Tissue engineering efforts are currently being undertaken for every type of tissue and organ within the urinary system. Most of the effort expended to engineer genitourinary tissues has occurred within the past decade. Tissue engineering techniques require a cell culture facility designed for human application. Personnel who have mastered the techniques of cell harvest, culture, and expansion in addition to polymer design are essential for the successful application of this technology. Before these engineering techniques can be applied to humans, further studies need to be performed in many of the tissues described. Recent progress suggests that engineered urologic tissues and cell therapy may have clinical applicability.
Congenital disorders, cancer, trauma, infection, inflammation, iatrogenic injuries, or other conditions of the genitourinary system can lead to organ damage or complete loss of function. Both situations usually necessitate eventual reconstruction or replacement of the damaged organs. Currently, reconstruction may be performed with native nonurologic tissues (skin, gastrointestinal segments, or mucosa from multiple body sites), homologous tissues from a donor (cadaver fascia or cadaver or living donor kidney), heterologous tissues or substances (bovine collagen), or artificial materials (silicone, polyurethane, or polytetrafluoroethylene). All these materials often lead to various complications after reconstruction, however, because the implanted tissue is rejected or because inherently different functional parameters cause a mismatch in the system. For example, replacement of bladder tissue with gastrointestinal segments can be problematic because of the opposite ways in which these two tissues handle solutes—urologic tissue normally excretes material, and gastrointestinal tissue generally absorbs the same materials. This mismatched state can lead to metabolic complications in addition to infection and other issues. Therefore, the replacement of lost or deficient urologic tissues with functionally equivalent ones would certainly improve the outcome of reconstructive surgery in the genitourinary system. This goal may soon be attainable with the use of tissue engineering techniques.
Tissue engineering strategies for urogenital repair
Tissue engineering uses the principles of cell transplantation, materials science, and biomedical engineering to develop biologic substitutes that can restore and maintain normal function of damaged or lost tissues and organs. Tissue engineering may involve injection of functional cells into a nonfunctional site to stimulate regeneration. It can also involve the use of natural or synthetic matrices, often termed scaffolds , which encourage the body’s natural ability to repair itself and assist in determination of the orientation and direction of new tissue growth. Often, tissue engineering uses a combination of these techniques. For example, matrices seeded with cells can be implanted into the body to encourage the growth or regeneration of functional tissue.
Use of Cells in Urogenital Tissue Engineering Applications
Often, when cells are used for tissue engineering, donor tissue is dissociated into individual cells, which are then implanted directly into the host or expanded in culture, attached to a support matrix, and reimplanted after expansion. The implanted tissue can be heterologous, allogeneic, or autologous. Ideally, this approach allows lost tissue function to be restored or replaced in toto and with limited complications.
An area of concern has been the source of cells for regeneration, however. The concept of creating engineered tissue constructs involves obtaining cells from the organ to be replaced and expanding these cells in vitro so that sufficient quantities are available for the implantation technique chosen. A major concern has been that, in certain cases, there may not be enough normal cells present in the diseased organ to begin this expansion process. This may not be the case; for example, one study has shown that cultured neuropathic bladder smooth muscle cells possess and maintain different characteristics than normal smooth muscle cells in vitro, as demonstrated by growth assays, contractility, and adherence tests in vitro. Despite these differences, when neuropathic smooth muscle cells were cultured in vitro and then seeded onto matrices and implanted in vivo, the tissue-engineered constructs showed the same properties as the constructs engineered with normal cells. It is known that genetically normal progenitor cells, which are the reservoirs for new cell formation and are present even in diseased tissue, are programmed to give rise to normal tissue, regardless of whether they reside in normal or diseased tissues. Therefore, the stem cell niche, and its role in normal tissue regeneration, remains a fertile area of ongoing investigation.
Stem Cells
Most current strategies for tissue engineering depend on a sample of autologous cells from the diseased organ of the host. For many patients with extensive end-stage organ failure, however, a tissue biopsy may not yield enough normal cells for expansion and transplantation. In other instances, primary autologous human cells cannot be expanded from a particular organ, such as the pancreas. In these situations, pluripotent human embryonic stem cells are envisioned as an ideal source of cells because they can differentiate into nearly any replacement tissue in the body.
Embryonic stem cells exhibit two remarkable properties: the ability to proliferate in an undifferentiated but still pluripotent state (self-renewal) and the ability to differentiate into a large number of specialized cell types. They can be isolated from the inner cell mass of the embryo during the blastocyst stage, which occurs 5 days after fertilization. These cells have been maintained in the undifferentiated state for at least 80 passages when grown using current published protocols. In addition, many protocols for differentiation into specific cell types in culture have been published. Many uses of these cells are currently banned in the United States, however, because of the ethical dilemmas that are associated with the manipulation of embryos in culture.
Adult stem cells have the advantage of avoiding some of the ethical issues associated with embryonic cells, and, unlike embryonic cells, they do not transdifferentiate into a malignant phenotype; thus, there is a diminished risk for teratoma formation should the cells be implanted in vivo. Adult stem cells are limited for clinical use, however, because expansion to the large quantities needed for tissue engineering is difficult.
Fetal stem cells derived from amniotic fluid and placenta have recently been described and represent a novel source of stem cells. The cells express markers consistent with human embryonic stem cells, such as OCT4 and SSEA-4, but they do not form teratomas. The cells are multipotent and are able to differentiate into cells from all three germ layers. In addition, the cells have high replicative potential and could be stored for future self-use, without the risks for rejection and without ethical concerns.
Therapeutic Cloning
Nuclear cloning, which has also been called nuclear transplantation and nuclear transfer, involves the introduction of a nucleus from a donor cell into an enucleated oocyte to create an embryo with a genetic makeup identical to that of the donor. Two types of nuclear cloning, reproductive cloning and therapeutic cloning, have been described. Banned in most countries for human applications, reproductive cloning is used to generate an embryo that has the identical genetic material as its cell source to produce offspring that are genetically identical to the donor. Conversely, therapeutic cloning is used to generate early-stage embryos that are used in vitro. They are explanted in culture to produce embryonic stem cell lines that are genetically identical to the source. These autologous stem cells have the potential to become almost any type of cell in the adult body, and thus would be useful in tissue and organ replacement applications ( Fig. 1 ). A particularly useful application for these cells would be in the treatment of end-stage kidney disease, for which there is limited availability of immunocompatible tissue transplants.
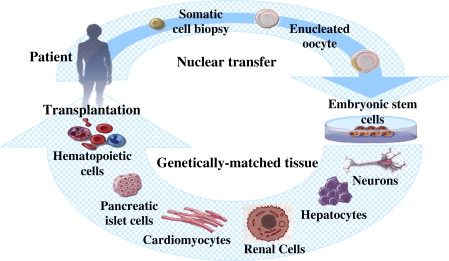
Biomaterials for Genitourinary Tissue Construction
Biomaterials in genitourinary tissue engineering function as an artificial extracellular matrix (ECM) and are used to replace biologic and mechanical functions of native ECM found in tissues in the body. Biomaterials facilitate the localization and delivery of cells or bioactive factors (eg, cell adhesion peptides, growth factors) to desired sites in the body and define a three-dimensional space for the formation of new tissues with appropriate structure. They also serve as a guide for the development of new tissues with appropriate function. Direct injection of cell suspensions without such matrices has been used in some cases; however, without this scaffold function, it is difficult to control the localization of transplanted cells.
The ideal biomaterial should be biocompatible, promote cellular interaction and tissue development, and possess the proper mechanical and physical properties found in the tissue to be generated. Generally, three classes of biomaterials have been used for the engineering of genitourinary tissues: naturally derived materials, such as collagen and alginate; acellular tissue matrices, such as bladder submucosa and small-intestinal submucosa (SIS); and synthetic polymers, such as polyglycolic acid (PGA), polylactic acid, and poly(lactic-co-glycolic acid). Although synthetic polymers can be produced reproducibly on a large scale with controlled properties of strength, degradation rate, and microstructure, naturally derived materials and acellular tissue matrices have the potential advantage of biologic recognition, which can lessen host-versus-graft reactions.
Vascularization of Engineered Tissue
Limitations of nutrient and gas exchange currently restrict tissue-engineered implants to a volume of approximately 3 mm 3 . Therefore, to achieve the goals of engineering large complex tissues and organs, vascularization of the regenerating cells is essential.
Three approaches have been used to encourage the vascularization of bioengineered tissue. First, incorporation of angiogenic factors in the bioengineered tissue has been used to attract host capillaries and to enhance neovascularization of the implanted tissue. Second, some studies have investigated the effects of seeding endothelial cells with other cell types in the bioengineered tissue. Finally, prevascularization of the matrix before cell seeding has been attempted. There are many obstacles to overcome before large tissue-engineered solid organs are produced, but recent developments in angiogenesis research may provide important knowledge and essential materials to accomplish this goal.
Tissue engineering of specific urologic and genital structures
Urethra
Various strategies have been proposed over the years for the regeneration of urethral tissue. Woven meshes of PGA have been used to reconstruct urethras in dogs. Also, PGA has been used as a cell transplantation vehicle to engineer tubular urothelium in vivo. SIS without cells was used as an onlay patch graft for urethroplasty in rabbits. Finally, a homologous graft of acellular urethral matrix was also used in a rabbit model.
Bladder-derived acellular collagen matrix has proved to be a suitable graft for repair of urethral defects in rabbits. In the rabbit model, the neourethras created with these matrices demonstrated a normal urothelial luminal lining and organized muscle bundles shortly after repair. These results were confirmed clinically in a series of patients with a history of failed hypospadias reconstruction, wherein the urethral defects were repaired with human bladder acellular collagen matrices ( Fig. 2 ). One of the advantages of this material over nongenital tissue grafts currently used for urethroplasty (eg, buccal mucosa) is that the material is “off the shelf.” This eliminates the necessity of additional surgical procedures for graft harvesting, which may decrease operative time, and the potential morbidity attributable to the harvest procedure.
These techniques using nonseeded acellular matrices were successfully applied experimentally and clinically for onlay urethral repairs. When tubularized urethral repairs were attempted experimentally, however, adequate urethral tissue regeneration was not achieved and complications ensued, such as graft contracture and stricture formation. Tubularized collagen matrices seeded with cells have performed better in animal studies. In a rabbit model, entire urethral segments were resected and urethroplasties were performed with tubularized collagen matrices seeded with autologous cells or without cells. The tubularized collagen matrices seeded with autologous cells formed new tissue that was histologically similar to native urethral tissue. The tubularized collagen matrices without cells lead to poor tissue development, fibrosis, and stricture formation.
Bladder
Currently, gastrointestinal segments are commonly used for bladder replacement or repair. Gastrointestinal tissues are designed to absorb solutes that urinary tissue excretes, however, and because of this difference in function, multiple complications may ensue, such as infection, metabolic disturbances, urolithiasis, perforation, increased mucus production, and malignancy. Because of the problems encountered with the use of gastrointestinal segments, numerous investigators have attempted alternative reconstructive procedures for bladder replacement or repair. The use of tissue expansion, seromuscular grafts, matrices for tissue regeneration, and tissue engineering with cell transplantation has been investigated.
Bladder Replacement Using Tissue Engineering
Cell-seeded allogeneic acellular bladder matrices have been used for bladder augmentation in dogs. A group of experimental dogs underwent a trigone-sparing cystectomy and were randomly assigned to one of three groups. One group underwent closure of the trigone without a reconstructive procedure, another group underwent reconstruction with a nonseeded bladder-shaped biodegradable scaffold, and the last group underwent reconstruction using a bladder-shaped biodegradable scaffold that was seeded with autologous urothelial and smooth muscle cells.
The cystectomy-only and nonseeded controls maintained average capacities of 22% and 46% of preoperative values, respectively. An average bladder capacity of 95% of the original precystectomy volume was achieved in the cell-seeded tissue-engineered bladder replacements, however ( Fig. 3 ). The subtotal cystectomy reservoirs that were not reconstructed and the polymer-only reconstructed bladders showed a marked decrease in bladder compliance (10% and 42% of total compliance, respectively). The compliance of the cell-seeded tissue-engineered bladders was almost no different from preoperative values (106%). Histologically, the nonseeded scaffold bladders presented a pattern of normal urothelial cells with a thickened fibrotic submucosa and a thin layer of muscle fibers. The retrieved tissue-engineered bladders showed normal cellular organization, consisting of a trilayer of urothelium, submucosa, and muscle.
A clinical experience involving engineered bladder tissue for cystoplasty reconstruction was conducted starting in 1999. A small pilot study of seven patients was reported, using a collagen scaffold seeded with cells with or without omentum coverage or a combined PGA-collagen scaffold seeded with cells and omental coverage ( Fig. 4 ). The patients reconstructed with the engineered bladder tissue created with the PGA-collagen cell-seeded scaffolds showed increased compliance, decreased end-filling pressures, increased capacities, and longer dry periods ( Fig. 5 ). Although the experience is promising in terms of showing that engineered tissues can be implanted safely, it is just a start in terms of accomplishing the goal of engineering fully functional bladders. Further experimental and clinical work is being conducted.
Ureters
Ureteral nonseeded matrices have been used as a scaffold for the ingrowth of ureteral tissue in rats. These matrices promoted regeneration of the ureteral wall components. Ureteral replacement with polytetrafluoroethylene grafts was also attempted in dogs but with poor functional results. In a more recent study, nonseeded acellular collagen matrices were tubularized and used to replace 3-cm segments of canine ureters. The nonseeded acellular matrix tube was not able to replace the segment of the ureter successfully.
Cell-seeded biodegradable polymer scaffolds have been used with more success to reconstruct ureteral tissues. In one study, urothelial and smooth muscle cells isolated from bladders and expanded in vitro were seeded onto tubular PGA scaffolds and implanted subcutaneously into athymic mice. After implantation, the urothelial cells proliferated to form a multilayered lining of the tubular structure, whereas the smooth muscle cells organized into multilayered structures surrounding the urothelial cells. Abundant angiogenesis was evident. The degradation of the polymer scaffolds resulted in the eventual formation of natural urothelial tissues. This approach was expanded to replacement of ureters in dogs.
Tissue engineering of specific urologic and genital structures
Urethra
Various strategies have been proposed over the years for the regeneration of urethral tissue. Woven meshes of PGA have been used to reconstruct urethras in dogs. Also, PGA has been used as a cell transplantation vehicle to engineer tubular urothelium in vivo. SIS without cells was used as an onlay patch graft for urethroplasty in rabbits. Finally, a homologous graft of acellular urethral matrix was also used in a rabbit model.
Bladder-derived acellular collagen matrix has proved to be a suitable graft for repair of urethral defects in rabbits. In the rabbit model, the neourethras created with these matrices demonstrated a normal urothelial luminal lining and organized muscle bundles shortly after repair. These results were confirmed clinically in a series of patients with a history of failed hypospadias reconstruction, wherein the urethral defects were repaired with human bladder acellular collagen matrices ( Fig. 2 ). One of the advantages of this material over nongenital tissue grafts currently used for urethroplasty (eg, buccal mucosa) is that the material is “off the shelf.” This eliminates the necessity of additional surgical procedures for graft harvesting, which may decrease operative time, and the potential morbidity attributable to the harvest procedure.
