This chapter reviews the molecular physiology of the NaCl co-transporters, with emphasis on the thick ascending limb of Henle’s loop Na + -Cl − co-transporter and the distal convoluted tubule Na + -Cl − co-transporter that serve as the receptors for the loop diuretics and thiazide-type diuretics, respectively. Inactivating mutations of these co-transporters produces the hypokalemic metabolic alkalosis syndromes known as Bartter’s and Gitelman’s disease, respectively, and their altered regulation by mutant kinases is associated with a salt-sensitive form of human hypertension. Thus, these proteins are potentially involved in complex disease such as essential hypertension.
Keywords
sodium; potassium; chloride; pore; ion channel; carrier; pump; diffusion; electrodiffusion
Introduction
There are four types of electroneutral co-transporter systems that have been identified: (1) the sulfamoylbenzoic (or bumetanide)-sensitive Na + -K + -2Cl − co-transporter; (2) the sulfamoylbenzoic (or bumetanide)-sensitive Na + -Cl − co-transporter; (3) the benzothiadiazine (or thiazide)-sensitive Na + -Cl − co-transporter; and (4) the dihydroindenyloxy-alkanoic acid (DIOA)-sensitive K + -Cl − co-transporter. All these possibilities are encoded by members of the family of solute carriers SLC12, according to the classification of the Human Genome Organization. Two genes of this family, SLC12A1 encoding the apical Na + :K + :2Cl − co-transporter, NKCC2 (also known as BSC1), and SLC12A3, encoding the Na + :Cl − co-transporter, NCC (also known as TSC1), are particularly relevant to kidney physiology, pharmacology, and pathophysiology. NKCC2 and NCC play a key role in salt reabsorption of the thick ascending limb of Henle’s loop and distal convoluted tubule, respectively, with consequent effects in potassium, calcium, and acid–base homeostasis. They also serve as receptors for the loop diuretics (furosemide, bumetanide, ethacrinic acid) and thiazide-type diuretics (chlortalidone, hydrochlorothiazide, metolazone), respectively, that are heavily used in the management of patients with arterial hypertension or edematous states, such a cardiac failure, chronic liver disease, chronic renal disease or nephrotic syndrome. Inactivating mutations of NKCC2 cause Bartter syndrome type I, and of NCC cause Gitelman’s syndrome. Additionally, dysregulation of NCC is implicated in the genesis of pseudohypoaldosteronism type II, and it is proposed that both co-transporters belong to the hypertension susceptibility genes. In this chapter we will discuss the cation-coupled chloride co-transporters, with particular emphasis on NKCC2 and NCC.
The SLC12A family was identified in the early 1990s with the cloning of the thiazide-sensitive Na + -Cl − co-transporter, followed by two genes encoding the Na + -K + -2Cl − co-transporters, and is composed of nine related genes. Later, the four genes encoding K + :Cl − co-tranporters that were named KCC1, KCC2, KCC3, and KCC4 were identified.
Figure 32.1 depicts a phylogenetic tree of all members of the SLC12 family for which functional properties are known, as well as the chromosome to which each gene has been mapped in humans, the inherited disease that results from inactivating mutations of the gene, and the consequence of knocking-out each gene in the mouse. Two branches within the family are clearly identified. The Na + -driven branch encompasses three genes: SLC12A1 and SLC12A2 encode the Na + :K + :2Cl − co-transporters, NKCC2 and NKCC1, respectively. NKCC2 is a kidney-specific gene, with expression restricted to the apical membrane of the thick ascending limb of Henle’s loop (TAL). In contrast, NKCC1 is a ubiquitously expressed protein that is located at the basolateral membrane of secretory epithelial cells, as well as in many non-epithelial cells (neurons, fibroblasts, erythrocytes, etc.). SCL12A3 is the third gene of the Na + -driven branch, and encodes the thiazide-sensitive Na + :Cl − co-transporter, NCC. It is mainly expressed in the apical membrane of the distal convoluted tubule (DCT). Initially it was thought that NCC is a gene with restrictive expression in the kidney; however, later it was demonstrated to be expressed at the protein level along the gut, in bone, and in the lens. Although a thiazide-sensitive Na + -Cl − co-transporter has been postulated to exist in other tissues, blood vessels, pancreas, peripheral blood mononuclear cells, gallbladder and heart, its presence at the molecular level has not been demonstrated. In bone, NCC activity is associated with the rate of bone formation. Many clinical studies have shown that thiazide diuretics in elderly subjects promote an increase in bone mineral density and help to prevent pathological fractures. Consistent with this beneficial effect of thiazides, NCC is expressed in osteoblasts of rat and human bones, and addition of thiazides to osteoblasts in culture increases the formation of mineralized nodules. This effect of thiazides was not present after NCC expression was reduced by transfecting cells with an NCC antisense plasmid.

The degree of identity at the protein level between NKCC1 and NKCC2 is ~60%, and between NCC and the NKCCs is ~50%. The degree varies, however, within specific domains of the co-transporters. It is >80% in some of the central hydrophobic membrane spanning domains, ~50% in the carboxyl terminal domain, and <10% in the amino terminal domain and most of the interconnecting loops that are oriented toward the extracellular side.
The K + -driven branch is composed of four genes, SLC12A4 to SLC12A7, encoding the K + :Cl − co-transporters KCC1 to KCC4, respectively. KCC1 is ubiquitously expressed, while KCC2 is only present in neurons. KCC3 and KCC4 are expressed in several tissues, including the kidney. Along the nephron KCC3 has been shown to be present only in the proximal tubule, while KCC4 is also expressed in the basolateral membrane of TAL and the intercalated cells of collecting duct (CD). The degree of identity is about 60% between KCCs and about 25% with the members of the Na + -driven branch.
Two additional genes are classified within the SLC12A family (not shown in Figure 32.1 ) due to a degree of identity of ~20% with either the Na + -driven or the K + -driven branches. SLC12A8 gene (human chromosome 3) encodes a protein of 714 amino acid residues that has been identified as a psoriasis susceptibility gene by two independent groups. One report suggests that this protein may translocate polyamines and amino acids across the plasma membrane. SLC12A9 (human chromosome 7) encodes a 918 residue protein originally named as co-transporter interacting protein (CIP) for its ability to modulate the activity of NKCC1. Its topological similarity and the 25% identity with other members of the family suggest that it is likely that CIP transports substrates that have not been identified.
The Physiology of NaCl Co-Transporters in the Kidney
The electroneutral cation–chloride co-transporters translocate Cl − ions together with a cation, which can be Na + , K + or both, maintaining the requirement of electroneutrality: 1Na + -1Cl − , or 1K + -1Cl − or 1Na + -1K + -2Cl − stoichiometry. The direction of the transport process is defined by the cation gradient. Therefore, NKCC1, NKCC2, and NCC move ions inward across the plasma membrane, while KCCs move ions outward. Because Na + and K + are returned to the steady-state by the Na + :K + -ATPase, the activity of the SLC12A co-transporters is considered to be primarily regulation of the intracellular chloride concentration [Cl − ] i , a role that is critical to some physiological processes. One of these is cell volume regulation. During cell shrinking, due to increased osmolarity of the extracellular medium, the regulatory volume increase response stimulates transport mechanisms to enhance the intracellular osmolarity, including activation of NKCC1 > NKCC2 > NCC. In contrast, during cell swelling, the regulatory volume decrease promotes the activation of KCCs to reduce intracellular osmolarity. Because basically every cell expresses NKCC1 and KCC1, this cell volume regulatory mechanism is universal.
Another major function of electroneutral cation–chloride co-transporters is the setting of the intraneuronal chloride concentration, either above or below its electrochemical equilibrium potential. For this reason, the activity of these co-transporters is critical in determining the polarity and magnitude of the effect of neurotransmitters that gate Cl − channels in postsynaptic membranes, such as GABA. It is known that before birth GABA behaves mostly as an excitatory neurotransmitter, in neurons in which intracellular chloride is above the electrochemical equilibrium due to more prominent expression of NKCC1 than KCC2. After birth, however, GABA becomes predominantly an inhibitor transmitter, due to the inversion of NKCC1/KCC2 ratio of expression that lowers intracellular chloride below equilibrium.
A third major role of the SLC12A co-transporters that will occupy our interest for the rest of this chapter is the transepithelial movement of ions. NCC and NKCC2 are polarized to the apical membrane of DCT and TAL, respectively, where they participate in renal salt reabsorption. NKCC1 is expressed in the basolateral membrane of several chloride-secreting epithelia, where its activity is critical to provide the cell with chloride ions to be secreted in the apical membrane. The K + :Cl − co-transporters are also involved in epithelial movement of ions. One example is KCC4 that is expressed in the intercalated cells of the CD, where it plays a role in the chloride efflux required to maintain hydrogen secretion and thus, acid–base homeostasis.
The Thiazide-Sensitive Na − -Cl − Co-Transporter
NCC is the major salt transport pathway in the apical membrane of mammalian DCT cells which mediates reabsorption of 5–10% of glomerular filtrate. In the early DCT (DCT1) NCC is fully in charge of salt reabsorption, while in the late DCT (DCT2) it shares the responsibility with the sodium channel ENaC. This is an important difference, since DCT1 is not considered to be part of the aldosterone-sensitive distal nephron, due to the lack of 11β-hydroxysteroid dehydrogenase type 2 (11β-HSD2), which prevents illicit occupation of the mineralocorticoid receptor by cortisol. Thus, NCC is susceptible to aldosterone regulation only in DCT2 cells. The molecular mechanism of salt reabsorption in DCT is illustrated in Figure 32.2 . The Na + gradient that drives transport from the lumen to the interstitium is generated and maintained by the intense activity of Na + /K + -ATPase that is polarized to the basolateral membrane. Potassium entering the cell through the Na + /K + pump is secreted by the luminal membrane via K + channels and by an apical K + -Cl − co-transporter. Thus, potassium secretion rate is determined, at least in part, by the rate of Na + -Cl − reabsorption. In addition, NCC modulates magnesium and calcium reabsorption, the latter in an inverse relationship with sodium reabsorption. The lower the sodium reabsorption, the higher the calcium reabsorption, and vice versa . As shown in Figure 32.2 , NCC is the target for thiazide-type diuretics (metolazone, hydrochlorothiazide, chlortalidone, bendroflumethiazide). Because thiazides are recommended for the treatment of arterial hypertension, some edematous states, and renal stone disease, this group of drugs are among the most commonly prescribed medicines in the world.

The Loop-Diuretic-Sensitive Na + -K + -2Cl − Co-Transporter 2 (NKCC2)
NKCC2 is the major salt transport pathway in the apical membrane of the mammalian TAL, a nephron segment where 15–20% of glomerular filtrate is reabsorbed. In addition to its role in salt reabsorption, NKCC2 activity also serves to keep the countercurrent multiplication mechanism by promoting salt concentration in the renal medulla and thus, the renal ability to concentrate urine. Divalent cation (Ca 2+ and Mg 2+ ) and ammonium (NH 4 + ) reabsorption in the TAL also requires the activity of NKCC2 (for reviews see ). As shown in Figure 32.2 , in the case of Ca 2+ and Mg 2+ this is due to the fact that simultaneous operation of NKCC2 with the basolateral chloride channels CLC-KB, the Na +/ K + -ATPase, and the apical inwardly rectifying K + channel known as ROMK, promotes the generation of positive voltage within the TAL lumen, which in turn drives the reabsorption of a second cation via a paracellular pathway, which could be Na + , Ca 2+ or Mg 2+ . Thus, in contrast to what occurs in DCT, in the TAL reducing salt reabsorption is associated with reducing calcium reabsorption. Because of this, loop diuretics are often used in the clinical setting for the management of life-threatening hypercalcemia. In the case of ammonium, this is due to the fact that NH 4 can use potassium transport pathways for its translocation through the plasma membrane. Thus, NKCC2 can also operate as the Na + :NH 4 + :2Cl − co-transporter.
NKCC2 is an important target in cardiovascular and renal pharmacology, because inhibition of this co-transporter is the base of the loop diuretic actions that are the most potent natriuretic agents available for clinical use (furosemide, ethacrinic acid, bumetanide). Loop diuretics decrease the salt reabsorption in TAL, producing significant natriuresis and diuresis. Because loop diuretics reduce interstitial osmolarity of the renal medulla, another mechanism of action is to reduce the concentration capacity of the kidney. Any increase in salt delivery to the macula densa is expected to be compensated by reducing the glomerular filtration rate due to the tubuloglomerular balance. This compensation is absent, however, in the presence of loop diuretics, because the salt-sensing protein in macula densa is also NKCC2. Since the B variant of NKCC2 is the most abundant variant in macula densa cells, and it is not expressed in shark kidney, which also lacks a macula densa, it has been proposed that NKCC2B is the Cl − sensor in TAL cells. It has been difficult to prove this hypothesis because the variant-specific knockout mice lacking NKCC2B developed a compensatory increase in NKCC2A expression in the TAL cells, and only exhibited a very slight shift to the right of the tubuloglomerular feedback curve. Similarly, the isoform-specific null mice for NKCC2A variant also showed slight changes in tubuloglomerular feedback. These observations suggest that both isoforms working together compose the Cl − sensor in the macula densa.
Increasing net NaCl reabsorption in TAL by hormones generating cAMP via Gs-coupled receptors such as vasopressin, glucagon, parathyroid hormone, β-adrenergic, and calcitonin is a fundamental mechanism for regulating salt transport in this nephron segment. Of these hormones, the most important is the antidiuretic hormone vasopressin, which increases NaCl absorption by TAL through a mechanism that appears to involve trafficking of NKCC2 to the apical plasma membrane. Supporting this proposal it has been observed in medullary TAL that most NKCC2 protein is located in intracellular vesicles, and that addition of cAMP increases the expression of NKCC2 in the apical membrane by activating the exocytosis, rather than by inhibiting the endocytosis of NKCC2-containing vesicles. Additionally, long-term increases in vasopressin are associated with increased expression of NKCC2 and maximal urinary concentration ability.
The Loop-Diuretic Sensitive Na + -K + -2Cl − Co-Transporter 1 (NKCC1)
NKCC1 is the Na + -K + -2Cl − co-transporter that is present in secretory epithelium, as well as in many non-epithelial cells. At the cellular physiology level, NKCC1 is very important for cell volume and [Cl − ] i regulation. The diverse phenotypes of NKCC1 knockout mice illustrate the role of this transporter in numerous physiological processes. NKCC1 knockout mice feature deafness due to both disrupted epithelial secretion in the labyrinth and a sensorineural defect, infertility due to a deficiency in spermatocyte production, cecum bleeding and blockade of the colon due to impaired intestinal secretion, salivation impairment, and low blood pressure due to vascular and renal effects. Blood pressure in NKCC1 null mice is decreased due to reduced vascular tone. In this regard, the loop diuretic bumetanide decreased blood pressure in normal mice by inhibiting the activity of NKCC1 in vascular beds, and reduced the vascular smooth muscle cells’ myogenic tone. Both effects are not present in the NKCC1 null mice, strongly suggesting that are the results of inhibiting NKCC1 in blood vessels. Of note, however, one study using telemetry to monitor blood pressure day and night for several days failed to confirm hypotension in the NKCC1 knockout mice, and suggested a salt-sensitive component because a significant increase in blood pressure was produced by a high-salt diet. Finally, NKCC1 is expressed in the basolateral membrane of the macula densa cells, where it has been suggested that it modulates renin secretion.
Molecular Biology of the Sodium-Dependent Chloride Co-Transporters
The Thiazide-Sensitive Na + -Cl − Co-Transporter
Following an expression cloning strategy using the functional expression system of Xenopus laevis oocytes, NCC cDNA was first identified at the molecular level from the winter flounder ( Pseudopleuronectes americanus ) urinary bladder. This clone was named as TSC (for thiazide-sensitive co-transporter), and later changed to NCC. The flounder’s transcript produced a 3.7 kb cDNA clone containing a 3609 bp open reading frame that predicted a 1023 amino acid residues protein with a core molecular mass of 112 kDa. The computer-based analysis hydrophobicity/hydrophylicity suggested the putative basic topology of the Na + -coupled-Cl − co-transporters shown in Figure 32.3a . The short amino terminal domain is followed by a central hydrophobic domain containing what appear to be 12 α-helices compatible with transmembrane-spanning segments. A long carboxyl terminal domain follows this. The amino and carboxyl terminal domains are predicted to be located within the cell. The long loop between transmembrane segments 7 and 8 is glycosylated in NCC and NKCC2, and thus presumably in all members of the SLC12A family. In flounder, a shorter 3.0 kb transcript due to alternative splicing is expressed in several tissues including the brain, eye, heart, intestine, gonads, and skeletal muscle. The functional consequence of this variant remains elusive. After the cloning of NCC from the flounder urinary bladder, cDNAs encoding NCC from a variety of mammalian sources were isolated, including rat ( Rattus norvegicus ), mouse ( Mus musculus ), rabbit ( Oryctolagus cuniculus ), and human ( Homo sapiens ). Additionally, the NCC cDNA sequence has been deposited in gene databases for at least another 10 species, mostly mammalians and one birth. The degree of identity between mammalian NCCs is ~90%, and of any mammalian with flounder is ~60%. Molecular identification of putative NCC sequences in eel suggests the existence of two different NCC genes. NCCα is expressed only in eel kidney, while NCCβ was observed in many tissues, but more abundantly in intestine. The amino acid residues of NCCα and NCCβ are 1027 and 1043, respectively. No functional expression was analyzed, but the extend of identity of NCCα or NCCβ with any NCC, NKCC1 or NKCC2 supports the proposition that these eel sequences probably correspond to NCC, since degree of identity of NCCα or NCCβ with any NCC sequence is higher than with any NKCC1 or NKCC2. In mammals, rabbit and human NCC is longer than other species due to the presence of 17–26 amino acid residues in the carboxyl terminal domain. These extra residues were shown to be encoded in humans by a separate exon (exon 20) which is not present in rodents. It is noteworthy that in humans, there is a putative protein kinase A (PKA) site (RPS) within the extra fragment. No functional significance for this extra exon in humans has been reported, but an extensive proteomic analysis of human urinary exosomes revealed, among may proteins found, the presence of several fragments of NCC, some of which have the serine 811 phosphorylated, that correspond to the putative protein kinase A site RPS contained by the human exon 20. The expression of NCC protein has been confirmed using specific antibodies in intestine, bone cells, and lens. Additionally, in silico analysis of NCC expression revealed that NCC transcripts are abundantly present in sensory ganglia, such as trigeminal and dorsal root ganglion. No report has confirmed the presence of NCC protein in this tissue, and its functional significance is unknown.

The Loop-Diuretic-Sensitive Na + -K + -2Cl − Co-Transporter 2 (NKCC2)
The product of SLC12A1 is the Na + -K + -2Cl − co-transporter known as NKCC2, and it has been located exclusively in the apical membrane of the TAL. Two groups simultaneously identified the cDNA encoding both Na + :K + :2Cl − co-transporter isoforms. Hebert and co-workers cloned the cDNA encoding NKCC2/BSC1 from rat renal outer medulla, and then NKCC1/BSC2 from a mouse inner medullary collecting cells cDNA library, while Forbush and co-workers first identified NKCC1/BSC2 cDNA from a shark rectal gland cDNA library, and later NKCC2/BSC1 from rabbit renal outer medulla. Later, human and mouse NKCC2 sequences were reported. Isolated cDNA clones were about 4.5 kb in size, with an open reading frame of 3285 bp encoding a 1095 residue protein. The predicted NKCC2 topology ( Figure 32.3A ) is highly similar to NCC, featuring the central hydrophobic domain with 12 putative membrane-spanning segments that is flanked by the predominantly hydrophilic amino terminal domain (~165 amino acids) and carboxyl terminal domain (~450 residues). Functional expression analysis in Xenopus laevis oocytes demonstrated that NKCC2 encodes a bumetanide-sensitive Na + -K + -2Cl − co-transporter. It was observed, in a tissue distribution analysis using Northern blot, that NKCC2 transcripts are exclusively present in the renal outer medulla. All other tissues were negative and, until today, no study has confirmed the presence of NKCC2 protein in any other tissue or culture cell. It was proposed that this is due to the presence of a tissue-specific promoter. Interestingly, however, as shown for NCC, in silico distribution analysis suggests the existence of NKCC2 transcripts in the sensory ganglia, such as trigeminal and dorsal root ganglion.
SLC12A1 gives rise to a number of alternative splicing variants. At least six isoforms have been suggested to be present in the mouse kidney. Two independent alternatively splicing mechanisms are responsible ( Figure 32.3a,b ). One splicing event was first observed in rabbit, and later also in mouse, rat, and human kidney. Three isoforms or variants arise from this splicing mechanism that involve the existence of three mutually-exclusive cassette exons nominated as A, B, and F ( Figure 32.3a ). These cassettes are composed of 96 bp encoding a 32 amino acid that is part of the second part of the transmembrane segment 2 and the first part of the intracellularly-located interconnecting segment between TM2 and 3 ( Figure 32.3c ). Thus, three NKCC2 proteins are produced differing only in the 32 amino acid residues, and all three are present in the mammalian NKCC2s known to date. Only variants A and F have been identified from Squalus acanthias (shark) kidney.
Another splicing of SLC12A1 gene that was observed in the mouse kidney is due to the presence of a poly-adenylation site located in the intron between exons 16 and 17, resulting in a shorter C-terminal domain with an extra 55 amino acid residues, not present in the long isoform ( Figure 32.3b ). Thus, two NKCC2 proteins are produced. These are identical from the first residue in the amino terminal domain to residue 74 of the carboxyl terminal domain. After this point, the longer isoform contains 383 residues not present in the shorter isoform, while the shorter contains 55 residues not present in the longer variant ( Figure 32.3c ). The existence of the shorter isoform in mouse kidney was demonstrated by a specific polyclonal antibody directed against the 55 unique piece of the shorter isoform. By means of RT-PCR, it was shown that mouse kidney exhibits transcripts of the three isoforms A, B, and F, combined with both possibilities for the carboxyl terminal domain, suggesting that both splicing mechanisms are independent of each other and thus, that a total of six isoforms are predicted to be produced in mouse kidney: three long NKCC2 isoforms (A, B, and F), and three short NKCC2 isoforms (A, B and F). The possible functional significance of spliced isoforms is discussed below.
The Ubiquitous Bumetanide-Sensitive Na + -K + -2Cl − Co-Transporter (NKCC1)
The SLC12A2 gene encodes the ubiquitously expressed Na + -K + -2Cl − co-transporter. NKCC1 is expressed in the plasma membrane of both epithelial and non-epithelial cells. In the first case, it is limited to the basolateral membrane in which the activity NKCC1 serves to provide the cell with the potassium or chloride ions to be secreted through the apical membrane. The only exception is the choroid plexus, in which both the Na + -K + -ATPase and NKCC1 are expressed in the apical membrane. NKCC1 has been identified at the molecular level in several species, including mouse, rat, human, Bos taurus (bovine), shark, Anguilla anguilla (eel), and Dicentrarchus labrax (sea bass), and even from the plant Arabidopsis thaliana . The existence of one alternatively-spliced isoform of NKCC1 has been suggested. This is a slightly shorter splice variant present in mouse brain total RNA due to the lack of 48 bp corresponding to the entire exon 21. This exon contains a potential PKA phosphorylation site. The existence of a splice transcript was supported by an RNAse protection assay. Distribution analysis within the brain showed that an NKCC1 transcript lacking exon 21 is present in all areas examined except in the choroid plexus, where only the full-length isoform containing exon 21 is expressed. Functional expression in heterologous systems revealed that the shorter variant behaves as a Na + -K + -2Cl − co-transporter. Interestingly, it was recently shown that exon 21 of NKCC1 is implicated in differential sorting in polarized epithelial cells.
Genes, Promoters, and Phylogenetic Analysis
The location of SLC12A1 , the gene encoding NKCC2 in humans is within chromosome 15, in rat within chromosome 3, and in mouse within chromosome 2. SLC12A1 is composed of more than 80 kb and 26 exons have been clearly described to encode for the full length 1095 amino acid residue protein. Mouse is the only species from which the SLC12A1 promoter region has been cloned. The NKCC2 transcript starts with the first exon of 34 bp that is non-coding, followed by a first intron of 1101 bp and a second exon containing the translation start codon. The promoter is composed of 2255 bp. There is a TATA box located at position -29 and consensus recognition sites for several transcription factors, of which the most interesting could be a binding site for HNF-1 at -211 bp. In developing mouse kidney, the expression of HNF-1 precedes the expression of NKCC2. HNF-1 has been implicated in the regulation of tissue-specific expression of genes in liver, pancreas, kidney, and intestine. Deletion of −2255 to −1529 bp of the promoter resulted in a ~three-fold increase in gene transcription rate, suggesting the presence of negative regulatory elements in this segment. No further effect was observed by deleting −1529 to −469 bp, but a significant reduction in gene expression was obtained by elimination from −469 to −190, suggesting that this region contains positive regulatory elements. An HFN-1-binding site is located in this region. A cAMP response element-binding protein is located at nucleotide −1111, and this could be of major importance because it is known that NKCC2 expression is stimulated by vasopressin.
The gene SLC12A2 encodes NKCC1 and is located to chromosome 5q23 in humans, and to chromosome 18 in mouse. The complete gene encompasses a region of 75 kb and is made up of 28 exons. Significant luciferase activity was produced by transfection of mouse IMDC3 cells with a 2063 bp promoter region, together with a luciferase reporter gene. Deletions of >1 kb that reduced the promoter region to 702 or 516 bp resulted in a significant increase in luciferase activity, suggesting the existence of silencer sequences in the deleted bases. Additional deletions resulted in progressive reduction of luciferase activity, suggesting the presence of enhancer elements. Expression of NKCC1 in vascular smooth muscle cells has been suggested to be associated with the development of hypertension in rat models of hypertension. In this regard, a recent report showed that SLC2A2 gene promoter hypomethylation upregulates NKCC1 expression in aorta and heart of spontaneously hypertensive rats.
SLC12A3 , the gene encoding the thiazide sensitive Na + -Cl − co-transporter, is located in humans to chromosome 16q13, in rat to chromosome 19p12-14, and in mouse to chromosome 8. Human SLC12A3 is 55 kb long and contains 26 exons. Transcription initiation is confined to an area from -18 to -6 bp upstream of the translation start codon. The promoter activity observed in the mouse distal convoluted cell line (MDCT), with a construct containing 1019 bp of the 5’ flanking region was reduced only 25% by eliminating the first 885 bp. Sequence analysis of the promoter revealed the presence of a TATA element, two Sp-binding sites, and potential binding sites for NF-1/CTF or NY-I/CP-I. Interestingly, the promoter activity of the rat NCC gene is inhibited by acidosis. This is consistent with a marked fall in renal cortical abundance of NCC protein assessed by Western blot, and by [ 3 H]Metolazone-binding to plasma membranes from the renal cortex of rats exposed to chronic NH 4 Cl-loading.
Functional Properties
The member of the family that has been more extensively characterized is NKCC1. This is because it is expressed in many different cell types that, on one hand, made it accessible for functional characterization, even before the cDNA was identified, and on the other hand, it attracted the interest of many groups of researchers in a variety of fields such as neuroscience, cell volume regulation, red blood cell, and epithelial transport mechanism (for an in-depth review see ). In contrast, before identification of the corresponding genes, functional characterization of NKCC2 and NCC was relatively scarce, and most of the knowledge came from analysis of renal outer medulla or cortical plasma membrane-binding of tracer [ 3 H]Bumetanide or [ 3 H]Metolazone, respectively. With the cloning of cDNAs encoding the SLC12A co-transporter proteins and several variants, in-depth characterization of their major functional, pharmacologic, and some regulatory properties has been made possible.
The Thiazide-Sensitive-Na + -Cl − Co-Transporter
Heterologous expression of teleost, rat, mouse, and human NCC has been achieved in Xenopus laevis oocytes. Oocytes are not epithelial cells; however, this expression system is a useful tool for robust and reproducible NCC expression. Unfortunately, expression of NCC in mammalian cells transfected with NCC cDNAs has been almost impossible to achieve, and so far has been reported only by two independent groups in MDCK and HEK-293. However, in both cases, the level of NCC expression was low, and not sufficient for that required to define the functional properties of the co-transporter. Thus, Xenopus laevis continued to be the best cells to assess NCC activity, and basically all of what is known today regarding the functional properties and regulation of NCC activity comes from studies performed in oocytes. Recent reports from Hoover and co-workers showed that a subcloned version of the immortal mouse DCT cell line originally produced by Gesek, Friedman and co-workers exhibit what appears to be a robust expression of endogenous NCC, promising an excellent tool for NCC functional analysis in a mammalian cell system.
As shown in Table 32.1 , a number of interesting differences have been observed between fish (flounder) and mammalian (rat and mouse) NCC. The affinity for Na + and Cl − in rat or mouse NCC proteins is significantly higher than the affinity observed in the flounder NCC. In addition, the Km values for Na + and Cl − in mammalian NCCs are similar, whereas in flounder the Km value for extracellular Cl − is lower than the value for Na + . NCC activity is inhibited by thiazide-type diuretics with the following profile: polythiazide > metolazone > bendroflumethiazide > trichloromethiazide > chlorthalidone. However, for all thiazides, flounder’s NCC exhibited lower affinity. At a concentration of 100 μM, the thiazides with lower potency, trichloromethiazide and chlorthalidone, reduced flounder NCC activity by only 68 and 46%, respectively, whereas the same concentration of all thiazides inhibited rat NCC by >95% .
Rat NCC | Mouse NCC | Flounder NCC | |
---|---|---|---|
Na + Km (mM) | 5.5±1.0 | 7.2±0.4 | 30.0±6.0 |
Cl − Km (mM) | 2.6±0.6 | 5.6±0.6 | 15.2±2.0 |
Metolazone IC 50 μM | 0.3±0.001 | 0.4±0.001 | 4.0±0.08 |
Two proposals for the order of ion-binding to NCC have been suggested. By assessing the [ 3 H]metolazone-binding to membranes extracted from rat renal cortex, Tran and collaborators observed that Na + increased tracer-binding to the putative thiazide-sensitive transport protein. In contrast, Cl − decreased the affinity for metolazone. Thus, the model that was proposed included two binding sites within the thiazide receptor: one selective for Na + ; and the other that recognizes either Cl − or metolazone in a competitive fashion. In this model, occupation of the Na + site increases the affinity of the second site for either Cl − and/or metolazone.
The second model was based on observations of functional properties of NCC as expressed in Xenopus laevis oocytes. Monroy et al. observed that affinity for Na + or Cl − changed as a function of counterion concentration. The lower the extracellular Na + concentration, the lower the Cl − affinity, a relationship that supports the initial conclusions of Tran et al. However, it was also observed that lower extracellular Cl − concentrations coincided with lower Na + affinity. In addition, it was observed that the IC 50 for metolazone inhibition increased when the thiazide dose–response curves were measured in lower Na + or Cl − conditions, suggesting that both ions compete with metolazone for binding to the co-transporter. Thus, the proposed model included a random order of binding, with both ions affecting affinity for the counterion and competing with thiazide diuretics. Supporting this model, data produced later that is discussed below suggest that affinity-defining domains or residues for Cl − – and thiazide-binding are located in different parts of the protein.
The Loop-Diuretic-Sensitive Na + -K + -2Cl − Co-Transporter 2 (NKCC2)
Several NKCC2 splice variants in different species have been analyzed at tissue-specific expression and functional level, including shark, rat, mouse, rabbit, and human. As previously discussed, there are three variants of NKCC2 named A, B, and F ( Figure 32.3c ). The three variants from mouse perform as Na + -K + -2Cl − , suggesting that the difference between variants could be in the kinetic properties for ion transport and/or bumetanide affinity. This hypothesis was supported by intrarenal localization studies that demonstrated axial distribution of these variants along TAL. As shown in Figure 32.3d , the A isoform is present in both cortical and medullary TAL. In contrast, the B isoform is present only in cortical TAL, and the F variant is expressed only in the inner stripe of the outer medulla. Early studies demonstrated that the NaCl transport rate in mTAL is significantly more rapid than in the cTAL, but with greater diluting power in the later segment. Thus, the possibility for heterogeneity of the transport process was suggested. Evidence supporting this hypothesis was obtained simultaneously by Plata et al. and Gimenez et al. using mouse and rabbit NKCC2 variants, respectively ( Figure 32.3e ). NKCC2F exhibits the lowest affinity for co-transported ions, and is more sensitive to changes in extracellular osmolarity. As shown in Figure 32.3d , NKCC2F is predominantly expressed in the inner stripe of the outer medulla, where the salt concentration is very high, and where greater changes in extracellular osmolarity occur. NKCC2A exhibits the highest transport capacity and is expressed along all TAL. Finally, NKCC2B, the variant that is expressed only the cTAL where ion concentration of the tubular fluid has decreased to values even below those in plasma, is the variant with the highest affinity for the co-transported ions. Thus, the dilution power along TAL is explained by the presence of three alternatively-spliced variants of NKCC2 with distinct functional characteristics. The NKCC2 influx data from Plata et al. were later used to construct a mathematical model for the NKCC2 co-transporter isoforms.
As discussed above, a shorter variant containing 55 amino acid residues at the end that are not present in the longer NKCC2 isoform has been described ( Figure 32.3b,c ). This variant is only present in mTAL, not in cTAL and performs as a K + -independent, but nevertheless, loop diuretic-sensitive Na + -Cl − co-transporter that is activated by hypotonicity and inhibited by cAMP. Therefore, the shorter NKCC2 variant may provide a molecular explanation for previous physiological studies that suggested a switch from Na + -Cl − to Na + -K + -Cl − co-transporter mode in TAL by extracellular osmolarity or by the presence of vasopressin. Eveloff and co-workers observed, in rabbit TAL cells, the existence of a K + -independent, furosemide-sensitive Na + -Cl − co-transporter in hypotonic conditions that became K + -dependent, constituting the Na + -K + -2Cl − co-transporter, when cells were changed to isotonic medium. In addition, Sun et al. found that in mouse TAL cells, vasopressin (i.e., cAMP) shifts the mode of apical co-transport from Na + -Cl − (in its absence) to Na + -K + -2Cl − (in its presence). Consistent with these studies, the large NKCC2 is a Na + -K + -2Cl − co-transporter that is activated by increased tonicity or the presence of vasopressin, while the shorter NKCC2 is a Na + -Cl − co-transporter that is activated in hypotonicity or by inhibition of protein kinase A activity.
The shorter variant also exerts a dominant-negative effect upon the activity of the longer NKCC2 that can be abrogated by cAMP. Using confocal microcopy, in oocytes injected with both the long (NKCC2F) and the short variant of mouse NKCC2, Meade et al. observed that the short NKCC2 reduced the activity and surface expression of the long NKCC2. This effect could be prevented by cAMP, and correlated with the observation that the short NKCC2 variant prevented co-transporter trafficking and surface membrane expression of the long NKCC2. These studies thus suggested that in mouse medullary TAL, activation of Na + -K + -2Cl − co-transporter by hormones that increase intracellular cAMP (e.g., vasopressin, PTH) requires the presence of the short NKCC2 protein. The absence of cAMP allows the short form of NKCC2 to reduce co-transporter activity, whereas in the presence of cAMP, the negative effect of the short isoform on NKCC2 is inhibited. In this regard, Mount and collaborators observed that expression of the short NKCC2 is axially distributed along TAL, as cortical TAL appears to lack this isoform. This heterogeneity may explain the observation that in mouse the vasopressin effect is present only in the medullary TAL. Interestingly, the short isoform is also expressed in the thin ascending limb ( Figure 32.3d ), but its significance in this region is not known.
Structure–Function Relationships
The proposed topology for the Na-coupled chloride co-transporters NKCC1, NKCC2, and NCC is shown in Figure 32.3 . There is only one study that has addressed the topology at the biochemical level. Gerelsaikhan and Turner used an in vitro translation experiment with a variety of human NKCC1 constructs with the carboxyl-terminal reporter sequence containing multiple N-linked glycosylation sites located after each of the putative transmembrane domain. The authors concluded that the amino- and carboxyl-terminal domains are located intracellulary and flank the central hydrophobic domain in which the first eight TM segments are easily identifiable, exhibiting the classical ~20 residue α-helices. It was impossible to differentiate between TMs 9 and 10, and between TMs 11 and 12, and thus it was proposed that probably there are only two large TMs of ~36 residues in length that form tight hairpin-like structures in the membrane or take up either a non-helical or a partial-helical structure.
Ion or Diuretic Affinity Modifier Domains or Residues
Several attempts to begin to understand structure–function relationship issues in the Na + -coupled Cl − -co-transporters have been made on NKCC1 (for review see ), NKCC2, and NCC. In most cases, functional differences in NKCC1 or NCC between fish and mammalian orthologs have been exploited to design chimeric proteins to begin to define domains, regions or individual residues that are defining the ion transport or diuretic-binding affinities and/or specificity. In the case of NKCC2, most of the structure–function information available comes from the functional analysis of alternatively spliced variants.
The identity degree between shark and human NKCC1 is 74%, and interesting functional differences occurs between these orthologs ( Table 32.2 ). Several chimeric constructs were studied by interchanging fragments of NKCC1 between human and shark cDNAs. First, the amino- and carboxyl-terminal domains between both species were swapped, providing evidence that residues located within the central hydrophobic core define ion affinity. Then, a variety of chimeras were constructed and analyzed by in-depth kinetic analysis. The chimeric design took into account the transmembrane segments that are identical in NKCC1 of both species. According to the results, the three TM segments playing an important role in ion transport kinetics in NKCC1 are TM2, TM4, and TM7. TM2 is involved in Na + and Rb + kinetics, TM 4 in Rb + and Cl − kinetics, and TM7 in Na + , Rb + , and Cl − kinetics. Interestingly, the behavior of several chimeric proteins with respect to bumetanide inhibition was completely different from that observed in ion transport kinetics. In the case of bumetanide, data suggested that TM segments 2–6 and 10–12 play a role in defining affinity for loop diuretics. Thus, results from studies using shark and human NKCC1 chimeric co-transporters argued against a previous hypothesis that loop diuretics and Cl − compete for the same binding site.
Sodium Km (mM) | Rubidium Km (mM) | Chloride Km (mM) | Bumetanide Ki (μM) | Reference | |
---|---|---|---|---|---|
hNKCC1 | 19.6±4.9 | 2.68±0.72 | 26.5±1.3 | 0.16 | |
hNKCC1 | 15.2±1.5 | 1.6–2.5 | 31±1.0 | 0.044–0.079 | |
HEK-293 | 22 | 12 | 110 | 0.054 | |
sNKCC1 | 42 | 12 | 110 | 0.54 | |
sNKCC1 | 165±34 | 14±8.0 | 101±24 | 0.57 | |
sNKCC1 | 113±11 | 9.6–11.6 | 102±7 | 0.22–0.30 |
Analysis of the spliced variants A, B, and F of NKCC2 revealed a clear role of the segments encoded by these exons (TM2) in transport kinetics properties, not only for Na + and Rb + , but also for Cl − and bumetanide ( Figure 32.3 ). Further studies showed that NKCC2 variants A and F, but not B, are present in the shark kidney, and that also in shark, the affinity for transported ions of the NKCC2A variant is higher than the NKCC2F variant. Additional chimeric proteins were constructed: chimera A/F contained the TM2 sequence of variant A followed by an interconnecting sequence of variant F, and vice versa . The results suggested that Cl − transport affinity-defining domains between isoforms A and F were located within the predicted interconnecting segment between TM2 and TM3, rather than within the TM2 segment. The chimeras’ behavior with respect to bumetanide affinity was opposite to that of Cl − affinity, suggesting that residues located within TM2, and not within the TM2–TM3 interconnecting segment, play a role in defining bumetanide affinity. The most recent study regarding structure–function relationship on the NKCC2 variants included a series of single or multiple point mutations of rabbit NKCC2 variants B and F or A and B, in order to switch one or few residues between variants. The results demonstrated that replacement of six residues in NKCC2B resulted in a variant with Na + and Cl − affinities identical to those of NKCC2F. Interestingly, three of these residues are located within the TM2 segment (ATG in B isoform were switched to TAY), and the other three within the interconnecting segment between TM2 and TM3 (SVT in B isoform were changed to MCV). The six mutations (ATG-TAY/SVT-MCV) turned NKCC2B into the F variant ( Figure 32.3c ), suggesting that the interconnecting loop between TM2 and TM3 might actually be part of a membrane-embedded domain.
The domains or residues defining the affinity for co-transporter ions or inhibitors may not be the same as the residues critical for the specificity for certain ions or inhibitors. All studies discussed above were performed in native, chimeric, and/or mutant NKCC1 or NKCC2 that were expected to behave as Na + -K + -2Cl − co-transporters. Thus, no information was obtained concerning structural requirements to define specificity for ions or diuretics. In this regard, the short isoform of NKCC2 in mouse perform as a K + -independent, but loop diuretic-sensitive, Na + -Cl − co-transporter, suggesting that sequences within the carboxyl-terminal domain could be critical to endow NKCC2 with K + transport ability. However, a study in which chimeras were constructed by swapping the amino- and carboxyl-terminal domains between NCC and NKCC2F showed that chimera with the central hydrophobic domain of NKCC2, flanked by amino- and carboxyl-terminal domains of rat NCC, exhibit NKCC2 behavior. That is, as a bumetanide-sensitive Na + -K + -2Cl − co-transporter, indicating that the residues that endow rat NKCC2F with its functional properties should be located within the central hydrophobic domain. Thus, the explanation on how the short NKCC2 variant performs as a bumetanide-sensitive Na + -Cl − co-transporter remains elusive. The unique 55 amino acid segment at the end of this isoform contains several negatively-charged residues, suggesting that this fragment could interact with the co-transporter core to prevent K + translocation, like the ball and chain mechanism proposed for some membrane channels.
Similar chimera construction and directed point mutation approaches have been used to gain insight into the structure–function relationships of NCC. It was first observed that rat and mouse NCCs exhibit similar functional properties that are, however, significantly different from those shown for flounder NCC. Differences in functional properties and primary sequences between rat and flounder NCCs were exploited to define affinity-modifying domains and residues. Figure 32.4 depicts what is known regarding the structure–function relationship of NCC. By means of silent restriction sites, NCC cDNAs from rat and flounder were divided into five fragments: amino terminal domain; TM segments 1–7; extracellular glycosylated loop; TM segments 8–12; and the entire carboxyl-terminal domain, and then fragments were swapped between them to obtain a variety of combinations. The results first indicated that the central hydrophobic domains define functional characteristics of NCC. Chimeras in which the TM segments 1–7 were interchanged between flounder and rat NCCs demonstrated that affinity-defining residues for Cl − are located within these segments. Similarly, affinity-defining residues for thiazide inhibition are located within TM segments 8–12. The observation that Cl − affinity is defined by residues located within TM segments 1–7 is supported by another study, in which functional consequences of single nucleotide polymorphisms (SNP) located within the exons of the human SLC12A3 gene changed the primary structure of NCC. The results revealed that a highly-conserved glycine within the fourth transmembrane domain plays a critical role in defining the level of co-transporter activity and the affinity for Cl − . Accordingly, it was observed that a glycine-to-alanine SNP at position 264 resulted in decreased activity of the co-transporter by 50%, with increased affinity for Cl − by one order of magnitude. Based on these observations, it was later observed that this SNP on NCC increases the response of humans to loop diuretics, probably because of the reduced capacity of DCT to compensate for the effect of inhibiting salt reabsorption in TAL. The information that thiazide affinity-defining residues were located within TM8 to TM12 was further explored by site directed mutagenesis, revealing that a single residue in rNCC and flNCC is responsible for the differences in affinity for thiazides between mammalian and fish co-transporters. The serine 575 in rat NCC corresponds to the cysteine 576 in flounder NCC. By changing this residue, the affinity for thiazides can be transformed from mammalian to fish affinity, and vice versa . It is worth noticing that the NCC mutants that changed the affinity for thiazides had no effect upon the co-transporter affinity for Cl − , arguing against the proposal that thiazide diuretics and Cl − compete for the same binding site.

Analysis of the putative glycosylation sites in rat NCC by means of mutagenesis revealed that glycosylation itself is another component of the co-transporter that affects thiazide affinity. In one study, eliminating the glycosylation sites located within the extracellular loop between TM segments 7 and 8 has remarkable consequences in NCC transport activity and properties. Elimination of one site (either N204 or N242) resulted in a 50% reduction of NCC activity, and elimination of both sites was associated with a 95% reduction. Interestingly, prevention of glycosylation was associated with increased affinity for extracellular Cl − and metolazone. This effect of glycosylation on thiazide affinity seems to be unique to rat NCC, since it was not observed after preventing glycosylation of flounder NCC or rat NKCC2F. In this last co-transporter, elimination of glycosylation by means of site directed mutagenesis was associated with increased affinity for extracellular chloride, but no effect was observed on the affinity for the loop diuretic bumetanide.
The carboxyl terminal domain appears to have an important role in maturation and trafficking of the co-transporters. A region of 77 amino acid residues of NKCC2 (amino acid 708–884), that is not present in NKCC1, seems to be required for driving NKCC2 to the apical membrane in polarized cells, while there is a dileucine motif NKCC1, not present in NKCC2, that directs this co-transporter to the basolateral membrane. A LLV motif in NKCC2 was identified as an ER retaining signal. A protein known as SCMP2 influences NKCC2 expression through recycling endosomes and interfering with its exocytotic trafficking.
The Na-Coupled Chloride Co-Transporters Form Homodimers
Moore-Hoon and Turner used rat parotid plasma membrane to analyze NKCC1 using the reversible chemical cross-linker DTSSP (3,3’-dithiobis-[sulfosuccinimidyl propionate]). They observed that NKCC1 migrates at ~335 kDa. After protein denaturation, single monomers of approximately ~170 kDa were obtained, in which the investigators were unable to detect the presence of any additional protein. Similar results were later observed on human NKCC2 by Starremans et al., and on NCC by De Jong et al. These studies were performed in Xenopus laevis oocytes injected with in vitro -transcribed cRNA from FLAG- and/or HA-tagged wild-type co-transporters and concatamer constructions. These experiments revealed that FLAG-NKCC2 and HA-NKCC2 are physically linked. Similar observations were obtained for FLAG-NCC and HA-NCC constructs. Thus, NKCC1, NKCC2, and NCC are able to form homodimers, and might be functional in this conformation. Because dimers are sensitive to reducing agents such as β-metcaptoethanol, it is likely that disulfide bonds between cysteines are involved. Additionally, some data suggest that members of the SLC12A family can build heterocomplexes comprised of different members of the family. For example, an orphan gene of the family known as CIP appears to inhibit transport activity of NKCC1 or activate KCC2. However, it does not affect the activity of other family members, raising the possibility that CIP may form an activating heterocomplex specifically with NKCC1. A recent study has shown, by means of yeast two-hybrid and pull down assays, that K + -Cl − co-transporter isoforms can interact among themselves, and even with NKCC1.
Regulation of Sodium Cation-Coupled Co-Transporters
The activity of the sodium–cation coupled chloride co-transporters, as occurs with many other ion transport proteins, can be modulated at multiple levels. One is the level of expression of the protein, which in turn can be due to increase in the rate of gene transcription, in the stability of the messenger RNA or post-translationally by affecting the turnover rate of the protein. This level of regulation requires activation/deactivation of synthesis/degradation mechanisms that usually take time to install, and thus are preferred with increased or decreased activity of the co-transporters required for longer periods of time. Another level of regulation is the balance between endocytosis and exocytosis of the co-transporter containing vesicles into the plasma membrane, as only the fraction of co-transporters inserted in the membrane are able to translocate ions. Finally, a co-transporter that is already in the membrane can be regulated to increase or decrease its turnover rate due to conformational changes that affect their activity. The presence of a co-transporter in the cell surface and its intrinsic level of activity are often modulated by phosphorylation/dephosphorylation processes and thus, are subjected to a rapid regulation through cascades of second messengers induced by a variety of hormonal signals.
With the availability of cDNA probes, primers, and high-quality polyclonal antibodies against NCC or NKCC2, several strategies have been implemented to study patterns of these co-transporters abundance, and changes under several physiological and pathophysiological circumstances. Discussion of each model is beyond the scope of this chapter, but Tables 32.3 and 32.4 summarize the several experimental, physiological or pathophysiological conditions in which the level of NCC or NKCC2 protein and/or mRNA expression has been analyzed. In general, increased expression of either NCC or NKCC2 is observed in models in which salt retention is induced, while decreased expression is observed by salt-wasting stimuli.
mRNA | Protein | References | |
---|---|---|---|
Low Na + diet | ↔ **** ↑ ** | ↑ | |
Loop diuretics | ↔ * ↑ **** | ↑ | |
Spironolactone & | ↔ * | ↑ | |
Thiazide diuretics | – | ↑ | |
Low-protein diet | – | ↑ + | |
L-NAME therapy | – | ↑ | |
Aldosterone | – | ↑ | |
Aldosterone + high-salt diet (4%) | ↑ | ↑ | |
Fludrocortisone | ↑ | ||
dDAVP | – | ↑ b ↔ c | b: ; c: |
Vasopressin escape | – | ↑ # | |
dDAVP + candesartan | – | ↑ d ↔ c | d: ; c: |
Obesity | – | ↑ | |
Angiotensin II | – | ↑ | |
Metabolic acidosis | – | ↑ | |
Estradiol | – | ↑ | |
Prenatally programmed hypertension a | ↑ ** | ↑ | |
Chronic renal failure + dDAVP (surgical reduction of renal mass) | – | ↑ | |
KS-WNK1 KO model | – | ↑ | |
Dexamethasone | – | ↑ | |
Tacroliums | – | ↑ | |
Isoproterenol | – | ↑ | |
WNK4 BAC transgenic mice. Two wild-type and two PHAII WNK4 alleles | – | ↑ | |
PHAII WNK4 knockin mice (WNK4 +/D561A ) | – | ↑ | |
Conditional kidney-specific Nedd4-2 knockout mice | – | ↑ | |
WNK1 knockout | – | ↑ | |
Kidney specific OSR1 knockout | – | ↑ | |
Gentamicin | – | ↔ | |
Water restriction | – | ↔ | |
Insulin | – | ↔ | |
Chronic noradrenaline infusion | – | ↔ | |
Lithium (low dose or high dose) 40 versus 60 mmol lithium/kg dry food | – | ↔ | |
Chronic renal failure (surgical reduction of renal mass) | – | ↔ | |
High-K + diet | – | ↓ | |
High-K + diet and HS diet after unilateral nephrectomy | – | ↓ | |
Low-K + diet | ↓ * | ↓ | , |
DOCA | – | ↓ | |
Candesartan | – | ↓ e ↔ c | e: ; c: |
Chronic renal failure + candesartan | – | ↓ | |
Aldosterone escape | ↔ **** | ↓ | |
L-NAME + aldosterone escape | – | ↓ | |
Bilateral ureteral obstruction | – | ↓ | |
Bilateral ureteral obstruction and release of obstruction (Day 14) | – | ↓ | |
Puromycin-induced nephrotic syndrome | – | ↓ | |
Spironolactone infusion in low-salt (0.7%) diet | ↓ | ↓ | |
SGK1 inducible knockout mouse model | ↔ | ↓ | |
Cyclosporine | – | ↓ | |
WNK4 BAC transgenic mice. Four wild-type WNK4 alleles | ↓ | ↓ | |
SPAK knockin mice (SPAK T243A/T243A ) | ↓ | ↓ | |
SPAK knockout mice | – | ↓ | |
Overexpression of WNK1 | – | ↓ | |
Dehydration | ↑ **** | – | |
High-Na + diet | ↔ *** ↓ ** | ↓ | |
Saline-loading | ↔ *** | – | |
Water-loading | ↔ *** | – |
& in loop- diuretic treated animals.
+ Associated with high plasma aldosterone levels.
# Increased NCC protein during days 1 and 2, no difference in days 3 or 7.
a Prenatal hypertension induced by low-protein diets in pregnant rats.
mRNA | Protein | References | |
---|---|---|---|
Low-Na + diet | ↔ * | ↔,↑ | |
Loop diuretics | ↔ * | ↑ | |
Low-protein diet | – | ↑ + | |
Dexamethasone | ↑ **** | ↑ | |
High-fat diet | – | ↑ | |
Lipopolysacharide injections | – | ↑ ++ | |
Candesartan | – | ↑ | |
Chronic renal failure + candesartan | – | ↑ | |
L-NAME therapy | – | ↑ | |
Diclofenac/indomethacin | – | ↑ | |
Growth hormone | – | ↑ $ | |
Obesity | – | ↓↑ | |
Rosiglitazone | – | ↑ | |
Hyperosmolarity | – | ↑ | |
Bilateral ureteral obstruction and release of obstruction (Day 14) | – | ↑ | |
Angiotensin II | – | ↑ | |
dDAVP | – | ↑ | |
Water restriction | – | ↑ | |
Lithium | – | ↑ | |
Chronic noradrenaline infusion | – | ↑ | |
Prenatally programmed hypertension a | ↑ ** | ↑ | |
Lithium (high dose) 60 mmol lithium/kg dry food | – | ↑ | |
Sildenafil in the presence of lithium-induced DI | – | ↑ | |
Vasopressin escape | – | ↑↓ # | |
Chronic renal failure + dDAVP | – | ↑ | |
(Surgical reduction of renal mass.) | |||
Hypothyroid rat model | – | ↑ | |
Dahl salt-sensitive rats | – | ↑ ## | |
SPAK knockout mice | – | ↑ | |
WNK1 knockout | – | ↑ | |
P2Y2 receptor KO mice with high-salt diet | – | ↑ | |
Aldosterone | – | ↔ | |
Candesartan | – | ↔ | |
Aldosterone escape | – | ↔ | |
dDAVP + candesartan | – | ↔ | |
Chronic metabolic acidosis | ↑ **** | ↔,↑ | |
Lithium (low dose) 40 mmol lithium/kg dry food | – | ↔ | |
Chronic renal failure (surgical reduction of renal mass) | – | ↔ | |
Low-K + diet | ↓ * | ↓ | |
DOCA | – | ↓ | |
Cyclosporine | – | ↓ | |
Parecoxib | ↓ *** | ↓ | |
Gentamicin | – | ↓ | |
Bilateral ureteral obstruction | – | ↓ | |
17-Beta-estradiol | – | ↓ | |
L-NAME + aldosterone escape | ↔ ** | ↓ | |
Puromycin-induced nephrotic syndrome | – | ↓ | |
SGK1 inducible knockout mouse model | – | ↓ | |
High-K + diet and high-salt diet after unilateral nephrectomy | – | ↓ #$ | |
Dahl salt-resistant rats | – | ↓ ### | |
P2Y2 receptor knockout mice with high-salt diet | – | ↓ | |
SPAK knockin mice (SPAK T243A/T243A ) | – | ↓ | |
Overexpression WNK1 | – | ↓ | |
Kidney-specific OSR1 knockout | – | ↓ | |
High-Na + diet | ↔ * | – | |
Saline-loading | ↔ * | – | |
Dehydration | ↔ * | – | |
Water-loading | ↔ * | – |
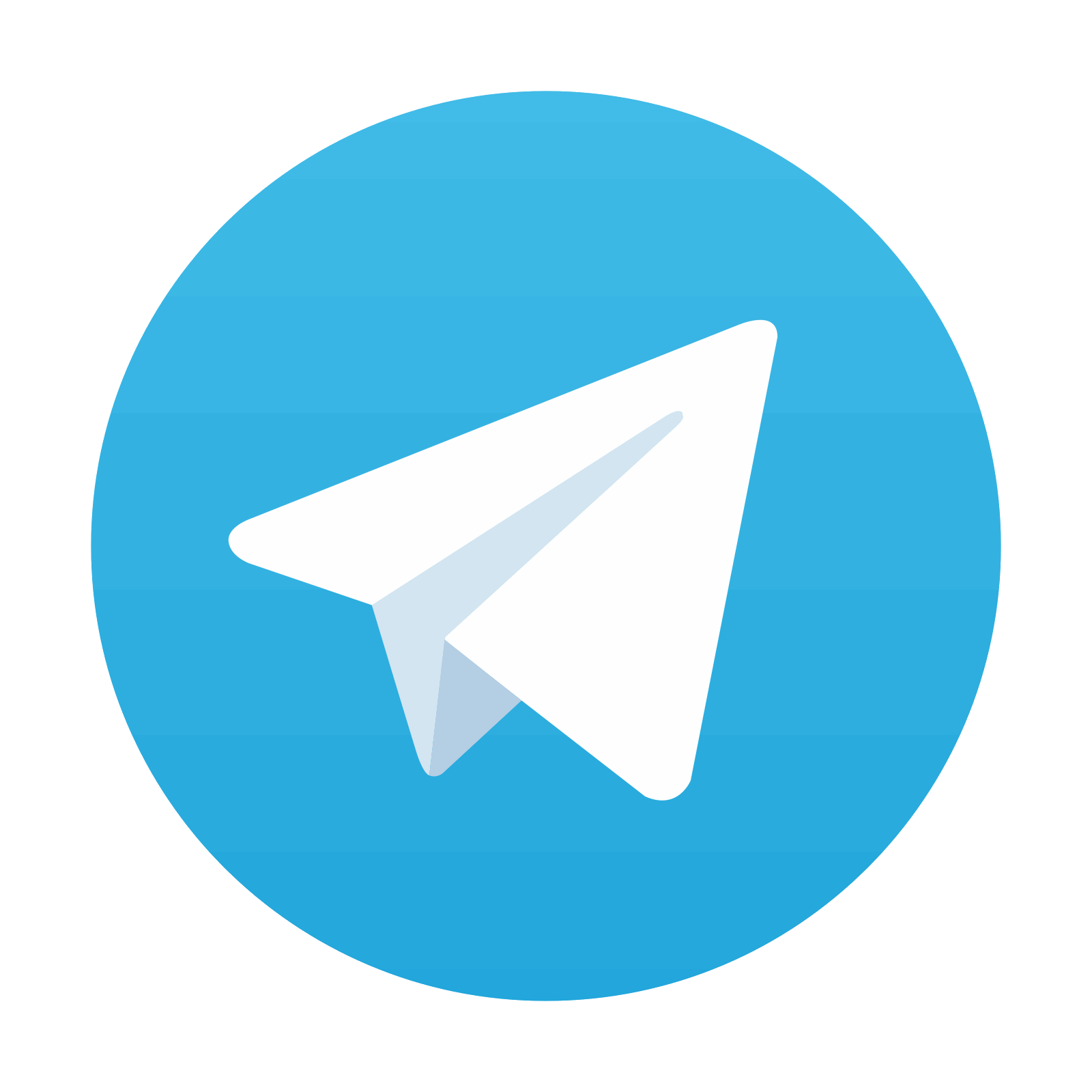
Stay updated, free articles. Join our Telegram channel

Full access? Get Clinical Tree
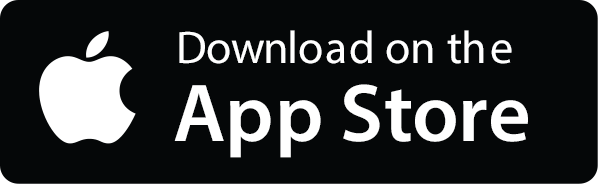
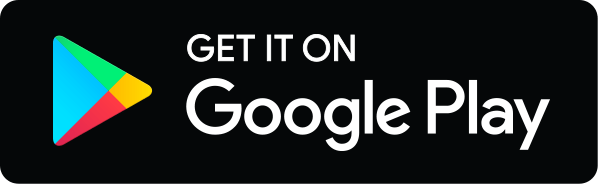
