Physiology of the Uterus and Cervix
Eric J. Bieber
The uterus, and specifically the endometrium, is a dynamic and ever-changing organ throughout much of a woman’s life. The fundamental physiologic processes that occur from menarche to menopause must be well understood by physicians to allow appropriate diagnosis and treatment of underlying disorders. As the end-organ of the hypothalamic-pituitary-ovarian-uterine axis, the uterus and endometrium may be significantly affected by disorders impacting at any level of this axis.
Although the cervix is not as intrinsically sensitive as the endometrium to hormonal change, significant variation does occur with age, parity, and, to a lesser degree, steroidal variation. The hysteroscopist must have a knowledge of the variations in uterine and cervical physiology to optimize hysteroscopic visualization and minimize complications that may make the difference between looking into a “sea of red” and having a crystal-clear field that will allow the best opportunity to make the correct diagnosis.
This chapter will focus on the endocrine events that affect the endometrium and cervix in both physiologic and pathologic states. The relationship and significance of these states relative to performance and optimization of hysteroscopic surgery will then be discussed.
The Hypothalamic-Pituitary-Ovarian-Uterine Axis
In the normally menstruating female, it is the coordinated sequence of events beginning at the hypothalamus and pituitary that allows appropriate follicular development, steroidal secretion, ovulation, and corpus luteum function. The endometrium is intimately tied to the function or dysfunction of the hypothalamus, pituitary, or ovary as an end-organ responsive to estrogen and progestational stimuli.
The Hypothalamus
The hypothalamus is found at the base and sides of the third ventricle. Condensations of neurons form the arcuate nucleus and other areas of importance, such as the paraventricular and supraoptic nuclei and the preoptic region. These locations at the base of the diencephalon receive significant neuronal communication from higher brain regions. Investigations have documented the stimulatory effects of norepinephrine and the inhibitory effect of dopamine on gonadotropin-releasing hormone (GnRH) release (Fig. 3.1). Opioid peptides have also been documented to have a direct inhibitory effect on GnRH secretion, as well as modulating the norepinephrine and dopaminergic pathways. The clinical consequences of changes in these systems may be seen as oligo-ovulation or anovulation induced via such events as significant weight changes, exercise, and stress.
GnRH is a relatively small 10-amino-acid peptide. It is released into the portal circulation, which then flows to the anterior pituitary and stimulates gonadotropes.
GnRH is secreted in a pulsatile fashion every 60 to 90 minutes, depending on the phase of the cycle. Pulses during the early follicular phase have been shown to occur every 70 minutes at the time of ovulation and to decrease to every 200 or more minutes in the late luteal phase. In elegant investigations into GnRH physiology, Knobil et al. demonstrated the ability of exogenous pulsatile GnRH to mimic the effect of native GnRH. Of great clinical relevance was the discovery that chronic or continuous GnRH stimulation caused what seemed a paradoxical decrease in gonadotropins and estrogen (Fig. 3.2). This occurs when gonadotropes are bathed continuously in GnRH versus the normal episodic pattern of release. Down-regulation of receptor sites and desensitization to GnRH have been shown to be the mechanisms involved in this response. Multiple GnRH analogues have now been developed that allow pharmacologic modification of gonadotropin release; these are discussed in the section on hormonal uterine preparation.
The Pituitary and Ovary
The physiology of the pituitary and ovary is much more complex than initially believed, with paracrine control and numerous growth factors playing important roles. The intricacy of these changes is outside the scope of this text and have been described in detail elsewhere. In response to GnRH, gonadotropes within the anterior pituitary directly release follicle-stimulating hormone (FSH) and luteinizing hormone (LH) into the system’s circulation. Whereas the
half-life of GnRH is only 2 to 4 minutes, the half-life of LH is much longer. It is the modulation of GnRH that causes variable release of gonadotropins and allows appropriate ovarian follicular development. During the follicular phase, FSH levels affect granulosa cell development, ultimately leading to a dominant follicle being selected from a cohort of developing follicles. There is a concomitant increase in estradiol-17b production. Ultimately, serum estradiol levels of >200 pg/mL for >24 to 48 hours cause a positive feedback on the hypothalamus and pituitary, resulting in the midcycle LH surge with subsequent ovulation.
half-life of GnRH is only 2 to 4 minutes, the half-life of LH is much longer. It is the modulation of GnRH that causes variable release of gonadotropins and allows appropriate ovarian follicular development. During the follicular phase, FSH levels affect granulosa cell development, ultimately leading to a dominant follicle being selected from a cohort of developing follicles. There is a concomitant increase in estradiol-17b production. Ultimately, serum estradiol levels of >200 pg/mL for >24 to 48 hours cause a positive feedback on the hypothalamus and pituitary, resulting in the midcycle LH surge with subsequent ovulation.
After ovulation, there is luteinization of the granulosa and theca cells and a marked increase in vascularity to form the corpus luteum. The corpus luteum continues to secrete progesterone for only 12 to 14 days if unrescued by human chorionic gonadotropin (hCG) production from a pregnancy.
The Uterus and Endometrium
Uterine Morphology
The uterus and endometrium are dynamic entities markedly changing from birth to puberty to menopause. At birth the uterus is small, measuring only one half the size of the cervix with the entire cervix and uterus being only 3 to 4 cm in length (Fig. 3.3). The neonatal endometrium at birth may show changes from in utero exposure to maternal steroids. In one study, proliferative endometrium was seen in 16% of patients, secretory changes in 27%, and menstrual endometrium in 5%, consistent with the fact that menstrual-type bleeding may be seen nonpathologically in the female newborn. After birth, the endometrium returns to a resting low cuboidal epithelium unless stimulated by exogenous steroids.
At menarche, the uterus and cervix respond to increases in circulating estrogen. At age 13, the cervix and uterus each measure 3 cm in length. As adulthood is reached, the uterine/cervical ratio approaches 2:1, with the uterus having an approximate length of 8 cm, a width of 5 cm, and a weight of 50 to 60 gm (see Fig. 3.3 and Chapter 2).
Uterine and Endometrial Histology
The uterus is composed histologically of myometrium and endometrium. The myometrium consists of smooth muscle fibers in a framework of rich arterial and venous blood supply supported by an underlying dense connective tissue. The normal nonpregnant adult myometrium varies in thickness from 1.5 to 2.5 cm.
The endometrium in the cycling female is an ever-changing structure (see chapter 1). It is composed of simple columnar ciliated epithelium that is supported by an underlying matrix of cellular connective tissue stroma, which includes simple glands. The endometrium is structurally divided into three layers the stratum basalis, stratum spongiosum, and stratum compactum. The stratum basalis is the deepest layer, changing little during the menstrual cycle and left intact during menstruation. This is the layer that is important to the hysteroscopist during endometrial ablation. If not completely destroyed, further endometrial regeneration will ensue.
The second and third layers are collectively considered the stratum functionalis. These are the layers that respond to ovarian steroids and are shed at the time of menstruation. The intermediate layer, the stratum spongiosum, is described as such because of the spongy appearance of the stroma, versus the stroma of the stratum compactum, which is highly dense and compact in appearance.
The second and third layers are collectively considered the stratum functionalis. These are the layers that respond to ovarian steroids and are shed at the time of menstruation. The intermediate layer, the stratum spongiosum, is described as such because of the spongy appearance of the stroma, versus the stroma of the stratum compactum, which is highly dense and compact in appearance.
![]() FIGURE 3.3 Schematic representation of the change in uterine length and cervix/corpus ratios at birth, puberty, and adulthood. |
These layers are supplied by vasculature arising from the uterine arteries. Straight arteries are short and provide the arterial supply to the more static stratum basalis. Spiral arteries, unlike the straight arteries, are highly steroid responsive and supply the rich capillary vasculature of the stratum functionalis.
Uterine Estrogen and Progesterone Receptors
Cyclicity of the endometrium of the premenopausal uterus in response to the circulating hormones estrogen and progesterone requires the presence of steroid hormone receptors in the tissue. Steroid receptors are intracellular proteins that bind their respective hormones specifically and with high affinity. An estrogen receptor, by definition, is a protein that binds with high affinity only estrogens from the group of compounds known as steroid hormones. High affinity implies that avidity of the receptor for the hormone is sufficiently great that changes in circulating concentrations of hormones are reflected by variation in the portion of receptors occupied by hormones. Affinity of a receptor for its hormone is usually presented as a dissociation constant, a molar concentration. The dissociation constant is equal to the hormone concentration at which any population of the receptor is one-half filled.
Mechanistic models of steroid hormone action are the same for all species, all steroids, and all target tissue. Recent developments, elucidating steroid hormone mechanisms of action, have emphasized that biochemical models evolve over time. It is helpful to remember that any model is only a tool to assist in the management of practical problems. Currently there are two models to explain how steroids bind to their receptors and elicit a response by target tissues (Fig. 3.4). In all studies published before 1984, data were interpreted in accordance with the original model developed in the pioneering work of Jensen and Gorski. Studies published subsequent to 1984, although similar in design and identical in method to earlier studies, report data interpreted in accordance with both models. The second model has gained a great deal of acceptance recently and may soon supersede the original. This second model was originally proposed by Sheridan et al. in 1979 (Fig. 3.4). A review of the historical development of both of these models was presented by Walters in 1985.
Distribution of estrogen and progesterone receptors within the human uterus is not uniform. The quantity of both receptors in myometrium has been reported as two to ten times less than in endometrium. The biochemical properties of the myometrial steroid receptors, however, do not differ from those of endometrial receptors. It has also been reported that there is an uneven distribution of estrogen and progesterone receptors within the endometrium. A steep decrease, approximately tenfold, in both receptors exists from fundal to cervical sections. This steep receptor gradient persists throughout the menstrual cycle and after menopause.
There is a cyclic pattern in the quantity of uterine estrogen and progesterone receptors present during the menstrual cycle. In humans, as in other species, the progesterone receptor is an estrogen-induced protein. The uteri of postmenopausal women contain normal to high levels of
estrogen receptors. However, because there is a general absence of circulating estrogen, progesterone receptors are not present.
estrogen receptors. However, because there is a general absence of circulating estrogen, progesterone receptors are not present.
Santiana de Almeida more recently has investigated estrogen and progesterone receptors in postmenopausal women with endometrial polyps. They noted a higher concentration of estrogen receptors in the polyp than the surrounding endometrium and suggest this as a role in the underlying pathophysiology.
Molecular Physiology of the Endometrium
Recent studies continue to advance our understanding of the molecular and genetic basis for endometrial function. Borthwick et al. at Cambridge evaluated changes in transcripts over the genome between secretory and proliferative phases of the menstrual cycle. They noted that 149 discrete transcripts were expressed significantly differently between the menstrual phases. Although some of the transcripts were previously known to be important for steroid modulation, many remain as yet undescribed.
The classic relationships between ligands and their receptor has also been called into question recently as there are suggestions of ligands activating multiple unrelated receptors, a receptor being activated by unrelated ligands, and co-receptors being shared by unrelated receptors (Ben-Shlomo). Ben-Shlomo discusses such three-way partnerships in the endometrium with ATP, P2X7, P2Y2, WnT, Frizzled, and RYK systems.
Multiple cytokines and growth factors are also involved in endometrial myometrial function and are likely critical to endometrial receptivity during implantation. Kayisli has reviewed the roles some of these molecules play including interleukin (IL)-8 with cervical ripening and parturition. Monocyte chemotactic protein (MCP)-1, IL -8, as well as regulated-on-activation normal T-cell expressed and secreted (RANTES) proteins likely play roles in proliferation, differentiation, angiogenesis, and apoptosis and are regulated through local growth factors such as tumor necrosis factor-alpha, interferon-gamma, interleukin -1, and other mediators. Singer et al. have reported that IL-1 alpha activity is limited to the perimenstrual phase and is involved in tissue lysis and eventually onset of menstruation. Interestingly, they also describe a negative correlation between IL-1 alpha and endometrial cancer differentiation.
Another group of molecules, the matrix metalloproteins, are also involved in the significant tissue remodeling that occurs with each menstrual cycle (Curry and Osteen). Vascular endothelial growth factor (VEGF) and other angiogenic factors are also involved in the continuous building,
differentiation, sloughing, and rebuilding that constitute the menstrual cycle (Krikun et al.).
differentiation, sloughing, and rebuilding that constitute the menstrual cycle (Krikun et al.).
The complex interplay of gonadal steroids and the many local factors alluded to in brief here contribute to the series of events that allow for implantation. It is easy to imagine how small variations in physiology might cause derangement in this cascade and create a range of disease states.
The Endometrial Cycle
The endometrium is highly sex-steroid responsive and varies in size and content depending on the relative level of estrogens and progesterone. In the ovulatory female, cycle length may range from 21 to 35 or more days. In general, the follicular phase has greater temporal variation, since the luteal phase is tied to the corpus luteum, which has a limited life span if not rescued by pregnancy. Noyes et al. have described the cyclic changes that occur from day to day and allow the endometrium to be dated. To exclude the effect of a shortened or lengthened follicular phase, endometrial dating should be compared to the time of the LH surge or the onset of the next menstrual cycle when assessing “in or out of phase.”
Menstrual Phase
The first day of menses is considered cycle day 1. During this time constriction of the spiral arteries results in tissue ischemia with subsequent necrosis (Fig. 3.5). As glands collapse, neutrophils are seen infiltrating the areas of hemorrhage and necrosis. Prostaglandins likely play a critical role in this cascade and may be directly affected by the withdrawal of progesterone from the dying corpus luteum. The menstrual flow is composed of the cellular component of the stratum functionalis and accompanying blood and may last from 3 to 7 days. Studies have suggested that a blood loss of up to 80 mL per cycle is normal, although significant variation exists. At the point where only the basal endometrium remains, the endometrial thickness is 1 mm or less. Early during this period, even prior to final cessation of menses, the endometrium will begin to repair and regrow. Ferenczy et al. have suggested that this is independent of estrogen or other sex steroids.
Proliferative Phase
From the basal endometrium, stroma and glandular growth begins. Estrogen receptors may be seen on the cells by cycle day 5, allowing subsequent rapid growth in response to increasing levels of estrogen. It should be noted that this growth may be in response to ovarian estrogens, such as estradiol-17b, or estrogens produced via extraglandular aromatization, such as estrone. The glands formed during this stage are straight and tubular structures that eventually open to the endometrial surface during the late proliferative phase (Fig. 3.6). Increasing numbers of mitotic figures are seen in both the glandular element and the connective tissue stroma in response to the mitogen-estrogen. Estrogen also induces the development of progesterone receptors in the endometrium prior to ovulation. The endometrial thickness may be 8 mm or greater prior to ovulation, representing a remarkable increase from the 1-mm menstrual basal endometrium (Fig. 3.7).
Secretory Phase
Ovulation is the initiating event of the secretory phase. Significant endometrial changes occur with the secretion of progesterone from the corpus luteum. Most important, the glandular epithelium is stimulated to secrete glycogen. Initially, 2 to 3 days after ovulation, basal vacuolization may be identified, which represents subnuclear collections of glycogen. The formation of vacuoles in the basal aspect of the glandular epithelium displaces nuclei toward the cellular apex. These vacuoles move to a supranuclear position by cycle day 18 or 4 days postovulation, eventually being excreted in preparation for possible implantation. At the same time, significant morphologic changes occur to
the glandular structure, with marked increase in complexity and tortuosity. In the late secretory phase, glands may be significantly dilated, containing glycogen-rich secretions. The connective tissue and stroma have become highly vascular and slightly edematous (Fig. 3.8).
the glandular structure, with marked increase in complexity and tortuosity. In the late secretory phase, glands may be significantly dilated, containing glycogen-rich secretions. The connective tissue and stroma have become highly vascular and slightly edematous (Fig. 3.8).
![]() FIGURE 3.7 Vaginal ultrasound demonstrating a thin endometrial stripe immediately postmenstrual (A) but markedly thickened approximately 1 week later near ovulation (B). |
Marconi et al. investigated endometrial physiology by injecting methylene blue dye into the uterine cavity, then performing microhysteroscopy and obtaining endometrial biopsies. This was performed during the mid proliferative, periovulatory, and midluteal phases. They were able to characterize several interesting and different endometrial changes that varied throughout the cycle. Figure 3.9 demonstrates the appearance during the midproliferative phase. Interestingly, the authors suggest that the endometrial glands were oriented mostly toward the ostia, possibly with a greater concentration on the side of the ovulatory ovary. Also, only during the periovulatory phase did the methylene blue fail to stain the glands or the epithelium. They speculated that glandular secretions produced at this time prevented direct contact of the methylene blue with the glands. Figure 3.10 notes several findings that we have previously noted during hysteroscopy including the “worm sign” and the “ring sign.” Hysteroscopists may note some of these findings depending on the phase of the menstrual cycle following injection of methylene blue for tubal patency.
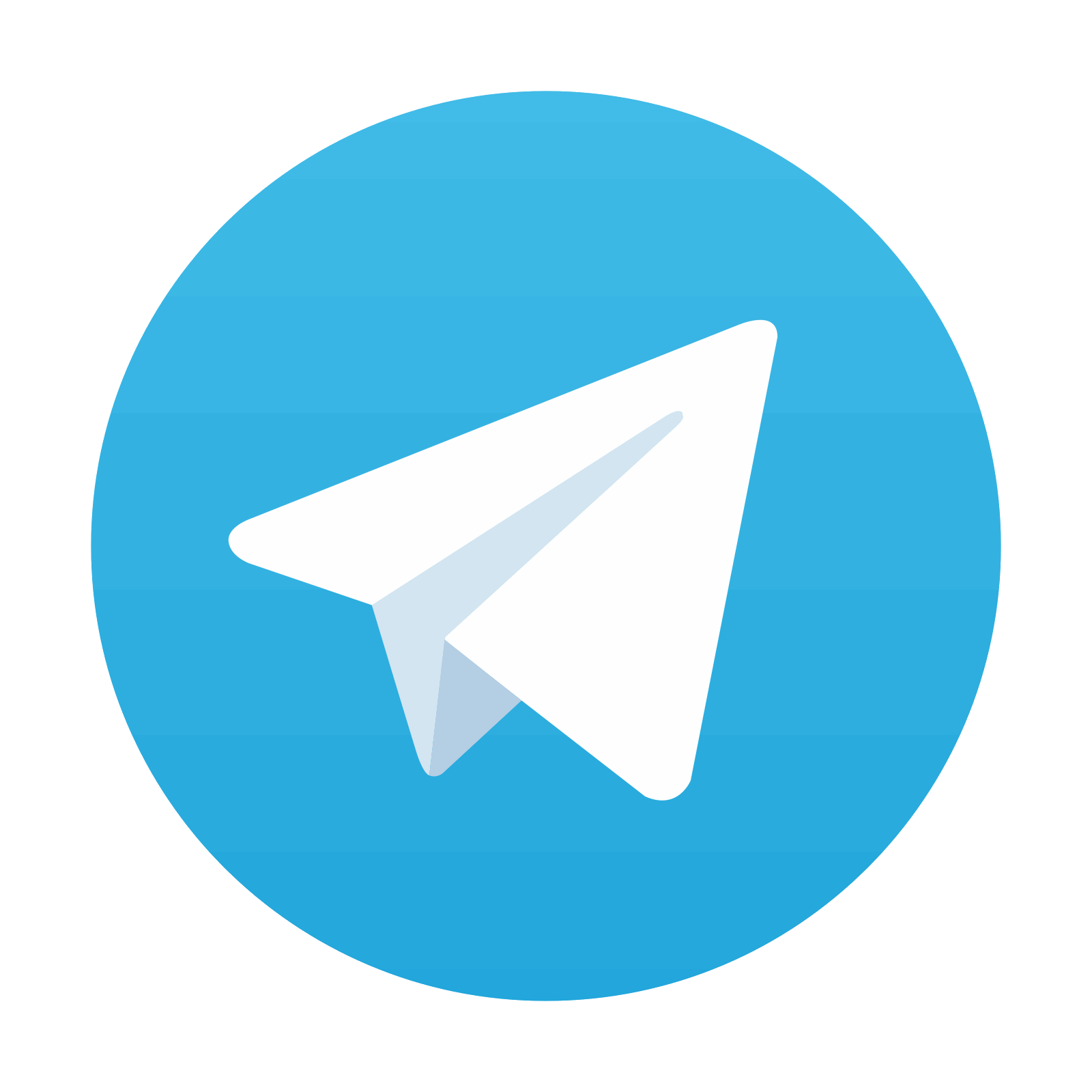
Stay updated, free articles. Join our Telegram channel

Full access? Get Clinical Tree
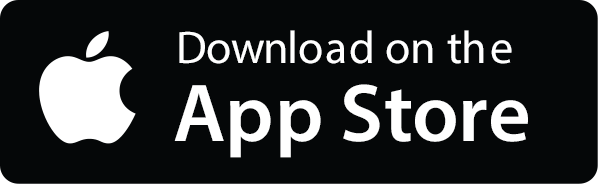
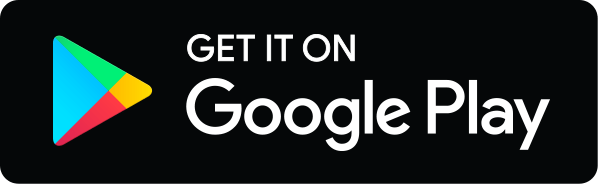
