Tom F. Lue, MD, ScD (Hon), FACS
Physiology of penile erection
Historical Aspects
The first description of erectile dysfunction (ED) dates from about 2000 BC and was set down on Egyptian papyrus. Two types were described: natural (“the man is incapable of accomplishing the sex act”) and supernatural (evil charms and spells). Later, Hippocrates reported many cases of male impotence among the rich inhabitants of Scythia and ascribed it to excessive horseback riding. Aristotle stated that three branches of nerves carry spirit and energy to the penis and that erection is produced by the influx of air (Brenot, 1994). His theory was well accepted until Leonardo da Vinci (1504) noted a large amount of blood in the erect penis of hanged men and cast doubt on the concept of the air-filled penis. His writings, however, were kept secret until the beginning of the 20th century (Brenot, 1994). Nevertheless, in 1585, in Ten Books on Surgery and the Book of Reproduction, Ambroise Paré gave an accurate account of penile anatomy and the concept of erection. He described the penis as being composed of concentric coats of nerves, veins, and arteries and of two ligaments (corpora cavernosa), a urinary tract, and four muscles. “When the man becomes inflamed with lust and desire, blood rushes into the male member and causes it to become erect,” Paré wrote. The importance of retaining blood in the penis was stressed by Dionis (1718; quoted by Brenot, 1994), who attributed this to the muscles cramping the veins at the proximal end, and by Hunter (1787), who thought that venous spasm prevented the exit of blood.
Modern investigations of penile hemodynamics began in the 1970s with xenon washout and cavernosography studies in human volunteers exposed to audiovisual sexual stimuli. These studies yielded conflicting results: Shirai and associates (1978) concluded that penile venous flow is increased during erection, but markedly increased arterial flow compensates for this; in contrast, Wagner (1981) also demonstrated increased arterial flow but concluded that venous drainage is decreased during erection.
Functional Anatomy of the Penis
The penis is composed of three cylindrical structures: the paired corpora cavernosa and the corpus spongiosum (which houses the urethra), covered by a loose subcutaneous layer and skin. Its flaccid length is controlled by the contractile state of the erectile smooth muscle and varies considerably, depending on emotion and outside temperature. In one study, penile length, measured from the pubopenile junction to the meatus, was 8.8 cm flaccid, 12.4 cm stretched, and 12.9 cm erect, with neither age nor the size of the flaccid penis accurately predicting erectile length (Wessells et al, 1996). In another study, the author concluded that about 15% of men have a downward curve during erection; erect angle is below horizontal in one quarter; and shorter erect lengths (from 4.5 to 5.75 inches) occur in 40% of men (Sparling, 1997). Since then, more studies have been reported from several countries (Awwad et al, 2005) (Table 23–1). Regarding penile morphology and erection, one study showed that, during erection, the penile buckling forces are dependent not only on intracavernous pressures but also on penile geometry and erectile tissue properties. The authors concluded that, in patients with normal penile hemodynamics but without adequate rigidity, structural causes should be investigated (Udelson et al, 1998).
Tunica Albuginea
The tunica affords great flexibility, rigidity, and tissue strength to the penis (Hsu et al, 1992) (Fig. 23–1). The tunical covering of the corpora cavernosa is a bilayered structure with multiple sublayers. Inner-layer bundles support and contain the cavernous tissue and are oriented circularly. Radiating from this inner layer are intracavernous pillars that act as struts to augment the septum and provide essential support to the erectile tissue. Outer-layer bundles are oriented longitudinally, extending from the glans penis to the proximal crura; they insert into the inferior pubic rami but are absent between the 5 and the 7 o’clock positions. In contrast, the corpus spongiosum lacks an outer layer or intracorporeal struts, ensuring a low-pressure structure during erection.
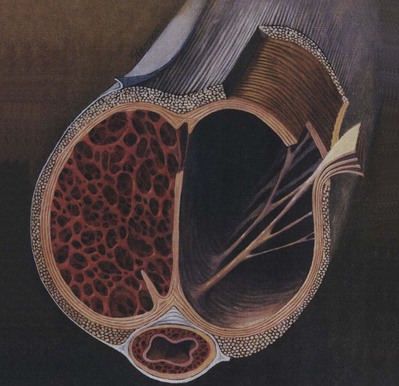
(From Lue TF, Akkus E, Kour NW. Physiology of erectile function and dysfunction. Campbell’s Urology Update 1994;12:1–10.)
The tunica is composed of elastic fibers that form an irregular, latticed network on which the collagen fibers rest (Fig. 23–2). The detailed histologic composition of the tunica varies with anatomic location and function. Emissary veins run between the inner and outer layers for a short distance, often piercing the outer bundles obliquely. However, the cavernous artery and the branches of the dorsal artery that give additional blood supply to the corpus cavernosum take a more direct route and are surrounded by a periarterial soft-tissue sheath, which protects the arteries from occlusion by the tunica albuginea during erection.
The outer tunical layer appears to play an additional role in compression of the emissary veins during erection. It also determines, to a large extent, the variability in tunical thickness and strength (Hsu et al, 1992). Between the 6 and 7 o’clock positions, the tunical thickness is 0.8 ± 0.1 mm; at the 9 o’clock position, 1.2 ± 0.2 mm; and at the 11 o’clock position, 2.2 ± 0.4 mm. At the 3, 5 to 6, and 1 o’clock positions, the measurements are nearly identical in mirror-image fashion. (Differences at specific locations have been found to be statistically significant.)
The stress on the tunica before penetration has been measured as 1.6 ± 0.2 × 107 N/m2 between the 6 and 7 o’clock positions, 3.0 ± 0.3 × 107 N/m2 at the 9 o’clock position, and 4.5 ± 0.5 × 107 N/m2 at the 11 o’clock position. The strength and thickness of the tunica correlate in a statistically significant fashion with location. The most vulnerable area is located on the ventral groove (between the 5 and 7 o’clock positions), where the longitudinal outer layer is absent; most prostheses tend to extrude here (Hsu et al, 1994).
External penile support consists of two ligamentous structures: the fundiform and suspensory ligaments. The fundiform ligament arises from Colles’ fascia and is lateral, superficial, and not adherent to the tunica albuginea of the corpora cavernosa. The suspensory ligament arises from Buck fascia and consists of two lateral bundles and one median bundle, which circumscribe the dorsal vein of the penis. Its main function is to attach the tunica albuginea of the corpora cavernosa to the pubis, and thus it provides support for the mobile portion of the penis (Hoznek et al, 1998). In patients with congenital deficiency or in whom this ligament has been severed in “penile elongation” surgery, the erect penis may be unstable or droop.
Corpora Cavernosa, Corpus Spongiosum, and Glans Penis
The corpora cavernosa are supported by a fibrous skeleton that includes the tunica albuginea, the septum, the intracavernous pillars, the intracavernous fibrous framework, and the periarterial and perineural fibrous sheath (Goldstein and Padma-Nathan, 1990; Hsu et al, 1992). Within the tunica are the interconnected sinusoids separated by smooth muscle trabeculae surrounded by elastic fibers, collagen, and loose areolar tissue. The terminal cavernous nerves and helicine arteries are intimately associated with the smooth muscle. Each corpus cavernosum is a conglomeration of sinusoids, larger in the center and smaller in the periphery. In the flaccid state, the blood slowly diffuses from the central to the peripheral sinusoids and the blood gas levels are similar to those of venous blood. During erection, the rapid entry of arterial blood to both the central and the peripheral sinusoids changes the intracavernous blood gas levels to those of arterial blood (Sattar et al, 1995).
The structure of the corpus spongiosum and glans is similar to that of the corpora cavernosa, except that the sinusoids are larger; the tunica is thinner in the spongiosum (with only a circular layer [see earlier]) and is absent in the glans (Table 23–2).
Table 23–2 Penile Components and Their Function during Penile Erection
Corpora cavernosa | Support corpus spongiosum and glans |
Tunica albuginea (of corpora cavernosa) | Contains and protects erectile tissue |
Provides rigidity of the corpora cavernosa | |
Participates in veno-occlusive mechanism | |
Smooth muscle | Regulates blood flow into and out of the sinusoids |
Ischiocavernosus muscle | Pumps blood distally to hasten erection |
Provides additional penile rigidity during rigid erection phase | |
Bulbocavernosus muscle | Compresses the bulb to help expel semen |
Corpus spongiosum | Pressurizes and constricts the urethral lumen to allow forceful expulsion of semen |
Glans | Acts as a cushion to lessen the impact of penis on female organs |
Provides sensory input to facilitate erection and enhance pleasure | |
Facilitates intromission because of its cone shape |
Arteries
The source of penile blood is usually the internal pudendal artery, a branch of the internal iliac artery (Fig. 23–3A). In many instances, however, accessory arteries exist, arising from the external iliac, obturator, and vesical and femoral arteries, and they may in some men constitute the dominant or only arterial supply to the corpus cavernosum (Breza et al., 1989). In a study of 20 fresh human cadavers, Droupy and colleagues (1997) reported three patterns of penile arterial supply: type I arising exclusively from internal pudendal arteries (3/20); type II arising from both accessory and internal pudendal arteries (14/20); and type III arising exclusively from accessory pudendal arteries (3/20). Nehra and colleagues (2008) studied 79 consecutive patients with a history of erectile dysfunction (ED) and noted that 35% had an accessory pudendal artery, typically arising from the obturator artery. In these men, the accessory pudendal was the dominant blood supply in 54% and the only corporal blood supply in 11%. The importance of accessory pudendal artery preservation during radical prostatectomy was demonstrated by Mulhall and colleagues, who reported more rapid recovery of sexual function in men who underwent artery-sparing radical prostatectomy (Mulhall et al, 2008).
The internal pudendal artery becomes the common penile artery after giving off a branch to the perineum. The three branches of the penile artery are the dorsal, bulbourethral, and cavernous. Distally, they join to form a vascular ring near the glans. The dorsal artery is responsible for engorgement of the glans during erection. The bulbourethral artery supplies the bulb and corpus spongiosum. The cavernous artery effects tumescence of the corpus cavernosum and enters it at the hilum of the penis, where the two crura merge. Along its course, it gives off many helicine arteries, which supply the trabecular erectile tissue and the sinusoids (Fig. 23–3B). These helicine arteries are contracted and tortuous in the flaccid state and become dilated and straight during erection.
Veins
The venous drainage from the three corpora originates in tiny venules leading from the peripheral sinusoids immediately beneath the tunica albuginea. These venules travel in the trabeculae between the tunica and the peripheral sinusoids to form the subtunical venous plexus before exiting as the emissary veins (Fig. 23–4A). Outside the tunica albuginea, venous drainage is as follows:
Pendulous Penis
The emissary veins from the corpus cavernosum and spongiosum drain dorsally to the deep dorsal, laterally to the circumflex, and ventrally to the periurethral veins. Beginning at the coronal sulcus, multiple venous channels coalesce to form the deep dorsal vein, which is the main venous drainage of the glans penis and distal two thirds of the corpora cavernosa. Usually a single vein, but sometimes more than one deep dorsal vein, runs upwards behind the symphysis pubis to join the periprostatic venous plexus. There are also small venous channels accompanying the paired dorsal artery. In addition, many veins also travel longitudinally within the layers of the tunica albuginea to join the dorsal vein or Santorini plexus proximally (Hsu et al, 2003). These become enlarged after the deep dorsal vein is ligated and may be the cause of recurrent leakage in venogenic ED (Chen et al, 2005).
Hemodynamics and Mechanism of Erection And Detumescence
Corpora Cavernosa
The penile erectile tissue, specifically the cavernous smooth musculature and the smooth muscles of the arteriolar and arterial walls, plays a key role in the erectile process. In the flaccid state, these smooth muscles are tonically contracted, allowing only a small amount of arterial flow for nutritional purposes. The blood partial pressure of oxygen (pO2) is about 35 mm Hg (Sattar et al, 1995). The flaccid penis is in a moderate state of contraction, as evidenced by further shrinkage in cold weather and after phenylephrine injection.
Sexual stimulation triggers release of neurotransmitters from the cavernous nerve terminals. This results in relaxation of these smooth muscles and the following events (Fig. 23–5): (1) dilation of the arterioles and arteries by increased blood flow in both the diastolic and systolic phases; (2) trapping of the incoming blood by the expanding sinusoids; (3) compression of the subtunical venous plexuses between the tunica albuginea and the peripheral sinusoids, reducing venous outflow; (4) stretching of the tunica to its capacity, which occludes the emissary veins between the inner circular and outer longitudinal layers and further decreases venous outflow to a minimum; (5) an increase in PO2 (to about 90 mm Hg) and intracavernous pressure (around 100 mm Hg), which raises the penis from the dependent position to the erect state (the full-erection phase); and (6) a further pressure increase (to several hundred millimeters of mercury) with contraction of the ischiocavernosus muscles (rigid-erection phase).
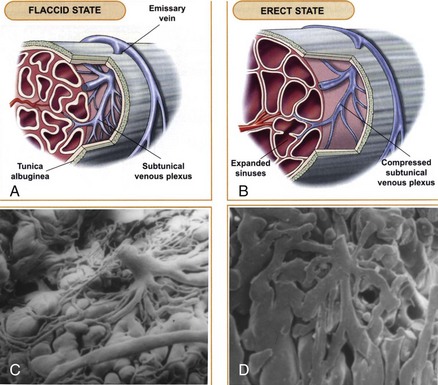
(A and B, From Lue TF, Giuliano F, Khoury S, Rosen R. Clinical manual of sexual medicine: sexual dysfunction in men. Paris: Health Publications; 2004.)
Three phases of detumescence have been reported in an animal study (Bosch et al, 1991). The first entails a transient intracorporeal pressure increase, indicating the beginning of smooth muscle contraction against a closed venous system. The second phase shows a slow pressure decrease, suggesting a slow reopening of the venous channels with resumption of the basal level of arterial flow. The third phase shows a fast pressure decrease with fully restored venous outflow capacity.
Erection thus involves sinusoidal relaxation, arterial dilation, and venous compression (Lue et al, 1983). The importance of smooth muscle relaxation has been demonstrated in animal and human studies (Saenz de Tejada et al, 1989a; Ignarro et al, 1990). To summarize the hemodynamic events of erection and detumescence, seven phases have been observed in animal experiments that reflect the changes in and the relationship between penile arterial flow and intracavernous pressure (Fig. 23–6).
Corpus Spongiosum and Glans Penis
The hemodynamics of the corpus spongiosum and glans penis are somewhat different from those of the corpora cavernosa. During erection, the arterial flow increases in a similar manner; however, the pressure in the corpus spongiosum and glans is only one-third to one-half that in the corpora cavernosa because the tunical covering, which is thin over the corpus spongiosum and virtually absent over the glans, ensures minimal venous occlusion. During the full-erection phase, partial compression of the deep dorsal and circumflex veins between Buck fascia and the engorged corpora cavernosa contributes to glanular tumescence, although the spongiosum and glans essentially function as a large arteriovenous shunt during this phase. In the rigid-erection phase, the ischiocavernosus and bulbocavernosus muscles forcefully compress the spongiosum and penile veins, resulting in further engorgement and increased pressure in the glans and spongiosum (Table 23–3).
Table 23–3 Comparison of Corpus Spongiosum and the Glans
CORPUS SPONGIOSUM | GLANS PENIS | |
---|---|---|
Tunica albuginea | Thin (circular layer only) | Absent |
Main blood supply | Bulbal and spongiosal arteries | Dorsal artery |
Venous occlusion during erection | No | No |
Compression by skeletal muscle | Yes (ischiocavernosus, bulbocavernosus) | No |
Neuroanatomy and Neurophysiology of Penile Erection
Spinal Centers and Peripheral Pathways
The innervation of the penis is both autonomic (sympathetic and parasympathetic) and somatic (sensory and motor) (Fig. 23–7). From the neurons in the spinal cord and peripheral ganglia, the sympathetic and parasympathetic nerves merge to form the cavernous nerves, which enter the corpora cavernosa and corpus spongiosum to modulate the neurovascular events during erection and detumescence. The somatic nerves are primarily responsible for sensation and the contraction of the bulbocavernosus and ischiocavernosus muscles.
Autonomic Pathways
The sympathetic pathway originates from the 11th thoracic to the 2nd lumbar spinal segments and passes through the white rami to the sympathetic chain ganglia. Some fibers then travel through the lumbar splanchnic nerves to the inferior mesenteric and superior hypogastric plexuses, from which fibers travel in the hypogastric nerves to the pelvic plexus. In humans the T10 to T12 segments are most often the origin of the sympathetic fibers, and the chain ganglia cells projecting to the penis are located in the sacral and caudal ganglia (de Groat and Booth, 1993).
The parasympathetic pathway arises from neurons in the intermediolateral cell columns of the second, third, and fourth sacral spinal cord segments. The preganglionic fibers pass in the pelvic nerves to the pelvic plexus, where they are joined by the sympathetic nerves from the superior hypogastric plexus. The cavernous nerves are branches of the pelvic plexus that innervate the penis. Other branches innervate the rectum, bladder, prostate, and sphincters. The cavernous nerves are easily damaged during radical excision of the rectum, bladder, and prostate. A clear understanding of the course of these nerves is essential to the prevention of iatrogenic ED (Walsh et al, 1990). Human cadaveric dissection has revealed medial and lateral branches of the cavernous nerves (the former accompanying the urethra and the latter piercing the urogenital diaphragm 4 to 7 mm lateral to the sphincter) and multiple communications between the cavernous and dorsal nerves (Fig. 23–8) (Paick et al, 1993). In addition to the cavernous nerve proper, pelvic ganglion cells also exist in and along the nerve components and pelvic viscera. These are seen at the bladder/prostate junction, the dorsal aspect of the seminal vesicles, and along the prostate. Takenaka and colleagues (2005) reported individual variations in distribution of these extramural ganglion cells in the male pelvis, which may complicate nerve-sparing efforts.
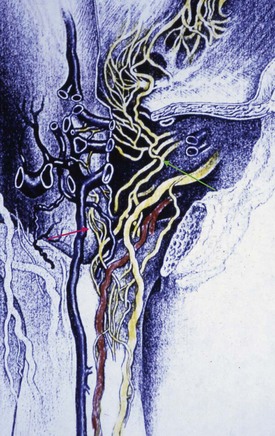
(Reprinted with permission from Paick JS, Donatucci EF, Lue TF. Anatomy of cavernous nerves distal to prostate: microdissection study in adult male cadavers. Urology 1993;42:145–9, with permission from Exerpta Medica, Inc.)
Stimulation of the pelvic plexus and the cavernous nerves induces erection, whereas stimulation of the sympathetic trunk causes detumescence. This clearly implies that the sacral parasympathetic input is responsible for tumescence and the thoracolumbar sympathetic pathway is responsible for detumescence. In experiments with cats and rats, removal of the spinal cord below L4 or L5 reportedly eliminated the reflex erectile response, but placement with a female in heat or electrical stimulation of the medial preoptic area (MPOA) produced marked erection (Giuliano et al, 1996; Sato and Christ, 2000). Paick and Lee (1994) also reported that apomorphine-induced erection is similar to psychogenic erection in the rat and can be induced by means of the thoracolumbar sympathetic pathway in case of injury to the sacral parasympathetic centers. In man, many patients with sacral spinal cord injury retain psychogenic erectile ability even though reflexogenic erection is abolished. These cerebrally elicited erections are found more frequently in patients with lower motoneuron lesions below T12 (Courtois et al, 1999); no psychogenic erection occurs in patients with lesions above T9. The efferent sympathetic outflow is thus suggested to be at the levels T11 and T12 (Chapelle et al, 1980). These authors have also reported that, in patients with psychogenic erections, lengthening and swelling of the penis are observed but rigidity is insufficient.
Somatic Pathways
The somatosensory pathway originates at the sensory receptors in the penile skin, glans, and urethra and within the corpus cavernosum. In the human glans penis are numerous afferent terminations: free nerve endings and corpuscular receptors in a ratio of 10 : 1. The free nerve endings are derived from thin myelinated Ad and unmyelinated C fibers and are unlike any other cutaneous area in the body (Halata and Munger, 1986). The nerve fibers from the receptors converge to form bundles of the dorsal nerve of the penis, which joins other nerves to become the pudendal nerve. The latter enters the spinal cord via the S2-S4 roots to terminate on spinal neurons and interneurons in the central gray region of the lumbosacral segment (McKenna, 1998). Activation of these sensory neurons sends messages of pain, temperature, and touch by means of spinothalamic and spinoreticular pathways to the thalamus and sensory cortex for sensory perception.
The dorsal nerve of the penis used to be regarded as purely somatic; however, nerve bundles testing positive for NO synthase (NOS), which is autonomic in origin, have been demonstrated in the human by Burnett and colleagues (1993) and in the rat by Carrier and colleagues (1995). Giuliano and colleagues (1993) have also shown that stimulation of the sympathetic chain at the L4-L5 level elicits an evoked discharge on the dorsal nerve and that stimulation of the dorsal nerve evokes a reflex discharge in the lumbosacral sympathetic chain of rats. These findings clearly demonstrate that the dorsal nerve has both somatic and autonomic components that enable it to regulate both erectile and ejaculatory functions.
The Onuf nucleus in the second to fourth sacral spinal segments is the center of somatomotor penile innervation. These nerves travel in the sacral nerves to the pudendal nerve to innervate the ischiocavernosus and bulbocavernosus muscles. Contraction of the ischiocavernosus muscles produces the rigid-erection phase. Rhythmic contraction of the bulbocavernosus muscle is necessary for ejaculation. In animal studies, direct innervation of the sacral spinal motoneurons by brainstem sympathetic centers (A5-catecholaminergic cell group and locus ceruleus) has been identified (Marson and McKenna, 1996). This adrenergic innervation of pudendal motoneurons may be involved in rhythmic contractions of perineal muscles during ejaculation. In addition, oxytocinergic and serotonergic innervation of lumbosacral nuclei controlling penile erection and perineal muscles in the male rat has also been demonstrated (Tang et al, 1998).
Depending on the intensity and nature of genital stimulation, several spinal reflexes can be elicited (Table 23–4). The best known is the bulbocavernosus reflex, which is the basis of genital neurologic examination and electrophysiologic latency testing. Although impairment of bulbocavernosus and ischiocavernosus muscles may impair erection, the significance of obtaining a bulbocavernosus reflex in overall sexual dysfunction assessment is controversial.
Supraspinal Pathways and Centers
Integration and processing of afferent inputs (e.g., visual, olfactory, imaginative, genital stimulation) in the supraspinal centers are essential in the initiation and maintenance of penile erection. Many brain areas have been found to be associated with sexual function including the medial amygdala, the medial preoptic area (MPOA), the paraventricular nucleus (PVN), the periaqueductal gray, and ventral tegmentum (Table 23–5). Marson and colleagues (1993) injected pseudo-rabies virus into the rat corpus cavernosum and traced labeled neurons from major pelvic ganglia to neurons in the spinal cord, brainstem, and hypothalamus. Mallick and colleagues (1994) also showed that stimulation of the dorsal nerve in the rat influenced the firing rate of about 80% of the neurons in the MPOA but not in other areas of the hypothalamus. Efferent pathways from the MPOA enter the medial forebrain bundle and the midbrain tegmental region (near the substantia nigra). Pathologic processes in these regions such as Parkinson disease or cerebrovascular accidents are often associated with erectile dysfunction. Axonal tracing in monkeys, cats, and rats has shown direct projection from hypothalamic nuclei to the lumbosacral autonomic erection centers. The neurons in these hypothalamic nuclei contain peptidergic neurotransmitters including oxytocin and vasopressin, which may be involved in penile erection (Sachs and Meisel, 1988). Several brainstem and medullary centers are also involved in sexual function. The A5-catecholamine cell group and locus ceruleus have been shown to provide adrenergic innervation to the hypothalamus, thalamus, neocortex, and spinal cord. Projections from the nucleus paragigantocellularis, which provides inhibitory serotonergic innervation, have also been demonstrated in the hypothalamus, limbic system, neocortex, and spinal cord.
Table 23–5 Brain Centers Involved in Sexual Function
LEVEL | REGION | FUNCTION |
---|---|---|
Forebrain | Medial amygdala Stria terminalis | Control sexual motivation |
Pyriform cortex | Inhibits sexual drive (hypersexuality when destroyed) | |
Hippocampus | Involved in penile erection | |
Right insula and inferior frontal cortex Left anterior cingulate cortex | Increased activity during visually evoked sexual stimulation (sexual arousal) | |
Hypothalamus | Medial preoptic area (MPOA) Lateral preoptic area (LPOA) | Ability to recognize a sexual partner, integration of hormonal and sensory cues Control nocturnal penile tumescence in rats |
Paraventricular nucleus (PVN) | Facilitates penile erection (via oxytocin neurons to lumbosacral spinal autonomic and somatic efferents) | |
Brainstem | Nucleus paragigantocellularis | Inhibits penile erection (via serotonin neurons to lumbosacral spinal neurons and interneurons) |
A5-catecholaminergic cell group Locus ceruleus | Major noradrenergic center | |
Midbrain | Periaqueductal gray | Relay center for sexually relevant stimuli |
Central Neural Activation during Arousal
In 1999 Stoléru and colleagues studied eight healthy right-handed heterosexual men with PET during visually evoked sexual arousal (Stoléru et al, 1999). Regions of brain activation were correlated with testosterone plasma levels and penile tumescence. Significant activation was seen in the bilateral inferior temporal cortices, right insula, right inferior frontal cortex, and left anterior cingulate cortex. From this landmark study a tentative model was introduced, suggesting that visually evoked sexual arousal has three components associated with neuroanatomic regions: (1) a perceptual-cognitive component that recognizes the visual stimuli as sexual and is performed in the bilateral inferior temporal cortices; (2) an emotional/motivational component that processes sensory information with motivational states and is performed in the right insula, right inferior frontal cortex, and left cingulate cortex (paralimbic areas); and (3) a physiologic component that coordinates the endocrine and autonomic functions and is performed in the left anterior cingulate cortex.
Bocher and colleagues (2001) demonstrated increased activation in the inferior lateral occipital cortex, bilateral posterior temporal cortices (right greater than left), right inferior lateral prefrontal cortex, left postcentral gyrus, bilateral inferior parietal lobules, left superior parietal lobules, frontal pole (Brodmann area 10), left prefrontal cortex, and midbrain regions. They also noted deactivation in the medial frontal and anterior cingulate, contrary to Stoleru’s report. Again, visual association centers were noted to be activated, in particular the posterior temporal cortices and postcentral gyrus. Interestingly, the midbrain activation seen in this study correlates with the location of the dopaminergic neurons. It was not demonstrated in other studies, but that may owe to these authors’ use of prolonged provocation: the visual sexual stimulus was a 30-minute continuous video clip, whereas other studies used brief visual stimuli (2 to 10 minutes).
Park and colleagues studied 12 healthy men with fMRI (Park et al, 2001) in response to erotic and nonerotic film clips. With the former, regional brain activation was generally seen in the inferior frontal lobe, cingulate gyrus, insular gyrus, corpus collosum, thalamus, caudate nucleus, globus pallidus, and inferior temporal lobes. Some activation regions were similar to those in other studies, in particular the inferior frontal lobes, inferior temporal lobes, and insular gyrus.
In a well-designed study with fMRI and visual stimuli, correlated with penile turgidity, Arnow and colleagues demonstrated a significant region of activation in the right subinsular/insular region, including the claustrum (Arnow et al, 2002), a response also seen in previous studies with PET (Stoléru et al, 1999; Redouté et al, 2000). This region has been associated with sensory processing, and this activation may represent somatosensory processing and recognition of erection. Other brain regions activated during visual sexual stimuli were the right middle gyrus, right temporal gyrus, left caudate and putamen, bilateral cingulate gyri, right sensimotor and premotor regions. Also, a lesser activation was seen in the right hypothalamus. (Dopamine is projected to the hypothalamus, and the evidence that dopamine assists male sexual behavior is substantial.) The activation of the right middle temporal gyrus is probably associated with visual processing.
In 2003 Mouras and colleagues studied eight men with fMRI. Video clips were not used; rather, still photographs (neutral and sexually arousing) were shown quickly. The authors believed that, by using shorter visual sexual stimuli, early neural responses would be generated instead of responses to the perception of penile tumescence. Again, activation of the middle and inferior occipital gyri was demonstrated, most likely linked to the visual stimuli, but not necessarily to the sexual component. In addition to multiple brain centers that showed activation with visual sexual stimuli (bilateral parietal lobules, left inferior parietal lobule, right postcentral gyrus, right parieto-occipital sulcus, left superior occipital gyrus, bilateral precentral gyrus), the cerebellum demonstrated activation in three subjects and deactivation in four. As many other reports have demonstrated activation of the cerebellum in response to erotic films and pictures of love partners (Garavan et al, 2000; Beauregard et al, 2001), visual sexual stimuli likely promote activation in regions within the cerebellum.
With the advances with fMRI, detailed comparisons of brain activation in response to visual sexual stimuli have been performed on varied groups. Stoléru and colleagues (2003) compared healthy men with men with hypoactive sexual desire disorder (HSDD) and reported that the left gyrus rectus, a portion of the medial orbitofrontal cortex, remained activated in the latter group in contrast to its deactivation in healthy men. This region is believed to mediate inhibition of motivated behavior, and its continued activation may help explain the pathophysiology of HSDD. Montorsi and colleagues (2003) compared men with psychogenic ED and potent controls after the administration of apomorphine. During visual sexual stimulation, the former group evidenced extended activation of the cingulated gyrus, frontal mesial, and frontal basal cortex, suggesting an underlying organic cause for psychogenic ED. However, their fMRI images after apomorphine were similar to those of the potent controls. Apomorphine caused additional activation of foci in the psychogenic ED patients (seen in the nucleus accumbens, hypothalamus, mesencephalon), and it was significantly greater in the right hemisphere than in the left. This greater right-sided activation is a common finding in sexually evoked brain activation studies.
Brain scanning with PET and fMRI has become a powerful tool in the study of central activation of sexual arousal, with many brain regions of activation demonstrated in these reports (Table 23–6). Psychogenic ED, premature ejaculation, sexual deviations, and orgasmic dysfunction are just a few conditions that may accompany alterations in higher brain function and perhaps now can be studied. As we begin to understand brain function with normal sexual response and arousal, the causes of dysfunction may be elucidated.
Table 23–6 Common Brain Activation Regions with Visual Sexual Stimuli*
BRAIN ACTIVATION REGIONS | FUNCTIONAL ASSOCIATION |
---|---|
Bilateral inferior temporal cortex (right > left) | Visual association area |
Right insula | Processes somatosensory information with motivational states |
Right inferior frontal cortex | Processes sensory information |
Left anterior cingulate cortex | Controls autonomic and neuroendocrine function |
Right occipital gyrus | Visual processing |
Right hypothalamus | Male copulatory behavior |
Left caudate (the striatum) | Processes attention and guides responsiveness to new environmental stimuli |
* These regions demonstrate activation with visual sexual stimuli in multiple studies.
The structures discussed earlier are responsible for the three types of erection: psychogenic, reflexogenic, and nocturnal. Psychogenic erection is a result of audiovisual stimuli or fantasy. Impulses from the brain modulate the spinal erection centers (T11-L2 and S2-S4) to activate the erectile process. Reflexogenic erection is produced by tactile stimulation of the genital organs. The impulses reach the spinal erection centers; some then follow the ascending tract, resulting in sensory perception, while others activate the autonomic nuclei to send messages via the cavernous nerves to the penis to induce erection. This type of erection is preserved in patients with upper spinal cord injury. Nocturnal erection occurs mostly during rapid-eye-movement (REM) sleep. PET scanning of humans in REM sleep shows increased activity in the pontine area, the amygdalae, and the anterior cingulate gyrus but decreased activity in the prefrontal and parietal cortex. The mechanism that triggers REM sleep is located in the pontine reticular formation; the cholinergic neurons in the lateral pontine tegmentum are activated, while the adrenergic neurons in the locus ceruleus and the serotonergic neurons in the midbrain raphe are silent. This differential activation may be responsible for these nocturnal erections. In rats, the area of the brain that appears to control nocturnal penile tumescence is the lateral preoptic area (LPOA) (Schmidt et al, 2000).
The brain centers activated during orgasm and ejaculation have also been studied. Holstege and colleagues (2003) used positron emission tomography (PET) to measure increases in regional cerebral blood flow during ejaculation versus sexual stimulation without orgasm in heterosexual male volunteers. Manual penile stimulation was performed by the volunteer’s female partner. Primary brain activation was found in the mesodiencephalic transition zone (including the ventral tegmental area), an area frequently activated with “reward” behaviors and with injection of opioids such as heroin. Other activated mesodiencephalic structures included the midbrain lateral central tegmental field, the zona incerta, the subparafascicular nucleus, and the ventroposterior, midline, and intralaminar thalamic nuclei. Increased activation was also observed in the lateral putamen and adjoining parts of the claustrum. Neocortical activity was found in Brodmann areas 7/40, 18, 21, 23, and 47, exclusively on the right side. Conversely, in the amygdala and adjacent entorhinal cortex, a decrease in activation was observed. Remarkably strong blood flow increases were observed in the cerebellum. These findings corroborate the notion that the cerebellum plays an important role in emotional processing. Although activation of these particular areas is of great interest, it is apparent that further studies are necssary to truly understanding the neurobiologic basis of orgasm, ejaculation, and sexual satisfaction in men (Table 23–7).
Table 23–7 Brain Centers of Orgasm
BRAIN AREAS | RELEVANCE | |
---|---|---|
Increased activity Primary area | Mesodiencephalic transition zone (including the ventral tegmental area) | “Reward” center also activated by opioid |
Increased activity Secondary areas | Midbrain lateral central tegmental field, the zona incerta, subparafascicular nucleus, ventroposterior, midline, and intralaminar thalamic nuclei Lateral putamen and adjoining parts of the claustrum Brodmann areas 7/40, 18, 21, 23, and 47, exclusively on the right side | |
Increased activity Other area | Cerebellum | Emotional processing |
Deceased activity | Amygdala and adjacent entorhinal cortex |
Neurotransmitters
Peripheral Neurotransmitters and Endothelium-Derived Factors
Flaccidity and Detumescence
α-Adrenergic nerve fibers and receptors have been demonstrated in the cavernous trabeculae and surrounding the cavernous arteries, and norepinephrine has generally been accepted as the principal neurotransmitter to control penile flaccidity and detumescence (Hedlund and Andersson, 1985; Diederichs et al, 1990). Receptor-binding studies have shown the number of α adrenoceptors to be 10 times higher than the number of β adrenoceptors (Levin and Wein, 1980). Currently, it is suggested that sympathetic contraction is mediated by activation of postsynaptic α1a– and α1d-adrenergic receptors (Christ et al, 1990; Traish et al, 1995) and modulated by presynaptic α2-adrenergic receptors (Saenz de Tejada et al, 1989b). Contraction mediated by α2 receptors depends on the entry of calcium from the extracellular compartment, while the activation of α1 receptors provokes the release of intracellular calcium, initially, with subsequent extracellular calcium entry for the maintenance of the contractile tone.
Endothelin, a potent vasoconstrictor produced by the endothelial cells, has also been suggested to be a mediator for detumescence (Holmquist et al, 1990; Saenz de Tejada et al, 1991a). Endothelin-1 is a member of a family of three peptides and is a potent constrictor synthesized by the sinusoidal endothelium (Holmquist et al, 1990; Saenz de Tejada et al, 1991a). Its presence in human cavernous tissue suggests the participation of this peptide in the regulation of trabecular smooth muscle. Endothelin also potentiates the constrictor effects of catecholamines on trabecular smooth muscle (Christ et al, 1995b). Two receptors for endothelin, ETA and ETB, mediate the biologic effects of endothelin in vascular tissue: ETA receptors mediate contraction, whereas ETB receptors induce relaxation.
Several constrictor prostanoids including prostaglandin I2 (PGI2), PGF2α, and thromboxane A2 (TXA2) are synthesized by the human cavernous tissue. In-vitro studies have demonstrated that prostanoids are responsible for the tone and spontaneous activity of isolated trabecular muscle (Christ et al, 1990). Functional characterization of prostanoid receptors in human trabecular and arterial penile smooth muscle has revealed that only TP receptors mediate contractile effects of prostanoids in these tissues (Angulo et al, 2002). Also, it has been observed in vitro that constrictor prostanoids, simultaneously released with NO, attenuate the dilator effect of the latter (Azadzoi et al, 1992; Minhas et al, 2001).
The renin-angiotensin system may also play a significant role in the maintenance of penile smooth muscle tone. Angiotensin II has been detected in endothelial and smooth muscle cells of human corpus cavernosum (Kifor et al, 1997) and evokes contraction of human (Becker et al, 2001a) and rabbit (Park et al, 1997) corpus cavernosum in vitro. This contractile effect is mediated by interaction with AT-I subtype receptors (Park et al, 1997). Intracavernous injection of angiotensin II reverses spontaneous erections in dogs, while the AT-I receptor antagonist, losartan, increases the intracavernous pressure (Kifor et al, 1997). Finally, intracavernous blood levels of angiotensin II, which are higher than in systemic peripheral blood, increase in the detumescence phase (Becker et al, 2001b). Thus local production of angiotensin II may increase penile smooth muscle contractility by way of AT-I receptors, assisting penile detumescence. In addition, the endothelium has been shown to release potent vasoconstrictors including endoperoxides, thromboxane A2, and superoxide anions.
The current consensus holds that the maintenance of the intracorporeal smooth muscle in a semicontracted (flaccid) state likely results from three factors: intrinsic myogenic activity (Andersson and Wagner, 1995); adrenergic neurotransmission; and endothelium-derived contracting factors such as angiotensin II, PGF2α, and endothelins. On the other hand, detumescence after erection may be a result of cessation of NO release, the breakdown of cyclic guanosine monophosphate (cGMP) by phosphodiesterases, and/or sympathetic discharge during ejaculation.
Erection
Acetylcholine has been shown to be released with electrical field stimulation of human erectile tissue (Blanco et al, 1988). Traish and colleagues (1990) reported the density of muscarinic receptors in cavernous tissue to range from 35 to 65 fmol/mg protein and in endothelial cell membrane from 5 to 10 fmol/mg protein. However, intravenous or intracavernous injection of atropine has failed to abolish erection induced in animals by electrical neurostimulation (Stief et al, 1989a) and in men by erotic stimuli (Wagner and Uhrenholdt, 1980). Although acetylcholine is not the predominant neurotransmitter, it does contribute indirectly to penile erection by presynaptic inhibition of adrenergic neurons and stimulation of NO release from endothelial cells (Saenz de Tejada et al, 1989a).
Most researchers now agree that NO released from nonadrenergic/noncholinergic (NANC) neurotransmission and from the endothelium is the principal neurotransmitter mediating penile erection. NO increases the production of cGMP, which in turn relaxes the cavernous smooth muscle (Ignarro et al, 1990; Holmquist et al, 1991; Kim N et al, 1991; Pickard et al, 1991; Burnett et al, 1992; Knispel et al, 1992; Rajfer et al, 1992; Burnett et al, 1993; Trigo-Rocha et al, 1993a). The consensus is that NO derived from nNOS in the nitrergic nerves is responsible for the initiation and majority of the smooth muscle relaxation whereby NO from eNOS contributes to the maintenance of the erection (Hurt et al, 2002). (For a fuller discussion of NO, see specific “Nitric Oxide” sections later.)
Interactions among Nerves and Neurotransmitters
Acetylcholine, by acting on the presynaptic receptors on adrenergic neurons, has been shown to modulate the release of norepinephrine (Saenz de Tejada et al, 1989b), which also can be inhibited by PGE1 (Molderings et al, 1992). In the human corpus cavernosum, noradrenergic responses are under nitrergic control. Conversely, adrenergic neurons, through prejunctional α2 receptors, can also regulate the release of NO.
Several studies have demonstrated that the interaction between the two systems also occurs in the smooth muscle (Brave et al, 1993; Angulo et al, 2001). The NO-cGMP-PKGI pathway can lead to inhibition at several sites on the noradrenergic contractile pathway in the vascular smooth muscle, impairing IP3 production by phospholipase C (Hirata et al, 1990), IP3 receptor activity (Schlossmann et al, 2000), and the RhoA/Rho-kinase pathway (Sauzeau et al, 2000). However, interaction sites have not yet been identified in penile smooth muscle. A nitrergic-noradrenergic imbalance owing to defective nitrergic neurotransmission has been implicated in penile tissue from patients and in animal models with erectile dysfunction (Christ et al, 1995a; Cellek et al, 1999). Similar to the interaction between nitrergic and noradrenergic pathways, vasoconstrictive actions of endothelin have been shown to be inhibited by NO during erection (Mills et al, 2001).
A number of factors have been reported to increase both NOS activity and NO release. These include molecular oxygen, androgen, chronic administration of L-arginine, and repeated intracavernous injection of PGE1 (Kim N et al, 1993; Escrig et al, 1999; Marin et al, 1999). Decreased NOS activity has been associated with castration, denervation, hypercholesterolemia, and diabetes mellitus. Interaction of different types of NOS may also occur. For example, nNOS activity has been to shown to decrease and iNOS levels to increase after injection of transforming growth factor (TGF)-β1 into the penis (Bivalacqua et al, 2000), and eNOS levels are reportedly significantly higher in nNOS-knockout mice (Burnett et al, 1996).
In a study of neurotransmitters in human corpus cavernosum and spongiosum, Hedlund and colleagues (2000) reported that vesicular acetylcholine transporter (VAChT), vasoactive intestinal polypeptide (VIP), and neuronal nitric oxide synthase (nNOS) are found in the same nerve terminals. Tyrosine hydroxylase (TH) positive nerves do not contain VAChT, VIP, or NOS. Heme oxygenases (HO-1 and HO-2) and eNOS are localized to the endothelium. Interaction of these neurotrasmitters may modify the effect of parasympathetic and sympathetic activation on penile function.
Role of Caveolae
Caveolae are invaginated microdomains of plasma membrane that are rich in eNOS and caveolins, as well as cholesterol, sphingolipids, and glycosylphosphatidylinositol-linked proteins. In addition, caveolae contain numerous other signaling proteins such as receptors with seven-transmembrane domains, G-proteins, adenylyl cyclase, phospholipase C, protein kinase C, calcium pumps, and calcium channels. Decreased caveolin-1 expression has been reported in the cavernous smooth muscle of aged rat (Bakircioglu et al, 2001). Linder and colleagues (2006) demonstrated that penile erection requires association of soluble guanylyl cyclase with endothelial caveolin-1 in rat corpus cavernosum. Shakirova and colleagues (2009) reported that nerve-mediated relaxation of penile tissue from caveolin-1 deficient mice was impaired. Caveolon-1 in the cavernous smooth muscle and endothelium are both decreased after bilateral cavernous nerve injury (Becher et al, 2009). These reports strongly suggest that the caveolae and caveolin are involved in the regulation of penile function.
Central Neurotransmitters and Neural Hormones
A variety of neurotransmitters (dopamine, norepinephrine,5-hydroxytestosterone [5-HT], and oxytocin) and neural hormones (oxytocin, prolactin) have been implicated in regulation of sexual function. It is suggested that dopaminergic and adrenergic receptors may promote sexual function and 5-HT receptors inhibit it (Foreman and Wernicke, 1990).
Dopamine
There are many dopaminergic systems in the brain with ultrashort, intermediate, and long axons. The cell bodies are located in the ventral tegmentum, substantia nigra, and hypothalamus. One of these dopaminergic systems, the tuberoinfundibular system, secretes dopamine (DA) into the portal hypophysial vessels to inhibit prolactin secretion (Ganong, 1999a). Five different DA receptors have been cloned (D1 to D5), and several of these exist in multiple forms (Ganong, 1999b). In men, apomorphine, which stimulates both D1 and D2 receptors, induces erection that is unaccompanied by sexual arousal (Danjou et al, 1988). In male rats, Hull and colleagues (1992) have found that low levels of dopaminergic stimulation through the D1 receptor increase erection; higher levels or prolonged stimulation produces seminal emission through D2 receptors. The erectile response induced by injection of apomorphine into the paraventricular area can be suppressed by blockers of both DA and oxytocin receptors (Melis et al, 1989). Injection of oxytocin into the paraventricular area also induces erection, but this cannot be blocked by DA receptor blockers. These findings suggest that dopaminergic neurons activate oxytocinergic neurons in the paraventricular area and that the release of oxytocin produces erection (Melis et al, 1992).
In general terms, DA is supportive of copulation and 5-HT is inhibitory. DA is released in the MPOA at the time of ejaculation (5-HT is not), and changes in DA and 5-HT in different areas of the brain may promote copulation and sexual satiety, respectively (Hull et al, 1999). Testosterone enhances DA release in the MPOA at rest and with sexual challenge, possibly by upregulating NOS, which increases NO and thereby increases DA release. The same pattern of copulatory activity promoting DA release in the MPOA and the enhanced effect of the presence of sex hormones is seen in female rats. Longer-lasting changes may be seen through the postcopulatory effects of gene expression, and this expression increases with increased sexual experience, effectively changing the phenotype of certain cells in sexually experienced animals. Cells of the MPOA have high densities of α2-noradrenergic receptors, as well as DA receptors, and the effects of DA in the MPOA are most likely assisted by the activation of α2 (inhibition) and α1 (excitation) adrenoceptors owing to cross-talk within central nervous system (CNS) catecholamine systems (Cornil et al, 2002).
DA agonists (apomorphine and pergolide) and DA uptake inhibitors (nomifensine and bupropion) have been reported to enhance sexual drive (Stimmel and Gutierrez, 2006). Sublingual apomorphine is available for the treatment of ED in many countries but its utility is limited due to emetic side effects. A selective DA D4 receptor agonist, ABT-724, has been shown to assist penile erection in a dose-dependent fashion in conscious rats; interestingly, this drug lacked emetic effects in a ferret model (Brioni et al, 2004; Osinski et al, 2005). A similar compound, ABT-670, with superior oral bioavailability has also been identified for potential clinical use (Patel et al, 2006).
Serotonin
Neurons containing 5-HT have their cell bodies in the midline raphe nuclei of the brainstem and project to a portion of the hypothalamus, limbic system, neocortex, and spinal cord (Ganong, 1999a). Currently, 5-HT receptors 1 to 7 have been cloned and characterized. Within the 5-HT1 group are the 5-HT1 A, B, D, E, and F subtypes. Within the 5-HT2 group are the 5-HT2 A, B, and C subtypes. There are two 5-HT5 subtypes, 5-HT5 A and B (Ganong, 1999b). General pharmacologic data indicate that 5-HT pathways inhibit copulation, but 5-HT may have both facilitory and inhibitory effects on sexual function, depending on the receptor subtype, the receptor location, and the species investigated (de Groat and Booth, 1993). Andersson and Wagner (1995) have summarized the results of administration of selective agonists and antagonists as follows: 5-HT1A receptor agonists inhibit erectile activity but assist ejaculation; stimulation of 5-HT2C receptors causes erection; and 5-HT2 agonists inhibit erection but assist seminal emission and ejaculation. Steers and de Groat (1989) have also shown increased firing of the cavernous nerve and erection when m-chlorophenylpiperazine, a 5-HT2C receptor agonist, is given to rats. Applying a novel 5-HT2c receptor agonist (YM348) and antagonist SB242084, Kimura and colleagues (2006) confirmed the pro-erectile effect of the 5-HT2c receptor stimulation in rats. Stimulation of both 5-HT2 and 5-HT2C receptors has also been reported to increase oxytocin secretion (Bagdy et al, 1992). In addition, 5-HT may also affect the spinal reflex because Marson and McKenna (1992) have reported that endogenous 5-HT may act in the lumbar cord to inhibit sexual reflexes.
5-HT is believed to be an inhibitory transmitter in the control of sexual drive (Foreman et al, 1989). Suppressed libido has been reported in patients taking fenfluramine, a 5-HT-releasing agent, but elevated libido occurred in patients taking buspirone, a 5-HT neuron suppressor (Buffum, 1982).
Norepinephrine
The cell bodies of the norepinephrine-containing neurons are located in the locus ceruleus and the A5-catecholaminergic cell group in the pons and medulla. The axons of these noradrenergic neurons ascend to innervate the paraventricular, supraoptic, and periventricular nuclei of the hypothalamus, the thalamus, and neocortex. They also descend into the spinal cord and the cerebellum. Central norepinephrine transmission seems to have a positive effect on sexual function. In both humans and rats, inhibition of norepinephrine release by clonidine, an α2-adrenergic agonist, is associated with a decrease in sexual behavior, and yohimbine, an α2-receptor antagonist, has been shown to increase sexual activity (Clark et al, 1985). β Blockers have also been implicated in sexual dysfunction, probably because of their central side effects such as sedation, sleep disturbances, and depression.
γ-Aminobutyric Acid
γ-Aminobutyric acid (GABA) activity in the PVN provides a mechanism to balance (inhibit) proerectile signaling. Agonism for the type A receptor for GABA in the PVN can reduce both pharmacologically (apomorphine) and physiologically induced erections (Melis et al, 2001).
Opioids
Endogenous opioids are known to affect sexual function, but the mechanism of action is far from clear. Injection of small amounts of morphine into the MPOA assists sexual behavior in rats. Larger doses, however, inhibit both penile erection and yawning induced by oxytocin or apomorphine. It is suggested that endogenous opioids may exert an inhibitory control on central oxytocinergic transmission (Argiolas, 1992). Injection of morphine into the paraventricular nucleus of the hypothalamus prevents noncontact penile erections and impairs copulation in rats. It is speculated that intracellular nitric oxide may be involved in this process (Melis et al, 1999).
Cannabinoids
Cannabinoid CB1 receptor activation inhibits sexual function by modulating the paraventricular oxytocinergic neurons, which mediate erection. Antagonism of CB1 receptors in the paraventricular nucleus of male rats induces penile erection, which seems to involve glutamic acid and nitric oxide (Melis et al, 2004, 2006).
Oxytocin
Oxytocin is a neural hormone secreted by the neurons into the circulation. These are found in the posterior pituitary gland, but, because they are also found in the neurons projecting from the PVN to the brainstem and spinal cord, oxytocin can also function as a neurotransmitter. The blood level is increased during sexual activity in humans and animals and, when injected into the paraventricular area in rats, oxytocin produces yawning and penile erection. Calcium has been suggested as the second messenger mediating oxytocin-induced penile erection. Oxytocin release following stimulation of dompamine receptors in the paraventricular nucleus influences the appetitive and reinforcing effects of sexual activity (Succu et al, 2007). Because neurons in the paraventricular area have been shown to contain NOS, and NOS inhibitors prevent apomorphine- and oxytocin-induced erection, it is suggested that oxytocin acts on neurons whose activity is dependent on certain levels of NO (Vincent and Kimura, 1992; Melis and Argiolas, 1993).
Nitric Oxide
Nitric oxide (NO) is a gaseous molecule produced from various tissues, in particular the endothelium and nerve. It mediates penile erection at the level of the PVN (Melis et al, 1998) and at other levels of the neural pathway supporting sexual response. The presence of NO and the soluble guanylyl cyclase needed to generate cGMP is seen throughout the human brain. The NO/cGMP pathway (see later) is affected by aging in the brain and offers a potentially significant but unexplored site for mediating the deleterious effects of age on sexual function (Ibarra et al, 2001). Reduced nNOS protein within the PVN leading to blunting of the erectile response has been reported in streptoxotosin-induced diabetic rats (Zheng et al, 2007). Testosterone or its metabolite dihydrotestosterone (DHT) downregulates NOS activity and mRNA expression and the number of nNOS-containing neurons (Singh et al, 2000). Direct evidence of the importance of NO in central signaling related to erectile function resulted from a series of experiments designed to alter CNS NO activity (Sato et al, 2001): Manipulation of NO or cGMP levels altered MPOA-triggered intracavernous pressure response through CNS, not peripheral, mechanisms.
