Energy targeting is greatly enhanced through imaging modalities, which greatly assist needle placement or energy delivery to the optimal location for maximal effectiveness. When vital structures obscure access to the renal lesion, laparoscopic mobilization of these structures with direct visualization of the tumor can increase the likelihood of ablation success and minimize complication risk. Ablative therapies are attractive because of their minimal impact on patient quality of life in addition to their morbidity and cost. Although they show promise of efficacy, they must be evaluated with long-term follow-up before they are considered the standard of oncologic care. Renal masses can be treated with a laparoscopic or percutaneous approach depending on tumor location, size, and the available technology and experience of the center.
Widespread use of abdominal imaging has increased the number of incidental tumors found in greater than 38,000 renal masses diagnosed in the United States in 2006 . It is estimated that as many as 51,190 cases of renal cancer were diagnosed in 2007 and that the disease would cause 12,890 deaths . By the mid-1990s, incidental lesions accounted for 60% of all renal masses . These incidental lesions present at a lower stage, grade, and likelihood of metastasis and have improved survival outcomes compared with tumors detected in symptomatic patients . The frequency of detection of small, asymptomatic, solid renal masses with low biologic activity continues to increase . The management of these tumors over the past decade has shifted from radical nephrectomy to NSS. The potential “overtreatment” of incidental small renal lesions with radical nephrectomy, along with a desire to reduce patient morbidity and preserve renal function, led to the development of nephron-sparing techniques and minimally invasive methods to manage renal tumors. Nephron-sparing surgery (NSS) was initially indicated for patients who have localized renal cell carcinoma (RCC) combined with a compromised contralateral kidney and a need to preserve overall renal function but now has become applicable in patients who have single, unilateral, localized RCC with a normal contralateral renal unit .
Partial nephrectomy has proved to be effective for patients who have small single renal tumors that do not involve the collecting system. It is also associated with significant morbidity, however, including longer operative time, increased blood loss, and prolonged hospital stay compared with radical nephrectomy . Minimally invasive approaches, such a laparoscopic partial nephrectomy, are rapidly gaining acceptance for treatment of small localized renal tumors; however, laparoscopic partial nephrectomy is associated with increased complications compared with open partial nephrectomy . Renal ablative techniques were developed to offer widespread application, improved patient procedural morbidity, and reduced potential for complications. A large variety of generators, ablation probes, and energy delivery systems are now commercially available. In addition to their efficacy and safety, oncologic outcomes have been excellent to date, which supports the rationale of in situ tumor destruction to reduce morbidity and invasiveness further . In situ thermal destruction of RCC uses techniques that destroy tumor tissue through freezing (cryotherapy) or heating (radiofrequency ablation [RFA], microwave ablation, laser interstitial therapy, high-intensity focused ultrasound [HiFU]), and radiosurgery). Each of these techniques relies on controlled energy delivery in an attempt to minimize collateral damage to normal renal parenchyma and other surrounding structures.
Cryotherapy
The term cryotherapy refers to the therapeutic use of cold. Cryosurgery is the use of cold as a means of tissue destruction. The Egyptians began using cold to treat injuries and inflammation around 2500 bc . The first application of cryotherapy to treat malignancy was described in the mid-nineteenth century by Arnott , who used a salt solution containing crushed ice at −18°C to −24°C to shrink breast, cervical, and skin cancers. Technologic advances leading to the invention of an automated cryosurgical apparatus in the early 1960s and vacuum-insulated liquid nitrogen or argon-cooled probes led to a renewed interest in cryosurgery and its possible application in renal cancer .
Animal models of renal cryoablation were first published in 1974 . Human literature was not reported until more than 2 decades later when Uchida and colleagues first published their reports of percutaneous cryoablation of renal lesions. Soon after, reports on open and laparoscopic approaches appeared in 1996 and 1998 , respectively. Many of these early studies demonstrated that renal cryoablation was technically feasible, safe, and initially efficacious. As new 5-year postoperative data emerge, cryoablation is finally gaining acceptance as a standard option for the treatment of patients who have localized renal masses less than 4 cm.
Principles of cryotherapy (freezing)
The key factors involved in freezing injury include direct mechanical shock, osmotic shock, and cellular hypoxia. Cryotherapy-induced necrosis is essentially a three-step process. Initially, extracellular ice formation increases the osmolarity in the extracellular space, leading to a shift of fluid from the intracellular to extracellular space. As a result, changes in pH lead to protein denaturation and accumulation of toxins within the cell. Next, intracellular ice forms with lower temperatures, and disruption of cellular membranes occurs. Finally, delayed microcirculatory failure occurs with the thaw phase of the freeze-thaw cycle, resulting in further cell disruption, endothelial damage, microvascular thrombosis, and eventual tissue ischemia. This last process may take days to weeks and is ultimately responsible for the coagulative necrosis, fibrosis, and collagen deposition seen by 1 month .
The lethal temperature for achieving reliable deaths of normal and cancerous renal cells is near −20°C. Investigators have shown that exposure of renal cancer cell lines to −10°C for 60 minutes resulted in cell death in only 5% of cells. Exposure to −20°C, however, resulted in 85% cell death . Despite the low temperatures created near the tip of the cryoprobe, or center of the ice ball, there is rapid warming of the tissue toward the periphery of the ice ball ( Fig. 1 ). Campbell and colleagues showed in a canine model that the edge of the ice ball had to extend at least 3.1 mm beyond the edge of the target lesion for adequate cell death to occur. We routinely extend the ice ball at least 10 mm beyond the edge of the tumor to ensure an adequate margin of tissue death. In addition to the temperature that should be achieved, the duration of freezing has been demonstrated to be an important factor leading to tissue destruction. Once the tissue temperature reaches a certain level, more cellular destruction can be achieved by prolonged freezing at this temperature . Not all structures are equally cryosensitive. The renal collecting system has been shown to remain intact after ice ball involvement if the tissue has not been lacerated mechanically .
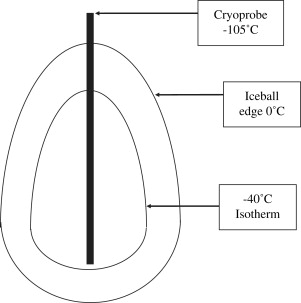
As outlined, thawing of the cryoablated tissue is as instrumental as the freezing process to ensure tumor destruction. It has been reported that repeating the freeze-thaw cycle exacerbates tissue damage and increases liquefaction necrosis . The authors currently use two freeze-thaw cycles. Optimal freeze times have also been studied in swine. Five-minute freeze times were shown to be inadequate to cause tissue necrosis and were associated with excessive bleeding, whereas 15-minute freeze times produced consistent necrosis but were associated with renal fracture. It was concluded that the 10-minute freeze time seems to be optimal to produce necrosis without complications . Still debated, however, is whether a slow passive thaw is more effective than a rapid active thaw. Finally, clamping the renal artery before cryotherapy to avoid the heat sink effect of renal blood flow has been shown to increase the size of the cryolesion only marginally .
Principles of cryotherapy (freezing)
The key factors involved in freezing injury include direct mechanical shock, osmotic shock, and cellular hypoxia. Cryotherapy-induced necrosis is essentially a three-step process. Initially, extracellular ice formation increases the osmolarity in the extracellular space, leading to a shift of fluid from the intracellular to extracellular space. As a result, changes in pH lead to protein denaturation and accumulation of toxins within the cell. Next, intracellular ice forms with lower temperatures, and disruption of cellular membranes occurs. Finally, delayed microcirculatory failure occurs with the thaw phase of the freeze-thaw cycle, resulting in further cell disruption, endothelial damage, microvascular thrombosis, and eventual tissue ischemia. This last process may take days to weeks and is ultimately responsible for the coagulative necrosis, fibrosis, and collagen deposition seen by 1 month .
The lethal temperature for achieving reliable deaths of normal and cancerous renal cells is near −20°C. Investigators have shown that exposure of renal cancer cell lines to −10°C for 60 minutes resulted in cell death in only 5% of cells. Exposure to −20°C, however, resulted in 85% cell death . Despite the low temperatures created near the tip of the cryoprobe, or center of the ice ball, there is rapid warming of the tissue toward the periphery of the ice ball ( Fig. 1 ). Campbell and colleagues showed in a canine model that the edge of the ice ball had to extend at least 3.1 mm beyond the edge of the target lesion for adequate cell death to occur. We routinely extend the ice ball at least 10 mm beyond the edge of the tumor to ensure an adequate margin of tissue death. In addition to the temperature that should be achieved, the duration of freezing has been demonstrated to be an important factor leading to tissue destruction. Once the tissue temperature reaches a certain level, more cellular destruction can be achieved by prolonged freezing at this temperature . Not all structures are equally cryosensitive. The renal collecting system has been shown to remain intact after ice ball involvement if the tissue has not been lacerated mechanically .
As outlined, thawing of the cryoablated tissue is as instrumental as the freezing process to ensure tumor destruction. It has been reported that repeating the freeze-thaw cycle exacerbates tissue damage and increases liquefaction necrosis . The authors currently use two freeze-thaw cycles. Optimal freeze times have also been studied in swine. Five-minute freeze times were shown to be inadequate to cause tissue necrosis and were associated with excessive bleeding, whereas 15-minute freeze times produced consistent necrosis but were associated with renal fracture. It was concluded that the 10-minute freeze time seems to be optimal to produce necrosis without complications . Still debated, however, is whether a slow passive thaw is more effective than a rapid active thaw. Finally, clamping the renal artery before cryotherapy to avoid the heat sink effect of renal blood flow has been shown to increase the size of the cryolesion only marginally .
Cryoprobes and cryogens
The goal of cryotherapy is to freeze a specified volume of tissue resulting in necrosis without significant damage to the surrounding healthy tissue. With this in mind, cryoprobes have been developed with characteristics, such as variable diameter and length of the freeze zone, to produce a specific size of cryolesion. In addition, the velocity of the temperature change and the nadir probe temperature affect the dimensions and temperature gradient of the ice ball. Finally, thermal conductivity of the target tissue affects the volume of tissue that is ablated. For example, a 3.4-mm probe cooling at the rate of 50°C per minute to a nadir probe tip temperature of −175°C creates a cryolesion 4 cm in diameter in 20 minutes. In comparison, an 8-mm probe cooling at the rate of 100°C per minute to a nadir tip temperature of −190°C results in a cryolesion 7 cm in diameter in 20 minutes . Knowing this, a cryoprobe may be chosen on the basis of the size of the lesion to be ablated. Alternatively, a single probe may be placed repeatedly to achieve a larger cryolesion in large or irregular tumors.
Various clinically relevant cryogens are available, with the boiling point of each determining the nadir temperature that the specific cryoprobe can produce. Liquid argon and liquid nitrogen, the two most commonly used cryogens, have boiling points of −186°C and −196°C, respectively. Liquid nitrogen–based systems circulate the compressed nitrogen within the cryoprobe, allowing it to boil at the tip, thereby extracting the latent heat of boiling from its surrounding environment. Liquid argon–based systems rely on the Joule-Thompson effect, in which compressed gas or liquid under high pressure is allowed to expand rapidly through a narrow orifice in the tip cavity of the cryoprobe. The rapid cooling that results creates an ice ball .
Surgical technique
Cryotherapy can be delivered by means of an open, laparoscopic, or percutaneous surgical approach. Open cryotherapy requires an incision large enough for kidney mobilization and tumor exposure. This approach is typically entertained when a concomitant open abdominal procedure is being performed. Although invasive, the open approach allows for excellent sonographic monitoring with ultrasound.
Laparoscopy has been the preferred method for renal cryoablation, especially for anterior and anteromedial tumors. The transperitoneal approach offers the advantages of familiarity to most urologic surgeons and a distensible cavity to provide a large working space. Retroperitoneoscopy offers the advantages of working away from other organs and limitation of hematoma spread with the protection of the perirenal fat and Gerota’s fascia. Advantages of laparoscopy include careful visual monitoring of probe placement and ice ball progression in relation to the mass under laparoscopic ultrasound guidance; mobilization of vital structures away from the treatment area, including bowel, liver, and spleen; and easy establishment of hemostasis.
The percutaneous approach can also be used successfully under cross-sectional imaging or ultrasound guidance. The advantages of a percutaneous approach over the laparoscopic approach include less invasiveness, shorter hospitalization, excellent ice ball monitoring with cross-sectional imaging, less pain medication requirement, decreased operating time, and cost-effectiveness. Disadvantages include the lack of visual cues for probe placement, inability to separate vital structures from the treatment area, and radiation exposure during CT treatment.
Regardless of the approach used, the fundamentals are the same. The procedure involves real-time imaging of the tumor, needle biopsy of the tumor, insertion of the cryoprobe perpendicular to the tumor, placement of the distal tip of the probe at the deep margin of the tumor, ensuring that the probe is not in contact with surrounding viscera, creation of an ice ball extending approximately 10 mm beyond the tumor, and achievement of adequate hemostasis after removal of the cryoprobe.
In most series, the investigators follow the ablated lesion using contrast-enhanced CT or MRI every 3 to 6 months initially. Lack of enhancement on CT or MRI, with stable or decreased tumor size, can be considered a sign of successful cryoablation ( Fig. 2 ). Signs of recurrent cancer include increased tumor size, lack of tumor shrinkage, and nodular or lesion enhancement greater than 10 Hounsfield units (HU).
At the University of Wisconsin, select patients are offered cryoablation or laparoscopic partial nephrectomy for most localized renal tumors 4 cm or less in size. Laparoscopy is the preferred technique for anterior and lateral tumors, and percutaneous cryoablation is preferred for posterior tumors with a clear window for probe placement. Cryotherapy is administered under general anesthesia, although some centers use local sedation, and is performed using an argon gas–based system that operates on the Joule-Thompson principle. Cryoprobes are available in diameters of 1.4 mm, 2.4 mm, 3.4 mm, and 3.8 mm. The number and size of cryoprobes used in individual cases vary because of differences in tumor size and location. Most probes can typically be passed percutaneously or laparoscopically, because variable lengths are offered with each probe size. For the laparoscopic approach, we now leave Gerota’s fascia intact to tamponade the bleeding from the cryoablated site and minimize tumor spillage. Laparoscopic ultrasound is used to confirm tumor and proper probe placement, and cryotherapy is initiated using two 10-minute freeze cycles ( Fig. 3 ). The freeze cycles are monitored so that the ice ball extends 1 cm beyond the tumor margin. The cryolesion is monitored with real-time sonography performed by a radiologist experienced in laparoscopic ultrasound, which we believe better confirms adequate treatment of the margin. A passive thaw is allowed between the freezing cycles, and an active thaw is allowed after the second freeze. The authors allow the probe to loosen spontaneously before removing it during the active thaw. Hemostatic matrix is then injected into the cryoprobe defect ( Fig. 4 ) and covered with absorbable hemostat. Direct pressure is applied for 10 minutes. With the insufflation pressure decreased, the cryolesion is observed for 15 minutes to confirm hemostasis. For the percutaneous approach, the cryoprobes are placed under ultrasound or CT guidance with the patient in the prone position. After the cryoprobe is removed, images are routinely obtained to rule out postoperative hemorrhage.
All patients are admitted overnight after surgery for observation. Serum creatinine and hematocrit are measured on the day after surgery, and patients are discharged home once they tolerate diet with their pain controlled on oral analgesics. The ablated lesions are followed using contrast-enhanced CT or MRI every 3 months during the first year, every six months during the second year, and then yearly. If a lesion does not shrink appropriately, more diligent follow-up is required.
Outcomes
Three clinical series have recently matured to provide us with 5-year follow up data for renal cryoablation. Hegarty and colleagues reported on 194 patients undergoing laparoscopic renal cryoablation since 1997, with 66 patients having more than 5 years of follow up with MRI surveillance. Of the 66 patients with long-term follow-up, the indication for treatment was a solitary sporadic renal lesion in 48 (73%). Mean patient age was 66 years. Mean tumor size was 2.3 cm (range 1–4.5 cm). Three patients (6%) developed tumor recurrence requiring nephrectomy. One patient was diagnosed with metastatic RCC shortly after treatment and died 19 months later. The authors report an overall 5-year survival of 81% and a 5-year cancer-specific survival of 98% .
The second series with a 5-year follow-up included 48 patients, all with more than a 3-year follow-up (median of 64 months). Twenty-four patients were treated with the open technique, whereas the other 24 were treated with the laparoscopic technique. The median lesion size was 2.6 cm (range: 1.1–4.6 cm). The overall survival rate was 89.5%. Five patients (12.5%) were diagnosed with persistent disease and treated with repeat renal cryoablation (n = 1), laparoscopic nephrectomy (n = 2), laparoscopic partial nephrectomy (n = 1), and observation (n = 1). The cancer-specific survival rate was 100%, and the cancer-free survival rate after a single cryoablation procedure was 87.5%. This improved to 97.5% after repeat treatment. No complications were observed .
Bandi and colleagues recently reported the first series with 5-year data using percutaneous cryoablation. A total of 78 patients had 88 small renal masses treated by laparoscopic cryoablation (n = 58) and percutaneous cryoablation (n = 20). Mean tumor size was 2.6 cm. Mean follow-up was 19 months. The overall, cancer-specific, and recurrence-free survival rates were 88.5%, 100%, and 98.7%, respectively. Four patients required a repeat treatment because of persistent disease, and 1 had progression to locally advanced disease. Five patients had complications during cryoablation, and 7 had complications afterward .
Table 1 summarizes the largest cryotherapy trials (>20 patients) to date. These studies suggest that cryotherapy is a safe minimally invasive treatment option for patients who have small renal masses at the intermediate follow-up.
Study (year) | Approach | Patients | Tumor size (cm) | Follow-up (months) | OR time (minutes) | EBL (mL) | Complications | Hospital stay (days) | Recurrence |
---|---|---|---|---|---|---|---|---|---|
Shingleton and Sewell (2001) | Perc | 20 | 3.2 | 9.1 | 97 | NA | 1 | 1 | 0 |
Cestari et al (2004) | Lap | 37 | 2.6 | 20.5 | 194 | 165 | 7 | 3.8 | 1 |
Gill et al (2005) | Lap | 56 | 2.3 | NA (all >3 years) | 180 | 87 | 4 | NA | 2 |
Hegarty et al (2006) | Lap | 66 | 2.3 | NA | NA | NA | NA | NA | 3 |
Schwartz (2006) | Open/Lap | 85 | 2.6 | 10 | NA | 58 | 3 | 3 | 2 |
Davol et al (2006) | Open/Lap | 48 | 2.6 | 64 | NA | NA | 7 | 3 | 5 |
Polascik et al (2007) | Lap | 26 | 2.5 | 7 | NA | NA | 2 | 2 | 0 |
Tillet et al (2006) | Lap | 41 | 3.1 | 12.5 | NA | NA | 3 | NA | 3 |
Beemster and De la Rosette (2006) | Lap | 31 | 2.4 | NA | 204 | NA | 3 | 5.1 | 1 |
Bandi et al (2007) | Lap/Perc | 78 | 2.6 | 19 | 220 | NA | 11 | 2.1 | 4 |
Complications
The complications of renal cryotherapy are rare and have rates similar to those of other laparoscopic and percutaneous procedures. Complications stem from the technical approach and from the ablation. Pain or paresthesia at the probe site seems to be the most common complication, occurring in approximately 5% of all patients . Because the entire probe is cooled in cryotherapy, care must be taken to avoid injury to the sensory nerves at the body surface. Other complications include hemorrhage, urinary infection, pneumonia, renal insufficiency, wound infection, ileus, adjacent organ injury, and respiratory difficulty. The complications of the larger cryotherapy series are listed in Table 2 .
Study (year) | Major complications | Minor complications |
---|---|---|
Shingleton and Sewell (2001) | None | Wound infection |
Cestari et al (2004) | UPJO |
|
Schwartz (2006) |
| Hydronephrosis (resolved) |
Gill et al (2005) |
|
|
Davol et al (2006) | None |
|
Polascik et al (2007) | None |
|
Tillet et al (2006) | None |
|
Beemster and De la Rosette (2006) | None |
|
Bandi et al (2007) |
|
|
In a multi-institutional study evaluating complications after 139 cryoablation procedures, with 2 major and 18 minor complications, was reported. Overall, 4 complications were reported in the laparoscopic group and 16 were reported in the percutaneous group. Of these 20 complications, only 2 were reported as major complications and no deaths were reported. The investigators also reported that all major complications occurred early in the series, suggesting that experience with laparoscopy and percutaneous procedures is important for preventing and managing these complications .
Radiofrequency ablation
Indications for radiofrequency ablation treatment
RFA is a heat-based method of tissue destruction originally developed for the treatment of aberrant cardiac pathways. The first oncologic application involved the treatment of primary and metastatic liver tumors . Current applications include the treatment of breast malignancy, aberrant cardiac pathways, gynecologic tumors, prostate disease, bone lesions, pancreatic cancer, renal tumors , and metastatic lesions.
With reports of RFA success in renal tumors ranging from small incidental lesions to metastatic deposits, how do we determine who is appropriate for renal RFA treatment? Prospective randomized clinical series to identify patients appropriate for RFA therapy have not been performed to date. Phase II trials have included patients receiving RFA simultaneously with open or laparoscopic nephrectomy before nephrectomy, in patients deemed inappropriate for extirpative surgery because of comorbidities, and in patients who had hereditary RCC. Most clinicians agree that patients with a solitary kidney, multiple synchronous RCC, von Hippel-Lindau disease, familial RCC, or limited renal function are appropriate candidates for RFA treatment, but controversy exists in younger patients without significant comorbid conditions, normal contralateral renal function, and minimal future risk for renal function loss. As experience with RFA accumulates and intermediate and long-term oncologic results become available, favorable outcomes may justify the treatment of smaller and potentially less aggressive tumors in younger and healthier patients with RFA.
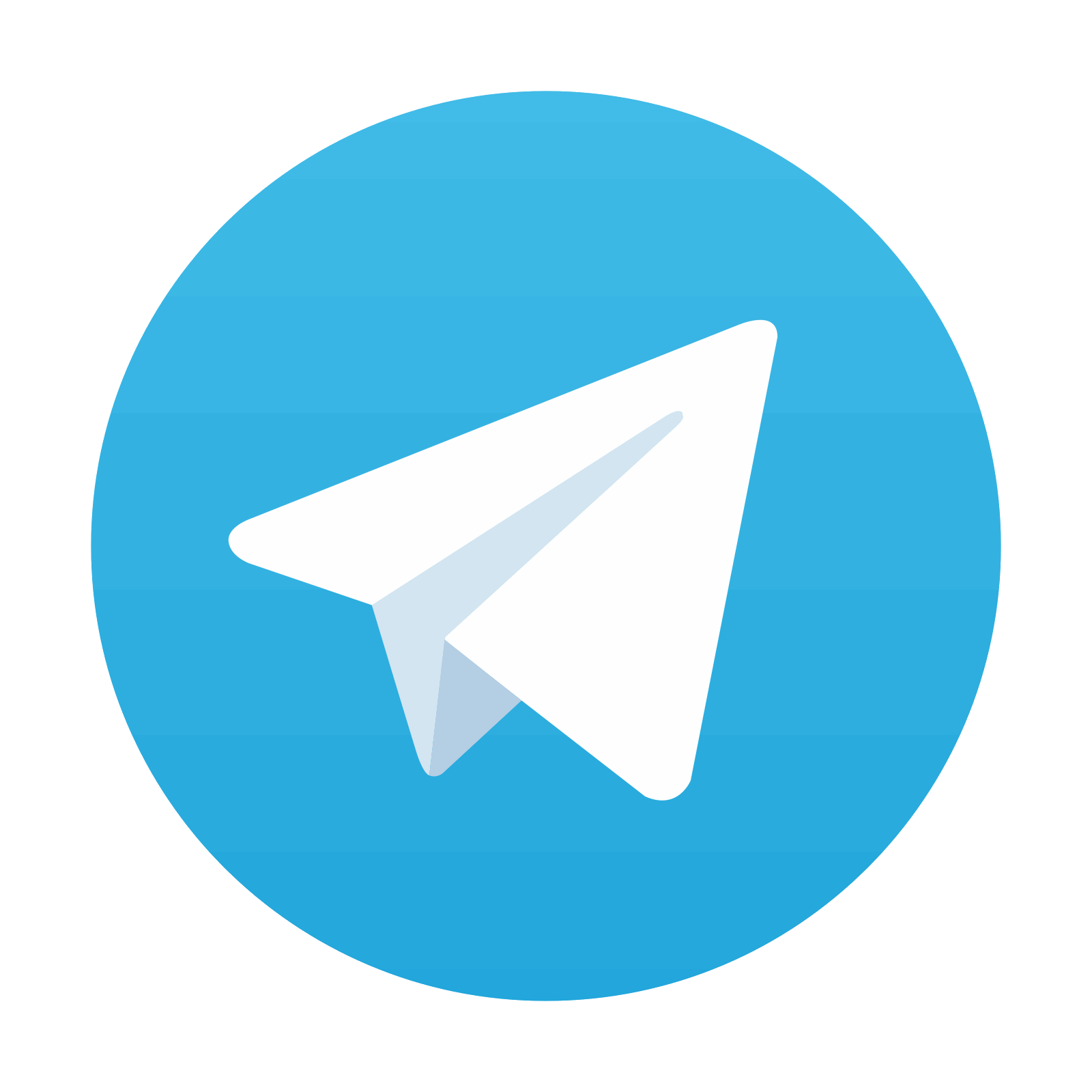
Stay updated, free articles. Join our Telegram channel

Full access? Get Clinical Tree
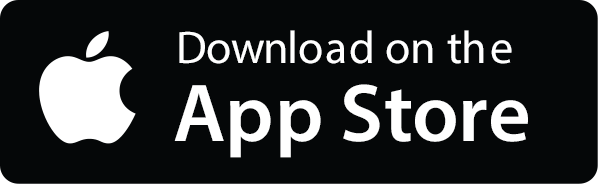
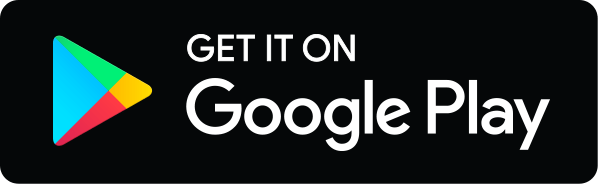