUNCTION
A major consideration in patients with chronic kidney disease (CKD) is volume status; this may be especially important not only in patients in the intensive care unit (ICU) but also in the ambulatory patient assessed for dyspnea. Volume homeostasis is typically preserved until the estimated glomerular filtration rate (eGFR) falls to 10 to 15 mL/min/1.73m2. These patients may however be at risk for volume overload with small perturbations in sodium and/or water intake.
For the physician caring for a dyspneic patient with ESKD on hemodialysis (HD), it is important to assess interdialytic weight gain which may be a marker for hypervolemia (7). Pulmonary edema is a recognized complication of fluid overload in patients with CKD stage 5 whether or not dialysis is ongoing and substantial pulmonary congestion in patients with ESKD may be present without symptoms or signs or with subtle signs (8). Lung ultrasound is a useful noninvasive technique to determine lung congestion in the setting of HD.
B-lines (also called “comet tails,” “ultrasound comets,” or “lung comets”) (FIGURES 26.1–26.4) are reverberation artifacts that occur due to reflection of the ultrasound beam off thickened interlobular septae in the setting of pulmonary congestion (9). The absence of B-lines is a good predictor of a low-wedge pressure (9), and positive correlations have been found between extravascular lung water (EVLW) determined by the PiCCO system, wedge pressure, and the number of B-lines counted across the chest (“comet-score”) (10) (FIGURES 26.5 and 26.6).
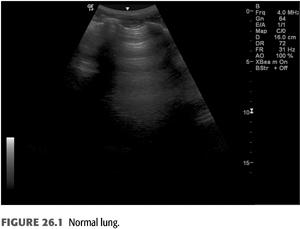
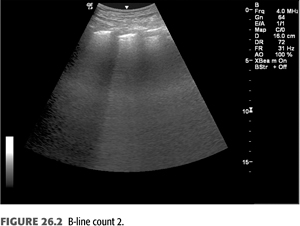
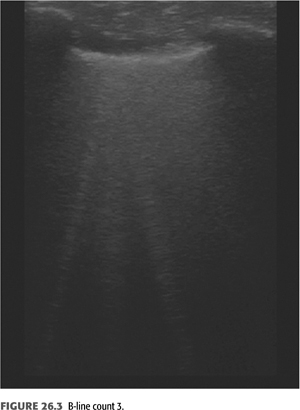
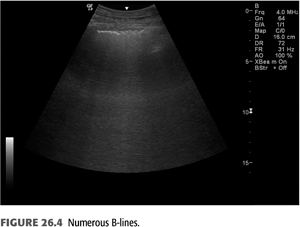
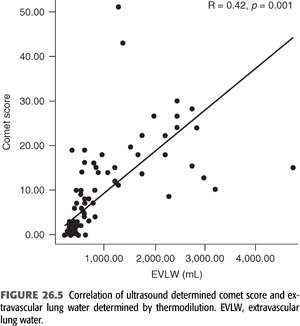
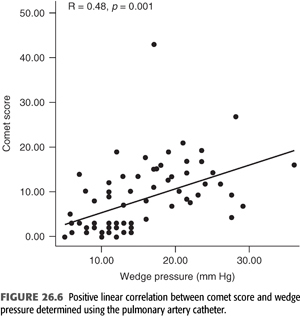
Pulmonary congestion as determined by B-line scores on lung ultrasound is highly prevalent in both symptomatic and asymptomatic patients on HD (11). These scores increase as New York Heart Association (NYHA) functional class worsens. B-line scores have been shown to decrease as fluid is removed during the course of dialysis in linear fashion and can be followed in real time (12); these scores also correlate with weight reduction during HD (13). Quantification of B-lines (a surrogate for lung congestion) has been shown to predict mortality in asymptomatic outpatients on HD (8). Lung ultrasound is easy to learn and is thus an attractive noninvasive test to assess pulmonary congestion in patients with CKD whether or not they are being dialyzed. Automated counting of B-lines is a promising technique described recently (14) that may standardize B-line scoring allowing for wider use in the setting of ESKD.
A hyperinflation pattern on pulmonary function testing (increased total lung capacity and residual volume), diminished inspiratory respiratory muscle strength (TABLE 26.1) (15), and a reduced diffusion capacity for carbon monoxide (DLCO or KCO) (TABLE 26.1 and FIGURE 26.7) (16) may be seen among patients with CKD. Other studies (17) have shown a restrictive pattern, reduced DLCO, and diminished inspiratory respiratory muscle strength in patients on HD. These abnormalities seem to remit slowly with transplantation (TABLE 26.2) (17).
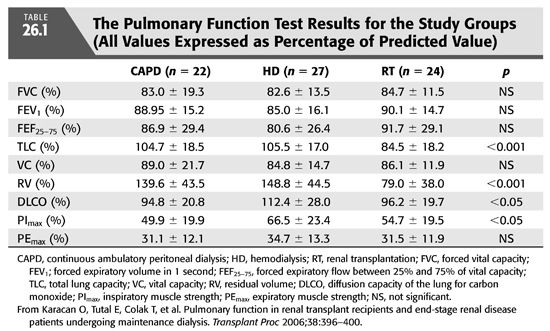
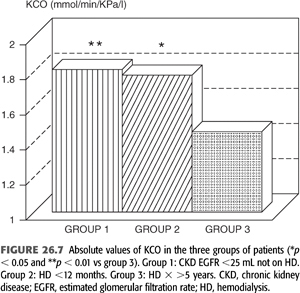
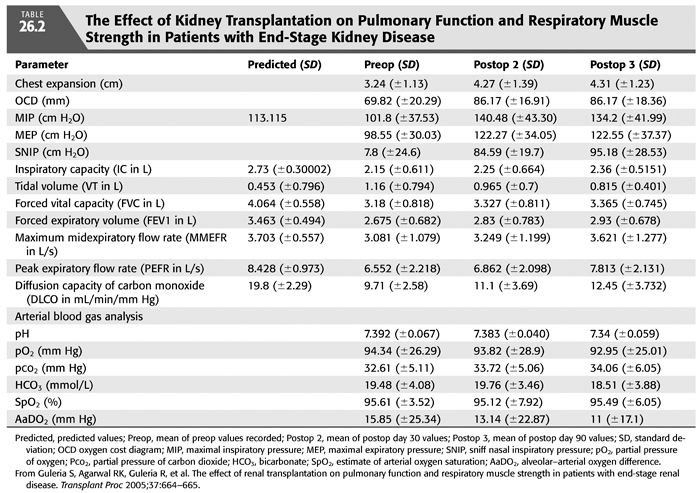
For the patient in the ICU presenting with dyspnea due to pulmonary congestion, supportive measures include noninvasive ventilation, oxygen, and fluid removal with dialysis as soon as feasible. Nondialytic measures to treat pulmonary edema in the setting of hypertensive urgencies or emergencies include intravenous nicardipine (slow onset of action and long half-life) (18), intravenous labetalol, or intravenous fenoldopam (19). Intravenous nitroglycerine may also be indicated in the right clinical setting (e.g., coronary ischemia). Studies of the effect of loop diuretics on central hemodynamics in this setting are conflicting, some showing favorable improvement (20) and others no change (21). Patient characteristics may account for some of the differences in the outcomes of these studies.
Key Points
• Interdialytic weight gain may be a marker for hypervolemia in apparently stable outpatients with ESKD.
• Assessment of B-lines using ultrasound is a useful and validated technique to assess congestion in relatively stable outpatients with ESKD. Pulmonary congestion assessed by ultrasound correlates with mortality among patients with ESKD.
• Restrictive and obstructive pulmonary function abnormalities remit with dialysis.
CHRONIC KIDNEY DISEASE AND PULMONARY HYPERTENSION
The Fifth World Symposium on Pulmonary Hypertension (PH) classifies PH-associated with ESKD as Group 5 (“of unknown or multifactorial origin”) (22). Dyspnea in a patient with CKD that remains unexplained ought to trigger a workup for PH. The diagnosis of PH requires right heart catheterization (RHC), performed after HD in the dialysis-dependent patient.
PH, defined as a mean pulmonary artery pressure ≥25 mm Hg determined by RHC (23), is common among patients with both dialysis-dependent and non–dialysis-dependent CKD stage 5. The prevalence of PH in non–dialysis-dependent CKD stage 5 ranges from 9% to 39% (24) and is higher in patients on HD (25). While connective tissue disorders and systemic illnesses may contribute, it is increasingly being recognized that abnormal kidney function per se is a trigger for the development of PH. Endothelial dysfunction is universal in patients with CKD and may contribute with other kidney-specific factors thought to be important including the presence of arteriovenous fistulas, exposure to dialysis membranes, secondary hyperparathyroidism, and anemia (24).
Most cases of PH in the setting of CKD, however, are Group 2 (secondary to left heart dysfunction) (26). It is important to ensure that reversible and treatable causes of PH are systematically investigated, the two major ones being venous thromboembolism and sleep apnea. PE is more frequent in patients with ESKD on HD than those with CKD. ESKD and CKD independently confer an increased risk of PE compared with patients with normal kidney function (27).
Sleep apnea (discussed later in this chapter) is intimately linked with volume overload both of which lead to nocturnal hypoxemia that may drive the development or progression of PH (28,29).
Assessment of pulmonary congestion with ultrasound as described previously in this chapter may assist in identifying some of these patients. The treatment of PH in the setting of CKD centers on the treatment of modifiable risk factors, aggressive control of blood pressure, and pulmonary congestion. In the absence of trials of specific vasodilator agents in this setting, a recommendation for vasodilator therapy cannot be made, although PH-specific therapies can be considered on a case-by-case basis for those with defined class I disease.
It is important to note that kidney transplantation results in substantial improvements in left ventricular (LV) dysfunction and PH (30,31). Established PH, while not a contraindication to kidney transplantation, was associated with an increased risk of mortality in kidney transplant recipients especially if it was severe [defined as pulmonary artery systolic pressure (PASP) >50 mm Hg] (32). Severe PH despite aggressive treatment is typically considered a contraindication to kidney transplantation.
Key Points
• The prevalence of PH in non–dialysis-dependent CKD stage 5 is higher than in the general population and is even higher in patients on HD.
• Most cases of PH in the setting of CKD are Group 2 (secondary to left heart dysfunction).
• PE is more frequent in patients with ESKD on HD than those with CKD.
• ESKD and CKD independently confer an increased risk of PE compared with patients with normal kidney function.
• Kidney transplantation results in substantial improvements in LV dysfunction and PH.
• Severe PH despite aggressive treatment is typically considered a contraindication to kidney transplantation.
PULMONARY KIDNEY INTERACTIONS IN SEPSIS
There is increasing appreciation for pulmonary kidney cross talk in the setting of acute kidney injury (AKI). Preexisting AKI increases the susceptibility of the lungs to an endotoxin insult (33). Additionally, in animal studies, absent obvious fluid overload, ischemic AKI has been demonstrated to increase lung water via altered Na-K aquaporin channels and pulmonary endothelial cell death (34). Increased lung endothelial cell apoptosis and increased lung inflammation as demonstrated by increased bronchoalveolar lavage fluid (BALF) protein, myeloperoxidase activity, and neutrophil cell counts has been demonstrated with primary ischemic AKI (35,36). This response is distinct from the response to experimental nephrectomy (37). AKI also induces cardiac cell apoptosis, which in turn may worsen EVLW (38
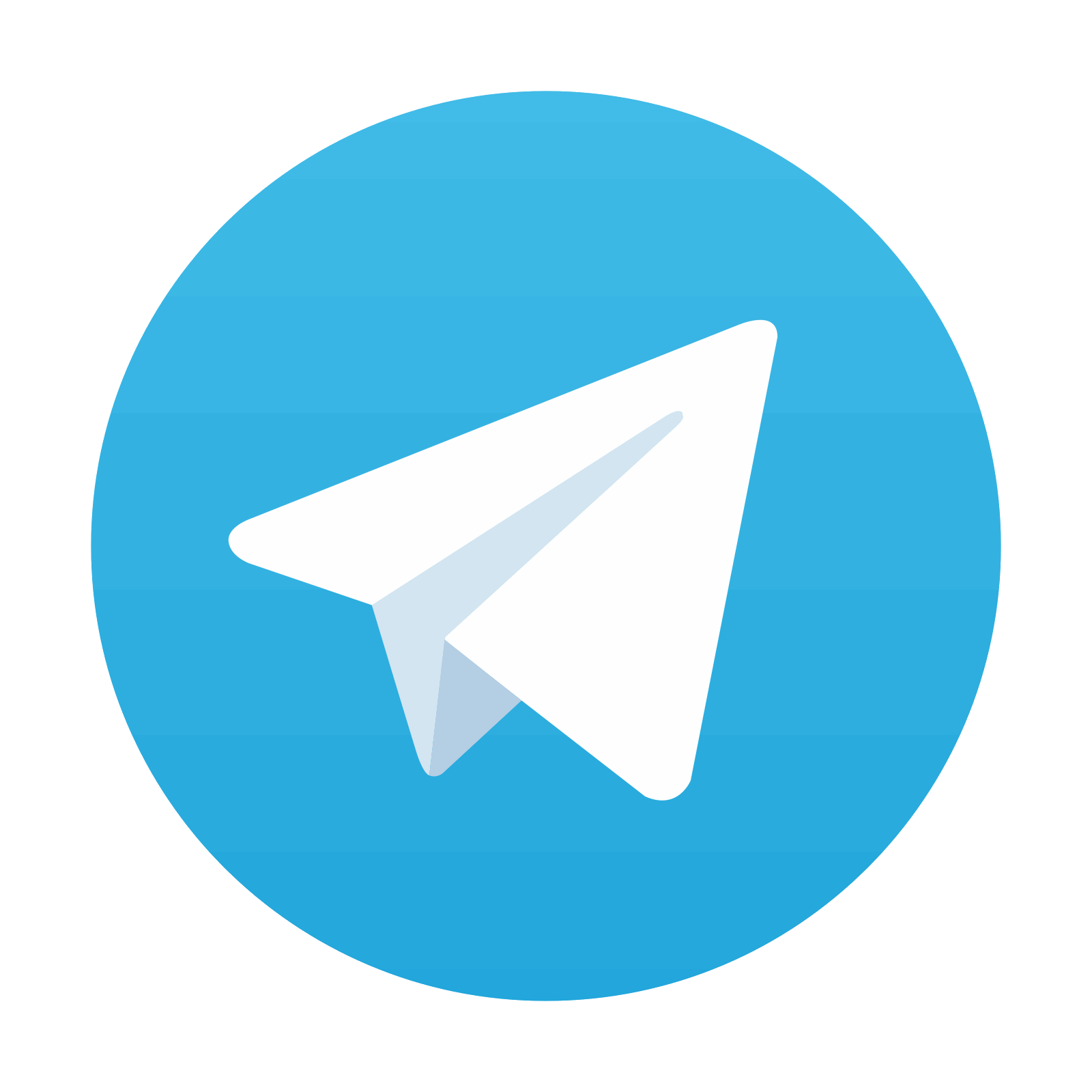
Stay updated, free articles. Join our Telegram channel

Full access? Get Clinical Tree
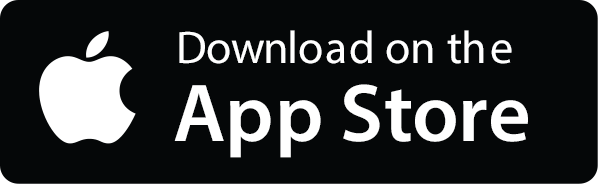
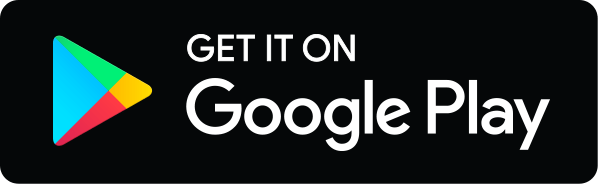