Fig. 27.1
Standard membrane filtration (a), adsorption (b), and selective filtration (c) systems. (a) Blood is pumped into a biocompatible membrane that allows the filtration of plasma while retaining cellular elements. Red cells, leukocytes, and platelets are reinfused to the patient along with replacement fluid. Plasma is pumped into a collection bag and discarded. (b) Following the separation of plasma and cellular elements, the separated plasma is passed through an adsorption column (or equivalent) which has the capacity to remove specific factors from the plasma by selectively binding to the active component of the device. The processed plasma is returned to the patient without the need for replacement fluid. (c) Following the separation of plasma and cellular elements, the separated plasma is passed through a membrane (or another device) which uses specific physicochemical properties to remove selected components from the plasma. The processed plasma is returned to the patient without the need for replacement fluid
There are a number of potential advantages of membrane filtration over centrifugal apheresis. The first advantage is its simplicity. Since the procedure can be performed using standard conventional or continuous hemodialysis machines, hemodialysis nurses may be comfortable using such a system. Second, the plasma separator membranes do not decrease the platelet count, whereas significant reduction in platelet count (up to 50 % reduction) may be observed with centrifugation. Third, no leukocyte loss is observed with membrane filtration, whereas in centrifugation, plasma may be contaminated with leukocytes. There are, however, a number of potential disadvantages to membrane filtration. The first disadvantage is that membrane filtration devices can only be used to isolate plasma. Centrifugation machines, on the other hand, can usually be adapted for either plasmapheresis or cytapheresis procedures, such as leukocytopheresis for leukemia, erythrocytopheresis for sickle cell disease, or thrombocytopheresis for symptomatic thrombocytosis. A second disadvantage of membrane filtration is the possible activation of complement and leukocytes on the artificial membrane. In addition, membranes may trigger anaphylactoid reactions; for instance, anaphylactoid reactions have been reported with membrane apheresis in patients taking angiotensin-converting enzyme inhibitors [7]. Finally, membrane filtration usually requires a large vein (central) catheter to obtain adequate blood flow rates, whereas peripheral venipunctures may suffice for centrifugal apheresis.
Processing of Separated Plasma
The plasma separated from the cells can be either discarded or reinfused to the patient after specific processing procedures. These specialized processing methods have been developed over the years with the goals of more selectively removing the pathogenic circulating factors and limiting the loss of plasma components. With these techniques, the plasma separated from the cells is further processed to eliminate only the purported pathogen(s). It is then returned to the patient without the need for replacement fluid. These approaches include adsorption techniques and selective filtration techniques (Fig. 27.1).
For adsorption techniques, the separated plasma is passed through a device (an adsorption column or equivalent) which has the capacity to remove specific components from the plasma by selectively binding them to the active component of the device. Adsorption columns have been designed to remove (1) IgG and immune complexes (using immobilized Staphylococcus aureus protein A which binds specifically to the Fc segment of IgG), (2) nonspecific immunoglobulins (using immobilized antihuman immunoglobulin antibodies), (3) anti-DNA and anticardiolipin antibodies (using immobilized dextran sulfate), (4) low-density lipoprotein (LDL) (using immobilized anti-LDL antibodies), and (5) endotoxin and inflammatory cytokines (using immobilized polymyxin B and others adsorbers) [8–12].
For selective filtration techniques, the separated plasma is passed through a membrane or another device which uses specific physicochemical properties to remove selected components from the plasma (Fig. 27.1). A variety of devices have been designed to remove selected components including (1) membrane filters with different pore diameters and filtration/adsorption characteristics (double filtration or cascade filtration) and (2) thermoregulation units to either warm the plasma (thermofiltration) or cool the plasma (cryofiltration) to enhance the removal of specific components [13–15].
The major advantage of selective plasmapheresis techniques over standard plasmapheresis is the possibility to reinfuse the treated plasma to the patient, therefore obviating the need to use either albumin or fresh frozen plasma. Hence, the side effects related to the use of replacement solutions (e.g., anaphylactoid reactions to fresh frozen plasma, coagulopathies induced by inadequate replacement of clotting factors, transmission of viral hepatitis and other infections) are eliminated. There are however disadvantages to selective plasmapheresis techniques. Additional equipment or devices are required which may add to the cost and the complexity of the therapies. These additional devices (i.e., membrane filters or ligands used in adsorption columns) may trigger allergic reactions or may cause adverse reactions in some patients. Finally, most of the selective procedures are considered investigational as only a limited number of studies have compared selective procedures to standard plasmapheresis [16, 17]. Therefore, clinical trials are needed to better appraise the place of these procedures among the various modalities of treatment available.
Anticoagulation
To prevent activation of the clotting mechanisms within the extracorporeal circuit, an anticoagulant is usually added to the patient’s blood as it is withdrawn. The most frequently used anticoagulant for centrifugation procedures is citrate which chelates ionized calcium. A continuous infusion of acid citrate dextrose (ACD) is given intravenously during the procedure. The amount of citrate delivered to the patients is proportional to the volume of blood treated, the duration of the procedure, and the use of plasma products (which are collected and stored with citrate). Symptoms and signs of citrate toxicity include a metallic taste in the mouth, a decrease in blood pressure, paresthesia, muscle twitching, tetany, and prolonged Q-T interval. Symptomatic hypocalcemia resulting from citrate infusion complicates 1.5–9 % of treatments [18, 19]. Mild signs and symptoms usually resolve by simply interrupting the administration of citrate for a few minutes to allow time for metabolism of the citrate. In more symptomatic patients, infusion of calcium chloride or calcium gluconate should be given.
Standard unfractioned heparin is the most frequently used anticoagulant for membrane plasmapheresis. The required dose of heparin is approximately twice that needed for hemodialysis, because a substantial amount of the infused heparin is removed along with the plasma. If heparin is used in patients with a high risk of bleeding, the activated clotting time (ACT) or the partial thromboplastin time (PTT) should be monitored closely. Heparin-induced bleeding may be reversed with protamine.
Replacement Fluids
The typical replacement fluids are fresh frozen plasma or other plasma derivatives (e.g., cryosupernatant), 5 % albumin, colloidal starches, and crystalloids (e.g., 0.9 % saline, Ringer’s lactate). The choice of fluid has implications for the efficacy of the procedure, oncotic pressure, coagulation, and spectrum of side effects. Fresh frozen plasma (FFP) replaces the normal proteins that have been removed. As a result, there is no depletion of coagulation factors or immunoglobulins. However, albumin or a combination of albumin and saline is usually preferred to FFP because of the risk of hypersensitivity reactions, citrate-induced symptoms, and transmission of viral infections with the latter. Albumin (5 %) is generally combined with 0.9 % saline on a 50 %:50 % (vol/vol) basis. Other alternatives to plasma include colloidal starches and 0.9 % normal saline. Colloidal starches (e.g., hetastarch, pentastarch) can be safely used as a partial replacement fluid in combination with 0.9 % saline or human albumin. Normal saline (0.9 %) can transiently satisfy volume replacement needs during plasmapheresis, but quickly equilibrates with the extravascular compartment, thereby limiting its use.
The exact composition of replacement fluids must tailored to the needs of the patient. For example, plasma is the replacement fluid of choice in patients with thrombotic thrombocytopenic purpura (TTP) because the infusion of normal plasma may contribute to the replacement of a deficient plasma factor. Plasma may also be preferable in patients at risk of bleeding (e.g., those with liver disease or disseminated intravascular coagulation) or requiring intensive therapy (e.g., daily exchanges for several weeks). When replacement solutions other than FFP are used, a post-pheresis depletion coagulopathy is expected in most patients. With a typical 1.0–1.5 plasma volume exchange, the immediate post-exchange levels of most coagulation factors are expected to be approximately 25 % of their pre-procedure values. The exceptions are factors VIII and IX, which are reduced to lesser degrees [20]. The importance of this coagulopathy depends on the clinical situation. Most patients with no underlying hemostatic risk tolerate these changes well and require no supplementation with coagulation factors. In patients with underlying increased bleeding risk, it is reasonable to include 25 % FFP replacement to ensure adequate post-pheresis hemostasis.
Vascular Access
Adequate vascular access is required to allow the high blood flow rates (i.e., 50–150 mL/min) necessary to remove the typical amount of plasma (4–4.5 L) over the course of a few hours during standard plasmapheresis procedures. For discontinuous (or intermittent) procedures, a single large vein or a single-lumen catheter is adequate. For continuous-flow techniques, two venous access sites are required, one for blood drawing and the other for blood return. Two large veins or a double-lumen catheter are thus required. For patients with large veins and for whom large number of procedures are not expected, antecubital and forearm veins may be adequate. For all other patients, a double-lumen catheter which is rigid enough to withstand significant flow and pressures is preferable. For urgent procedures and short-term therapy, a femoral line may be the best option. For patients with non-urgent indications who are likely to require long-term therapy, placement of a tunneled catheter is preferable.
Exchange Volume
In most instances, 4–4.5 L of plasma are removed or processed during a typical plasmapheresis procedure, which corresponds to 1.0–1.5 times the plasma volume. A single plasma volume exchange will remove approximately 63 % of the IgM and IgG found in the intravascular compartment, and an exchange equal to 1.5 times the plasma volume will remove roughly 78 % of the intravascular immunoglobulins [21]. The following formula can be used to estimate the plasma volume in adults:
![$$ \begin{array}{llll}\text{Plasma}\text{}\text{volume}=\\ \text{}\text{}\text{}\text{}\left(0.65\times \text{Weight}\left[\text{kg}\right]\right)\times \left(100-\text{Hematocrit}\left[\%\right]\right)\end{array}$$](/wp-content/uploads/2016/10/A216852_1_En_27_Chapter_Equ00271.gif)
![$$ \begin{array}{llll}\text{Plasma}\text{}\text{volume}=\\ \text{}\text{}\text{}\text{}\left(0.65\times \text{Weight}\left[\text{kg}\right]\right)\times \left(100-\text{Hematocrit}\left[\%\right]\right)\end{array}$$](/wp-content/uploads/2016/10/A216852_1_En_27_Chapter_Equ00271.gif)
For example, the plasma volume of a 70 kg man with a hematocrit of 40 % is estimated at 2.7 L. Therefore, a typical exchange volume of 1.5 times the plasma volume for this patient will be around 4 L (1.5 × 2.7 L).
Apheresis Schedule
The American Association of Blood Banks general recommendation for conditions requiring plasmapheresis is that one exchange be performed every second or third day, each exchange consisting of 1–1.5 plasma volumes, for a total of 3–5 procedures [22]. In some conditions, such as thrombotic thrombocytopenic purpura, plasma exchanges should be performed daily until the platelet count is normal for 2–3 consecutive days (see below). Another exception is anti-glomerular basement membrane mediated disease; in this disorder, plasma exchanges should be performed on a daily basis for at least 2 weeks (see below).
Apheresis Physiological Principles
Many of the disorders for which plasmapheresis is advocated are thought to have an immunological basis, and plasmapheresis is often thought to work by removing immunoglobulins or immune complexes [23]. Plasmapheresis has also been proposed for removing abnormal circulating proteins in disorders such as in multiple myeloma and other dysproteinemias [24]. The removal of thrombotic factors has been suggested to explain the effect of plasmapheresis in TTP [25]. Infusion of normal plasma may itself have beneficial effects. Indeed, there is evidence that replacement of a deficient plasma component may be the principal mechanism of action of plasmapheresis in TTP [26]. Other theoretical beneficial effects on immune function include depletion of complement products, fibrinogen, and some cytokines, alterations in idiotypic/anti-idiotypic antibody balance, and improvement in reticuloendothelial system function [27–29].
Efficacy of Plasmapheresis in Specific Renal Diseases
In the following section, the therapeutic use of plasmapheresis in specific acute renal conditions is reviewed. For each condition, the clinical studies that evaluated its efficacy are summarized with special emphasis on the results of randomized controlled trials, when available. Consensus plasma exchange regimens are presented for diseases in which there are evidences to support its use. Table 27.1 provides the list of renal conditions for which plasmapheresis may be attempted along with the American Society for Apheresis (ASFA) categories assignment and recommendation grade [2]. The ASFA categories are Category I—Therapeutic apheresis is acceptable, as first-line therapy, either as primary stand-alone treatment or in conjunction with other modes of treatment; Category II—Therapeutic apheresis is acceptable, as second-line therapy, either as a stand-alone treatment or in conjunction with other modes of treatment; Category III—Disorders for which the optimum role of apheresis therapy is not established; and Category IV—Disorders for which published evidence demonstrates or suggests apheresis to be ineffective or harmful. Clinical applications should be undertaken only under an approved research protocol. The above guidelines are not intended to mandate plasmapheresis for conditions in which it is clearly efficacious nor are they intended to deny or exclude patients from receiving plasmapheresis when some benefit, although small, may be realized. Given the complexity, expense, and risks of the procedure, however, the guidelines provide a framework for clinical decisions regarding the use of plasmapheresis.
Table 27.1
Indications for plasmapheresis in renal conditions
Conditions | ASFA category | Recommendation grade |
---|---|---|
Rapidly progressive glomerulonephritis: | ||
Anti-GBM disease | I | 1a–b |
Pauci-immune RPGN | Ia | 1a |
Immune complex RPGN | IIIb | 2b |
Multiple myeloma: | ||
Myeloma cast nephropathy | II | 2b |
Hyperviscosity | I | 1b |
HUS—TTP: | ||
Primary—idiopathic (adult) | I | 1a |
Diarrhea associated (pediatric) | IVc | 1c |
Renal transplantation: | ||
ABO and HLA desensitization | II | 1b |
Antibody-mediated rejection | I | 1b |
Recurrent disease (FSGS) | I | 1c |
Rapidly Progressive Glomerulonephritis
Causes of rapidly progressive glomerulonephritis (RPGN) can be divided into three main groups based upon the immunofluorescence pattern on renal biopsy. Anti-glomerular basement membrane (anti-GBM) antibody disease accounts for 15 % of cases, pauci-immune crescentic glomerulonephritis for 60 % of cases and immune complex crescentic glomerulonephritis for 25 % of cases.
Anti-Glomerular Basement Membrane Antibody Disease
Anti-GBM antibody disease typically presents as RPGN without or with pulmonary hemorrhage (Goodpasture’s syndrome). Circulating anti-GBM antibodies are detected in greater than 90 % of patients and, in general, disease activity correlates with the titer of circulating antibodies. The rationale for plasmapheresis is the rapid removal of toxic anti-GBM antibodies.
The specific role of plasmapheresis in therapeutic regimens for anti-GBM disease has never been properly assessed by prospective randomized controlled trials. Only two controlled studies have evaluated the efficacy of plasmapheresis as an adjunct to conventional immunosuppressive therapy in this disease [30, 31]. Although small (17 and 20 patients), both studies suggested a benefit as evidenced by faster decline in anti-GBM antibody titers, lower serum creatinine after therapy, and fewer patients progressing to renal failure. However, the authors were cautious about accepting that plasmapheresis had been responsible for the improved outcome, as the groups receiving plasmapheresis had milder disease than the control groups.
The results of more than 20 uncontrolled studies and case reports including close to 450 patients, published over the past 20 years support the results of the above controlled studies. In aggregate, these reports suggest that survival rates of greater than 80 % and renal preservation rates of greater than 45 % may be obtained with therapeutic regimens combining plasmapheresis with immunosuppressive drugs (reviewed in references [32, 33]). These results compare favorably with historical data suggesting patient survival of 45 % and progression to ESRD in 85 %.
Thus, there is evidence, although largely based on uncontrolled or retrospective studies with historical comparison, that plasmapheresis is a useful adjunct to immunosuppressive drugs. Plasmapheresis can accelerate disappearance of anti-GBM antibodies and improve renal function if instituted promptly. Several series have demonstrated that patients with severe disease (oliguria, dialysis, or serum creatinine >600 μ[mu]mol/L—6.8 mg/dL) are unlikely to regain renal function despite the use of plasmapheresis. Hence, in such patients, plasmapheresis should probably be reserved for treatment of pulmonary hemorrhage. The recommended regimen for anti-GBM disease is the following: daily plasmapheresis for 14 days, with 4 L exchanges and albumin solution as replacement fluid. Response to therapy should be monitored by repeated assessments of urine output, serum creatinine, and plasma anti-GBM levels.
Pauci-immune Rapidly Progressive Glomerulonephritis
Pauci-immune RPGN is characterized by minimal immune deposits in the glomerulus and the presence of antineutrophil cytoplasmic antibodies (either C-ANCA or P-ANCA) which may contribute to the pathophysiology of RPGN. In the majority of these patients, RPGN is caused by granulomatosis with polyangiitis (GPA, formally Wegener’s granulomatosis) and microscopic polyangiitis (MPA) or “renal-limited” pauci-immune GN.
Seven randomized controlled trials have evaluated the efficacy of plasmapheresis as an adjunct to immunosuppressive therapy in patients with pauci-immune RPGN [34–39]. Three studies randomly assigned patients to receive immunosuppressive agents with or without plasmapheresis and found no statistically significant difference between the two groups as judged by serum creatinine or dialysis dependency [34, 35, 39]. Three other studies provided evidence of a benefit in subgroups of patients with severe disease [36–38]. Specifically, patients on dialysis or with a serum creatinine >800 μ[mu]mol/L—9 mg/dL were more likely to respond to plasmapheresis than patients with milder disease. Similarly, in a recent randomized controlled trial, the role of plasma exchange in patients with severe renal disease was studied in the methylprednisolone versus plasma exchange (MEPEX) trial [40]. This study enrolled 137 patients with a new diagnosis of pauci-immune RPGN (GPA or MPA) and a serum creatinine concentration above 5.8 mg/dL—500 μ[mu]mol/L. As compared with intravenous methylprednisolone, plasmapheresis was associated with a reduction in risk of progression to ESRD of 24 %, from 43 % to 19 %, at 12 months. However, none of the above randomized controlled trials reported improvements in patient survival with plasmapheresis.
In aggregate, these results suggest a benefit of plasmapheresis when used as an adjunct to immunosuppressive therapy in patients who have advanced renal dysfunction at presentation, as defined by a serum creatinine level above 500 μ[mu]mol/L—5.8 mg/dL and/or dialysis dependence. In addition, limited data suggest that patients with pulmonary hemorrhage may also benefit from prompt initiation of apheresis coupled with aggressive immunosuppressive therapy [41, 42]. Given the paucity of convincing data, however, it is impossible to provide firm recommendations regarding the specifics of therapy for patients with pauci-immune RPGN. It would seem prudent to reserve plasmapheresis for severe cases and to perform at least four plasmapheresis sessions during the first week of immunosuppressive therapy, using 4 L exchanges and albumin solution as replacement fluid. Response to therapy should be monitored with repeated assessments of urine output, serum creatinine, and possibly ANCA titers.
Immune Complex Crescentic Glomerulonephritis
Immune complex RPGN is characterized by granular immune deposits on immunofluorescence microscopy and is caused by a number of immune disorders including lupus nephritis, cryoglobulinemia, IgA nephropathy, Henoch–Schönlein purpura, post-streptococcal GN, and membranoproliferative GN. Plasmapheresis has been advocated mainly in the treatment of lupus, cryoglobulinemia, and IgA nephropathy/Henoch–Schönlein purpura.
Lupus Nephritis
Overt nephritis complicates 38–90 % of cases of systemic lupus erythematosus. Plasmapheresis has been advocated as a means of rapidly removing circulating autoantibodies and immune complexes that appear to play a key role in the pathophysiology of lupus nephritis.
Although initial case reports and uncontrolled case series suggested a benefit of plasmapheresis in severe lupus nephritis, a large multicenter prospective randomized controlled trial provided strong evidence against its use [43]. The Lupus Nephritis Collaborative Study Group assessed the value of plasmapheresis as an adjunct to prednisone and cyclophosphamide in 86 patients with severe lupus nephritis (serum creatinine value >2.0 mg/dL or 180 μ[mu]mol/L). Plasmapheresis caused a rapid reduction of serum anti-dsDNA antibodies and cryoglobulins. However, the percentage of patients progressing to renal failure (25 versus 17 %) and going into clinical remission (30 versus 28 %) was the same in both groups. Importantly, patients receiving plasmapheresis tended to have a worse outcome. Four other randomized controlled trials of plasmapheresis reported similar findings [44–47]. Plasmapheresis produced significant reduction in circulating immune complexes and anti-DNA antibodies, but the frequency and degree of partial or complete remission was the same in both plasmapheresis and control groups [44–47]. In addition, intensified treatment protocols that combine plasmapheresis with subsequent pulse cyclophosphamide have also been showed to provide no additional benefit when compared with pulse cyclophosphamide [48].
In summary, the results of multiple prospective randomized controlled trials do not support a role for plasmapheresis in the treatment of lupus nephritis. There is experimental and clinical evidence that plasmapheresis induces rapid removal of circulating immune complexes and anti-DNA antibodies, but plasmapheresis does not appear to influence renal function, remission rate, or mortality. However, plasmapheresis may have a role in lupus associated with antiphospholipid antibodies. Selected patients with acute renal failure and the antiphospholipid syndrome may respond to plasmapheresis, which presumably acts by removing the pathogenic antibody. In one uncontrolled case series of 12 cases, for example, renal recovery occurred in patients treated with plasma exchange [49]. Similarly, plasmapheresis may have a role in lupus associated with thrombotic thrombocytopenic purpura (see below).
Cryoglobulinemia
Cryoglobulins comprise immunoglobulins that reversibly precipitate at low temperature. The aggregates of cryoglobulins can deposit within the glomerular capillary lumen and cause damage by activating complement and recruiting leukocytes. Plasmapheresis has been reported to remove cryoglobulins efficiently. Case series and case reports suggest 55–87 % improvement in renal function and significant improvement in overall survival (from 55 % to 25 % mortality) with plasmapheresis [50–56]. Unfortunately, the specific role of plasmapheresis in therapeutic regimens for cryoglobulinemia has never been assessed by prospective randomized controlled trials. In addition, with the recognition that hepatitis C virus (HCV) is the etiologic agent for approximately 80 % cases of mixed essential cryoglobulinemia, a major theoretical concern with plasmapheresis is a possible enhancement of HCV replication. Hence, it would appear prudent to limit plasmapheresis to patients with acute fulminant disease.
A reasonable plasmapheresis prescription is to exchange one plasma volume three times weekly for 2–3 weeks. The replacement fluid can be 5 % albumin, which must be warmed to prevent precipitation of circulating cryoglobulins [57]. The optimal method for assessing the efficacy of plasmapheresis is uncertain. Changes in the percent cryocrit after plasmapheresis do not correlate closely with clinical activity. Thus, response to therapy should be monitored with changes in clinical manifestations.
IgA Nephropathy and Henoch–Schönlein Purpura
IgA nephropathy and Henoch–Schönlein purpura which are characterized by IgA immune complexes deposits present with acute rapidly progressive glomerulonephritis in approximately 10 % of cases. Plasmapheresis has been advocated to rapidly remove circulating IgA immunoglobulins, although circulating IgA levels do not correlate with severity or activity of disease.
Uncontrolled reports in patients with crescentic, rapidly progressive glomerulonephritis suggest possible benefit from plasmapheresis and immunosuppressive therapy [58–61]. One report evaluated the efficacy of aggressive combination therapy (including pulse methylprednisolone, oral cyclophosphamide, and plasmapheresis) in six patients with crescentic glomerulonephritis due to IgA nephropathy [61]. All patients improved in the short term but subsequent deterioration in renal function was observed in more than half of these patients. No randomized controlled trials have evaluated the efficacy of plasmapheresis in therapeutic regimens for rapidly progressive IgA nephropathy and Henoch–Schönlein purpura.
In summary, no firm conclusions can be drawn regarding the efficacy of plasmapheresis in therapeutic regimens for rapidly progressive IgA nephropathy and Henoch–Schönlein purpura because of lack of evidence. Hence, its use should be limited to fulminant cases in the context of research protocols.
Hemolytic Uremic Syndrome and Thrombotic Thrombocytopenic Purpura
The presenting features of hemolytic uremic syndrome (HUS) and thrombotic thrombocytopenic purpura (TTP) are essentially the same in most patients, beginning with thrombocytopenia and microangiopathic hemolytic anemia without apparent cause; in addition, most patients have neurologic and/or renal abnormalities. Several factors may contribute to the pathogenesis of HUS–TTP including genetic factors, drugs (e.g., quinine, cyclosporine, antiplatelet agents, chemotherapeutic agents), infections (e.g., Shiga toxin-producing Escherichia coli), autoimmune disorders (e.g., antiphospholipid syndrome or systemic lupus erythematosus), and either congenital or acquired (e.g., autoantibodies) ADAMTS-13 deficiency. Therapeutic plasma exchange has been proposed to benefit patients with HUS–TTP by (1) replacing a deficient plasma factor and/or (2) removing circulating toxins that cause endothelial injury and/or platelet aggregation and promote formation of microthrombi.
There has been no controlled, prospective, randomized, appropriately blinded study comparing plasma exchange with placebo or drug therapy in the treatment of HUS-TTP. Most of the evidence in favor of the role of plasmapheresis originates from uncontrolled or retrospective studies and from comparison with historical data. Prior to the introduction of plasma infusion and plasmapheresis, the disease typically progressed rapidly and was almost uniformly fatal (93 % fatality rate; 79 % within 90 days) [62]. With plasmapheresis using fresh frozen plasma, remission rates of greater than 75 % and survival rates greater than 85 % are now consistently reported. It is unclear whether this benefit is due to plasma infusion alone and replacement of a deficient plasma factor or to plasma exchange and removal of a circulating toxic. Two randomized controlled trials compared plasma exchange to plasma infusion [63, 64]. Rock et al. randomized patients with TTP to either plasma exchange or plasma infusion with fresh frozen plasma and observed that patients receiving plasma exchange had a better response rate and superior survival [63]. Patients treated with plasma exchange received approximately three times as much plasma as those treated with plasma infusion alone (in whom the degree of plasma administration was limited by the risk of volume overload). Thus, the apparent enhanced benefit from plasma exchange may have been due to infusion of more plasma rather than to the removal of some toxic substance. Indeed, a smaller controlled trial did not observe a difference in outcome when patients were randomized to receive either daily infusions of 15 mL/kg of fresh frozen plasma or plasma exchange with a mixture of 15 mL/kg of fresh frozen plasma and 45 mL/kg of 5 % albumin as replacement fluid [64]. Thus, the exact roles of plasma removal versus plasma infusion in the beneficial effect of plasma exchange remain controversial. In practice, this question is rather semantical since it is often necessary to perform plasma exchange in order to administer the required large amount of plasma, given that these patients are often oligo-anuric and at risk for hypervolemia and pulmonary edema.
In summary, there is evidence, largely based on studies using historical controls, that plasma exchange improves renal outcome and mortality in adult patients with HUS-TTP. The most widely recognized plasma exchange protocol involves daily plasma exchange for 7–14 days, using 4 L exchanges and fresh frozen plasma (FFP) as replacement fluid. Response to therapy is monitored with repeated assessments of platelet counts, serum lactate dehydrogenase (LDH) concentration, urine output, and serum creatinine values. Plasma exchange is initially performed daily until the platelet count has normalized and hemolysis largely ceased, as evidenced by a normal LDH concentration. When a normal platelet count has been achieved, plasma exchange is usually discontinued or gradually tapered by increasing the interval between treatments.
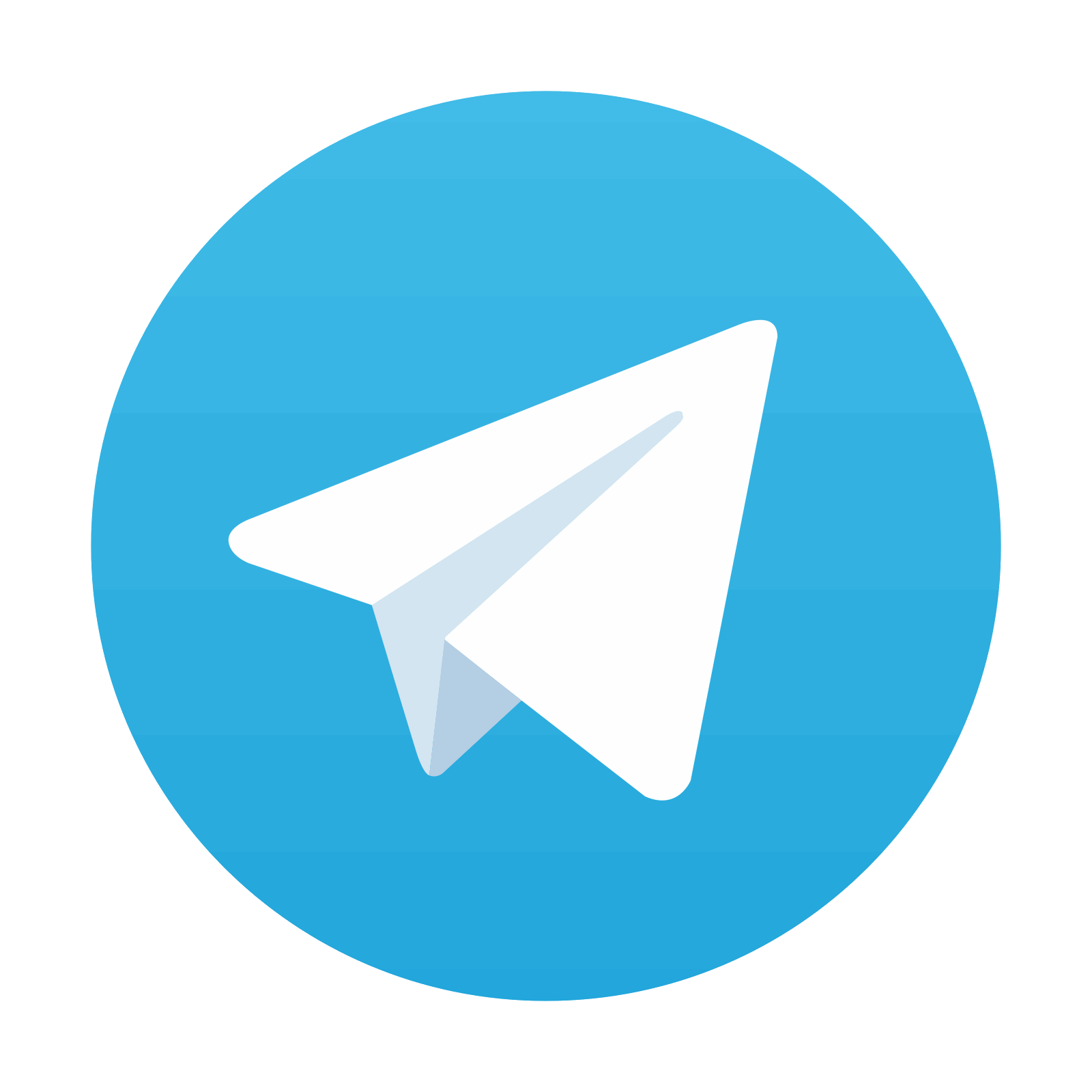
Stay updated, free articles. Join our Telegram channel

Full access? Get Clinical Tree
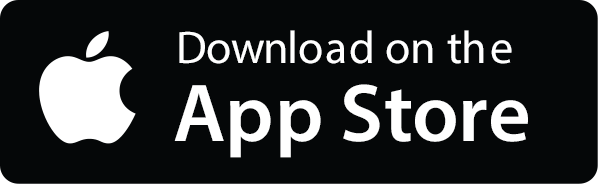
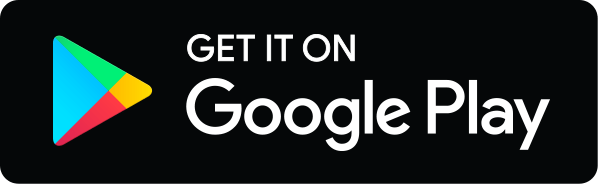