Physiology of Lower Urinary Tract—Bladder and Urethra
Toby C. Chai
Gopal N. Gupta
INTRODUCTION
Normal bladder function is typified by the storage of an adequate volume of urine at low pressure without leakage and unwanted bladder sensations (urgency) interspersed with periods of efficient unimpeded expulsion of urine. The bladder and bladder outlet (internal and external urethral sphincters) are under tightly regulated neural control. This allows for normal urinary storage and expulsion. The bladder and internal urethral sphincter are composed of smooth muscle fibers, while the external urethral sphincter is composed of skeletal striated muscle fibers. While we have learned much of bladder neurophysiology from studying animal models, the actual pathologic defects in bladder dysfunction frequently encountered clinically, such as urinary incontinence (both stress and urge), nonneurogenic detrusor overactivity, hypersensitive bladder syndromes (e.g., overactive bladder and interstitial cystitis), and nonobstructive urinary retention remain elusive. Animal models have helped test etiologic theories and define treatment modalities for disorders of micturition and urinary continence. This chapter will focus on data obtained from experiments performed with human tissues if available.
There is less published literature about neurophysiologic control of the urethra compared with that of the bladder. Additionally, the functional framework of the urethra is opposite to that of the bladder: during urine storage, the urethra is contracted while the bladder is relaxed, and during expulsion, the urethra is relaxed while the bladder is contracted. Therefore, treatments designed to reverse bladder dysfunction would mandate opposite effects on the urethra.
The bladder is unique among autonomically innervated organs due to the high degree of conscious or voluntary control that can be exerted on its function. This means that well-established neural connections from higher neural centers (e.g., cerebral cortex) to the bladder exist. Therefore, the neural control of bladder function is quite complex and a neurophysiologic defect anywhere from the cerebral cortex to the bladder can result in bladder dysfunction. This complexity in part explains our limited understanding of the pathophysiology of bladder dysfunction and also explains the limited treatments available for these bladder problems. The intent of this chapter is not to provide an exhaustive review of the basic science literature, but rather to present neurophysiological facts that have clinical relevance. Literature from experimental studies utilizing humans and/or human bladder tissues will be emphasized for maximal clinical relevance.
BLADDER EFFERENT PATHWAY
Peripheral Efferent Neural Pathways
The motor pathway to the bladder is autonomic. The bladder efferent neuronal bodies originate from the S2-S4 spinal cord within the sacral parasympathetic nucleus, which is situated between the ventral and dorsal horn gray matter. The preganglionic axons exit the spinal cord in the ventral roots and merge into the periphery in the pelvic nerves. These pelvic nerves synapse at ganglia within the periphery at the pelvic plexus or even within the bladder wall (intravesical ganglia) (1,2). The postganglionic nerves then synapse onto the bladder smooth muscle cells (Fig. 4.1). The
end effect of activation of the efferent pathway is a coordinated, efficient bladder contraction that results in bladder emptying.
end effect of activation of the efferent pathway is a coordinated, efficient bladder contraction that results in bladder emptying.
The sympathetic motor neuronal bodies reside in the thoracolumbar spinal cord. The preganglionic motor fibers also exit the spinal cord within the ventral nerve roots. The preganglionic fibers synapse on the postganglionic nerve at the paravertebral ganglia, which lie close to the spinal cord. The postganglionic nerve becomes the hypogastric nerve following closely to the hypogastric artery to innervate the bladder.
The role of sympathetic motor innervation in normal bladder function is not totally clear in the human. Studies in the cat suggest that it has a role in maintenance of urinary continence. Activation of sympathetic outflow to the bladder via stimulation of bladder afferents in anesthetized cats caused the bladder to relax and the urethra to contract (3,4). This pathway may have importance in maintaining continence in humans. The human bladder smooth muscle has been found to have β3-adrenergic receptor (β3-AR), which may mediate its relaxation (5). The relaxation of the bladder may be through inhibition of the parasympathetic intravesical ganglia or through activation of β3-AR on the bladder. Therefore, the concept of using a β3-AR agonist for detrusor overactivity is actively being investigated.
The motor pathway to the bladder does not have to be thought of as “isolated” from the afferent pathway (detailed later). Recent data has shown that “micromotions” of the bladder can be detected in humans with sensory urgency (overactive bladder syndrome) (6). These micromotions are presumed to be small localized contractions of the detrusor muscle that cannot be detected with conventional water-filled cystometrography. It is presumed that these bladder micromotions within the smooth muscle can disturb nearby sensory fibers, giving rise to a sense of urinary urgency. Patients with overactive bladder syndrome had much higher frequencies of these detrusor micromotions (6). Therefore, there can be a relationship
between detrusor overactivity (in this case micromotions) and increased sensory awareness of the bladder. It remains to be seen how clinically useful micromotion detection will become and whether this is truly related to overactive bladder syndrome.
between detrusor overactivity (in this case micromotions) and increased sensory awareness of the bladder. It remains to be seen how clinically useful micromotion detection will become and whether this is truly related to overactive bladder syndrome.
Detrusor Smooth Muscle Signaling
The neurotransmitter released by the preganglionic and postganglionic parasympathetic nerves is acetylcholine. Acetylcholine released by the postganglionic cells binds to muscarinic receptors (M2 and M3) on the bladder smooth muscle cells to initiate the excitation-contraction event. Bladder smooth muscle contraction mechanisms have been extensively studied because it is easy to obtain smooth muscle from animal models, both in normal and experimentally induced diseased states (e.g., bladder outlet obstruction, diabetes, inflammation models). One must remember that these are in vitro studies in which the bladder has been typically stripped of the urothelium and neural input; thus, the findings from organ baths do not necessarily reflect the complete in vivo picture.
The normal human bladder is composed of 70% M2 receptors and 30% M3 receptors. It is actually the M3 receptors that are responsible for organ bath-measured contractions (7,8). However, M2 receptors mediate organ bath contractions in spinal cord-injured humans (9). It was also shown that mRNA for M3 receptor decreased as a function of aging in the human bladder (10). This could possibly explain the decreased contractility of the bladder in the elderly. Another study suggested that unique variations in expression of M2 and M3 receptors could explain variable responses to antimuscarinics (11).
Most treatments for patients with urinary urgency, frequency, and urge incontinence (overactive bladder) are aimed at blockade of presumed “uninhibited” bladder smooth muscle contractions mediated by either M2 or M3 receptors. This is based on the supposed mechanism of action of antimuscarinic agents. Whether these presumed pathologic detrusor smooth muscle contractions are pathophysiologic, whether there is an alteration in muscarinic receptor phenotype distribution (M2:M3 ratio), and whether the primary pathology in overactive bladder is in the motor efferent pathway are currently unknown. It is unclear whether the physiologic defect in overactive bladder lies completely in the bladder motor pathway or may involve the sensory pathway. Urinary urgency would seem to involve a component of sensory-initiated phenomenon. Nevertheless, the efficacy of antimuscarinics for treatment of overactive bladder symptoms has been shown in multiple large clinical trials (12).
The trigger for smooth muscle contraction is the increase in intracellular cytosolic calcium. Muscarinic receptor activation initiates this through cascades of secondary messenger events. The prototypical smooth muscle contraction signaling by increased cytosolic calcium is best explained by M3-receptor activation. M3 activation results in activation of phospholipase C, which hydrolyzes phosphoinositide-4,5-biphosphate, with subsequent release of inositol triphosphate (IP3) and diacylgycerol (13). Diacylgycerol activates protein kinase C, which can increase cytosolic calcium through release of intracellular stores, while IP3 activates the release of calcium from the sarcoplasmic reticulum. These events ultimately result in increased cytosolic calcium. Surprisingly, this prototypical sequence of events of phospholipase C activation and IP3 and diacylgycerol release was not critical to M3-mediated human detrusor smooth muscle contraction (14). This demonstrates that there are species-specific differences in secondary messenger events even if M3-receptor activation is the upstream event. The contractions in human detrusor smooth muscle were largely mediated by calcium influx through L-type, voltage-dependent channels (nifedipine-sensitive channels). These mechanisms are depicted in Figure 4.2.
Although increased cytosolic calcium is still the ultimate event (15,16), the mechanism by which activated M2 receptors mediate detrusor smooth muscle contraction is less direct than the mechanism occurring with activated M3 receptors. Because human bladders obtained from spinal cord-injured patients have M2-mediated contractions (9), it is critical to understand the downstream events after M2-receptor activation. The activated M2 receptor interacts with the Gi protein, which then inhibits adenylyl cyclase, resulting in decreased cytosolic cAMP. cAMP ultimately regulates intracellular calcium. If cAMP is decreased by M2-receptor activation, cytosolic calcium will be increased, leading to contraction. In addition, adenylyl cyclase is the prototypical enzyme that is activated by activation of the β-adrenergic receptor, with resultant increased cAMP and smooth muscle relaxation. In this way, M2-receptor activation could induce smooth muscle contraction by counteracting sympathetic effects on detrusor smooth muscle. These mechanisms are depicted in Figure 4.2.
Receptors other than M3 may also mediate bladder contractions. This was based on findings
that bladder contractions in animals were resistant to both cholinergic and adrenergic blockade (termed “nonadrenergic, noncholinergic” [NANC]-mediated bladder contractions) (17). For example, in humans it was shown that the purinergic agent adenosine triphosphate (ATP) can cause bladder smooth muscle contractions (18). These ATP-mediated contractions occur through the P2X1 receptors on the bladder smooth muscle (19). It is possible that in disease states bladder contractions could shift from muscarinic- to purinergic-mediated mechanisms. In humans, it has been shown that bladder smooth muscle strips obtained from patients with interstitial cystitis are much more responsive to ATP compared to control human bladder strips (20). Also, in aging, the human detrusor has an increased purinergic trigger as the basis for its contractions (21).
that bladder contractions in animals were resistant to both cholinergic and adrenergic blockade (termed “nonadrenergic, noncholinergic” [NANC]-mediated bladder contractions) (17). For example, in humans it was shown that the purinergic agent adenosine triphosphate (ATP) can cause bladder smooth muscle contractions (18). These ATP-mediated contractions occur through the P2X1 receptors on the bladder smooth muscle (19). It is possible that in disease states bladder contractions could shift from muscarinic- to purinergic-mediated mechanisms. In humans, it has been shown that bladder smooth muscle strips obtained from patients with interstitial cystitis are much more responsive to ATP compared to control human bladder strips (20). Also, in aging, the human detrusor has an increased purinergic trigger as the basis for its contractions (21).
Detrusor smooth muscle contraction is triggered by increased cytosolic calcium. Calcium binds to calmodulin and causes a conformal change in calmodulin, exposing sites that interact with myosin light-chain kinase (MLCK). MLCK activation phosphorylates MLC protein, which results in cycling of myosin crossbridges (heads) along actin filaments and the development of tension. Furthermore, phosphorylation of MLC also activates myosin ATPase, which hydrolyzes ATP to provide energy for detrusor smooth muscle contraction. A secondary mechanism that is calcium independent can also occur via the rho-rho-kinase pathway. This pathway is dependent on inhibition of MLC dephosphorylation (via inhibition of myosin phosphatase) (22). The rho-rho-kinase pathway has been shown to mediate human detrusor smooth muscle contractions (14,23). The rho-rho-kinase pathway may represent another therapeutic target, assuming it either is pathologically altered in disease or can be harnessed to compensate for defects in the calcium-dependent excitation-coupling pathway.
A clinical condition that seems to be directly related to the efferent system is that of idiopathic nonobstructive urinary retention. Patients present in urinary retention without an identifiable bladder outlet obstruction. The etiology of the retention is presumed to be the inability of the detrusor smooth muscle to generate a contraction. However, treatment with oral urecholine, a muscarinic agonist, has not proven to be uniformly clinically useful, even though the first description of its use was over half a century ago (24). The failure of the smooth muscle to contract may be caused by a variety of reasons related to the neurophysiology discussed above, including failure of the efferent nerves to release acetylcholine, failure of urethra
or bladder outlet to relax, and/or failure of excitation-contraction coupling at any point along the pathway from muscarinic receptor activation to force generation. A synopsis of the complexities of smooth muscle function in the lower urinary tract has been published (25).
or bladder outlet to relax, and/or failure of excitation-contraction coupling at any point along the pathway from muscarinic receptor activation to force generation. A synopsis of the complexities of smooth muscle function in the lower urinary tract has been published (25).
Descending Efferent Neural Pathways
Descending neurons from the medullary pons (pontine micturition center [PMC], Barrington’s nucleus, or M-region) synapse on the sacral parasympathetic nucleus to modulate efferent outflow to the bladder. In cats this pathway has been demonstrated (26), and stimulation of this area caused the bladder to contract and the urethra to relax (27). Since the PMC is under volitional cortical control, the micturitional reflexes can be consciously suppressed (see Fig. 4.1). These descending pathways respond to adrenergic receptor agonists/antagonists (28,29) in modulating the micturition reflex. Intrathecal adrenergic agonists augment the micturition reflex (promote bladder emptying), whereas adrenergic antagonists inhibit the micturition reflex (promote bladder storage).
In the context of alpha-blocker use in treatment of benign prostatic hyperplasia (BPH) and lower urinary tract symptoms (LUTS) such as urinary frequency and urgency, these findings are relevant and interesting. Traditionally, the mechanism of action of alpha-blockers in the treatment of BPH has been thought to be relaxation of prostatic smooth muscle, with subsequent decreased “dynamic” tone of the prostatic urethra (30). Another effect on the prostate may be apoptosis of prostatic glandular cells (31). However, the therapeutic effect of alpha-blockers in decreasing urinary frequency and urgency may also target the central descending efferent pathways onto the sacral spinal cord and thereby inhibit the micturition reflex. Furthermore, elevated catecholamine states such as hypertension have been shown to be associated with increased LUTS in both animals and humans (32,33). These findings suggest that the beneficial actions of alpha-blockers in LUTS may be more complex than previously thought.
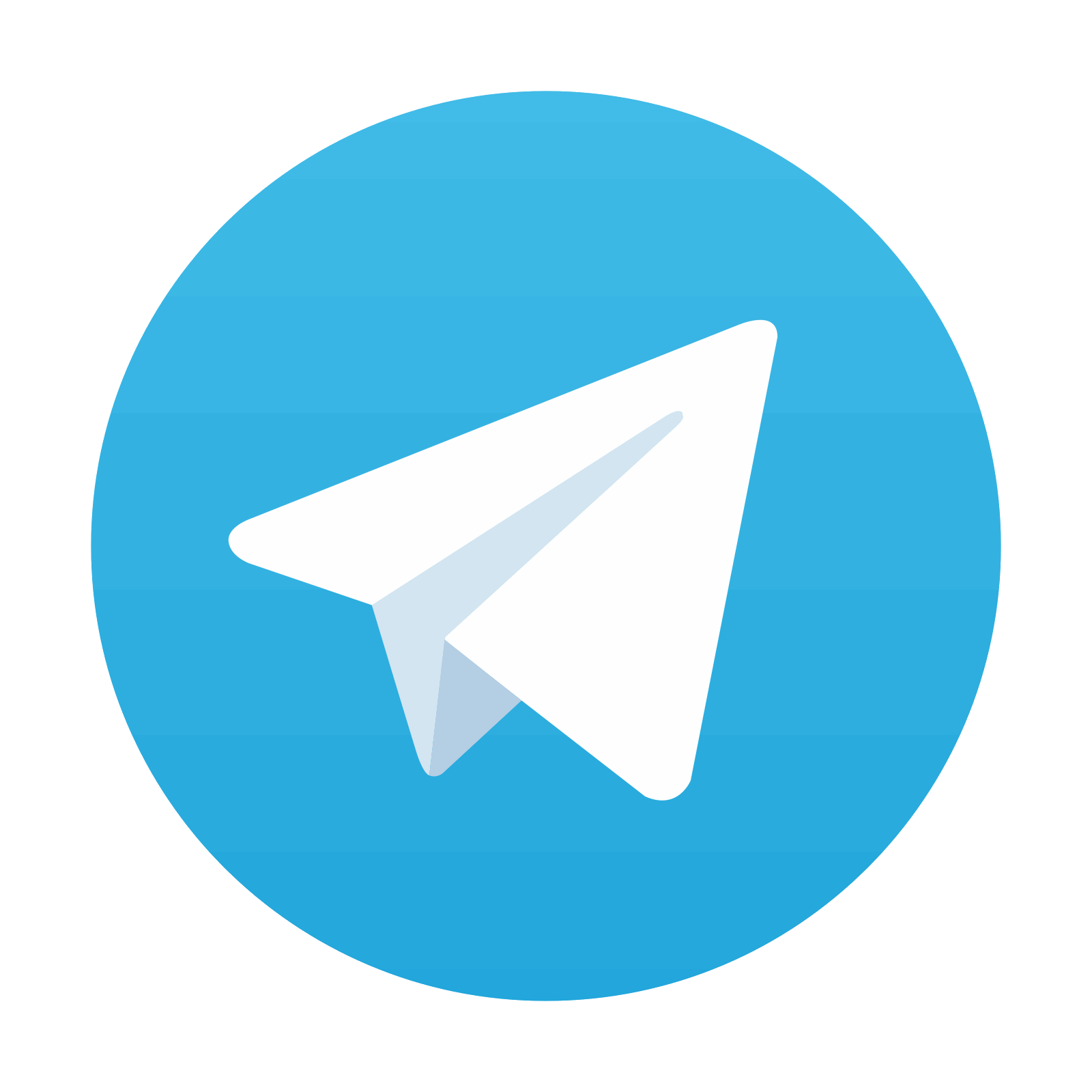
Stay updated, free articles. Join our Telegram channel

Full access? Get Clinical Tree
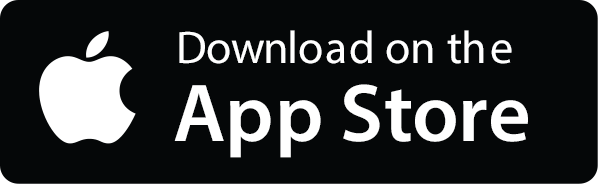
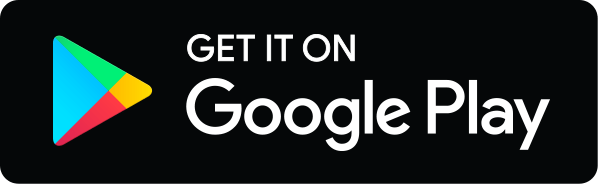