Classical transmitter ligands evolved about 1000 million years ago. 1 The role of dopamine as a neurotransmitter has evolved with time. In primordial and plant cells, dopamine is present, even though catecholamine signaling is not used. In invertebrate neural systems, dopamine is the pre-eminent catecholamine. In vertebrates, the catecholamine pathway terminates in norepinephrine and epinephrine. 2 Endogenous dopamine was mainly recognized as a precursor to norepinephrine and epinephrine 3 until the late 1950s, when Carlsson demonstrated that dopamine itself had a transmitter role. 4 This finding, for which he was awarded the Nobel Prize in Physiology or Medicine 2000, was revolutionary for the understanding of various central functions of the brain, including memory, learning, drug abuse, cognition, and attention; it has also enlightened us regarding the pathogenesis and treatment of various psychiatric and neurological disorders.
Historical Perspective
Classical transmitter ligands evolved about 1000 million years ago. The role of dopamine as a neurotransmitter has evolved with time. In primordial and plant cells, dopamine is present, even though catecholamine signaling is not used. In invertebrate neural systems, dopamine is the pre-eminent catecholamine. In vertebrates, the catecholamine pathway terminates in norepinephrine and epinephrine. Endogenous dopamine was mainly recognized as a precursor to norepinephrine and epinephrine until the late 1950s, when Carlsson demonstrated that dopamine itself had a transmitter role. This finding, for which he was awarded the Nobel Prize in Physiology or Medicine 2000, was revolutionary for the understanding of various central functions of the brain, including memory, learning, drug abuse, cognition, and attention; it has also enlightened us regarding the pathogenesis and treatment of various psychiatric and neurological disorders.
Since 1910, dopamine has been known to be a vasoconstrictor, but less potent than norepinephrine and epinephrine. However, in 1942 Holtz et al. reported that dopamine, via its oxidized form, decreases blood pressure in the guinea pig and rabbit. In 1958, Hornykiewicz reported that the vasodepressor effect of dopamine is due to dopamine per se . Goldberg et al. confirmed the depressor effect of low doses of dopamine in 1959, and demonstrated a direct negative effect of dopamine on vascular resistance in 1962. The same group reported that dopamine increased renal blood flow, glomerular filtration rate, and sodium excretion in dogs and humans. In 1977, Lokhandwala and Buckley subsequently reported that presynaptic dopamine receptors may be responsible for the dopaminergic inhibition of renal neurogenic vasoconstriction. In 1981, Morgunov and Baines demonstrated a positive relationship between renal sodium and dopamine excretion that was independent of renal nerves, indicating that the kidney can synthesize dopamine. Dopamine had been shown to increase cAMP production in the canine renal artery in 1973, and in renal particulate preparations containing tubules, glomeruli, and blood vessels in 1977. In 1980 Nakajima and Kuruma reported that renal particulate preparations had two binding sites to a radio-labeled dopamine receptor antagonist, indicating two types of dopamine receptors. In 1984, Felder et al. reported that renal tubules and glomeruli express D 1 -like and D 2 -like receptors, respectively. That dopamine can directly inhibit sodium transport in the renal proximal tubule was demonstrated by Bello-Reuss et al. in 1982 ; Aperia et al. in 1987 showed that this occurs, in part, by inhibition of Na + ,K + -ATPase activity. Subsequently, renal endogenous dopamine was shown to be important in the regulation of renal sodium excretion in normotensive rats, but not in spontaneously hypertensive rats (SHRs). In mice, deletion of any of the dopamine receptor gene subtypes produces hypertension, the mechanism of which is specific to the particular dopamine receptor subtype deleted.
Overview
Pharmacological doses of dopamine, such as those administered intravenously to increase blood pressure, achieve concentrations that stimulate other G-protein-coupled receptors (GPCRs) (e.g., α- and β-adrenergic receptors) in addition to dopamine receptors. In this dose range, the vasoconstrictor action of α-adrenergic receptors completely overcomes any vasodilatory effect of dopamine. Normal circulating concentrations of dopamine (picomolar range) are not sufficiently high to activate vascular dopamine receptors, but high nanomolar to low micromolar concentrations can be attained in dopamine-producing tissues. The Ki of dopamine for its receptors may be as low as 5 nM and as high as 2.5 μM.
Dopamine is important in the regulation of water and electrolyte balance, and blood pressure, partly by regulation of the secretion/release of hormones and humoral agents that affect water and electrolyte balance. Dopamine also modulates water and electrolyte intake via the “appetite” centers in the brain, and regulates ion and water transport in the kidney and gastrointestinal tract. Physiological concentrations of locally produced dopamine, acting in an autocrine or paracrine manner, inhibit ion transporter/channel/pump activity directly by actions on enzyme or channel kinetics, and indirectly by regulating their protein expression and cellular distribution. The physiological effects of dopamine occur by occupation of its specific receptors, as well as via synergistic interaction with natriuretic agents/receptors such as atrial natriuretic peptide (ANP)/ANPA, eicosanoids, endothelin/ETBR, nitric oxide, prolactin, urodilatin, angiotensin III/AT 2 R, and negative interaction with anti-natriuretic agents/receptors such as insulin, renin, angiotensin II/AT 1 R, and aldosterone. In general, under normal conditions and especially when extracellular fluid volume is moderately expanded, dopamine impedes ion and water transport and facilitates their excretion. During normal or moderately increased NaCl intake, inhibition of D 1 -like receptors decreases sodium chloride excretion by about 60%. Dopamine, however, is not important in natriuresis with marked volume expansion with isotonic saline or even moderate volume expansion with albumin or hypotonic saline. With volume deficit renal dopamine production decreases ; this may allow sodium retaining mechanisms to work more effectively. The decrease in renal dopamine production during volume depletion has been related to a decrease in angiotensin II-mediated renal tubular uptake of dopamine, and an increase in renal MAO activity and dopamine turnover, without altering COMT activity. 52, Indeed, during volume deficit, exogenous dopamine may actually decrease sodium excretion, apparently caused by an increase in sodium reabsorption in the distal nephron.
Renal Dopamine Production
Plasma dopamine is freely filtered through the glomerulus, but the concentration of free dopamine in the plasma is normally too low (below 1 nmol/L) to account for any significant contribution to urinary dopamine, which is in the high nanomolar to low micromolar range. Renal deconjugation of circulating conjugated dopamine probably does not occur and, therefore, cannot account for the free dopamine in the urine. Intact afferent vagal pathways have been shown to mediate the increase in renal dopamine production during acute volume expansion with isotonic saline. However, renal dopaminergic nerves contribute less than 30% of renal dopamine production. Moreover, renal denervation does not prevent the dopaminergic-mediated increase in sodium excretion associated with volume expansion with isotonic saline.
The synthesis of dopamine differs between renal tubular and neural cells. Neural cells express tyrosine hydroxylase (TH), which converts tyrosine to L-3,4-dihydroxyphenylalanine (L-DOPA) that is subsequently decarboxylated to dopamine by aromatic acid decarboxylase (AADC). Non-neural tissues, such as the renal tubules ( Figure 19.1 ), do not express TH, and therefore cannot synthesize L-DOPA. Rather, L-DOPA is taken up intracellularly by renal tubule cells and converted to dopamine by AADC, which is expressed in many non-neuronal tissues, such as immune cells, kidney, liver, adrenal, spleen, pancreas, and thyroid, among others. There is sexual dimorphism in the levels of AADC in mice; AADC protein expression and activity are higher in the kidneys of females than in males, while the converse is true in the intestines.

Renal dopamine production is regulated by several factors, including the availability and renal tubular uptake of L-DOPA, activity of AADC, transport of dopamine from inside the proximal tubule cell into the tubular lumen and perivascular space, and metabolism of dopamine. However, sodium intake and intracellular sodium are probably the major stimuli in the renal tubular synthesis/release of dopamine in normal adults.
Sources of L-DOPA
As previously mentioned, the major source of renal dopamine production is the renal tubular decarboxylation of L-DOPA taken up by renal tubule cells from the glomerular filtrate and circulation. Plasma L-DOPA, which is about 2 μM, reflects catecholamine turnover from sympathetically innervated tissues, such as skeletal muscle, heart, adrenal gland, and gut. There are non-neuronal sources of plasma L-DOPA, because patients with pure autonomic failure have normal plasma levels of L-DOPA. Indeed, L-DOPA is produced by non-neuronal cells that express TH in mesenteric organs (gastrointestinal tract, spleen, and pancreas ). Plasma L-DOPA sulfate levels increase after meals, possibly related to the amount of DOPA in the food. Inhibition of the decarboxylation of L-DOPA during a protein meal doubles its plasma levels, indicating that normally a protein meal-induced increase in plasma L-DOPA is immediately decarboxylated. Tyrosinase activation, decreased degradation of L-DOPA, and renal demethylation of 3-O-methyl DOPA may be additional sources of urinary dopamine.
Renal Tubular Uptake of L-DOPA
As already indicated, the rate-limiting step in the synthesis of dopamine by the kidney is the uptake of L-DOPA by renal tubule cells from the circulation and glomerular filtrate. The conversion of L-DOPA to dopamine occurs mainly in the proximal tubule, since AADC activity is highest in this nephron segment, although AADC is also present in more distal nephron segments. L-DOPA is taken up by the renal tubules via organic cation transporters located at both apical and basolateral membranes. These include sodium-dependent amino acid transporters, L-amino acid transporter-2 (LAT-2), and related to b0,+ amino acid transporter (rBAT), sodium-dependent L-amino acid transporters, ASCT2 and B0AT1, and as yet unidentified transporters. rBAT is predominantly expressed at the apical membrane, while LAT-2 is expressed at both apical and basolateral membranes. In normotensive rats, LAT-2 and rBAT may account for more than 50% of the L-DOPA uptake in renal proximal tubules, while ASCT2 and B0AT1 may account for the rest. In contrast in SHRs, 50% of L-DOPA uptake occurs via LAT-1, 25% via LAT-2, and 25% via ASCT2 and B0AT1. Aging is associated with a decreased tubular uptake of L-DOPA resulting in a decrease in urinary dopamine production, the mechanism of which has not been determined.
Aromatic Amino Acid Decarboxylase (AADC) Activity
Renal AADC activity is dependent on L-DOPA concentration and not on its Km. However, the increase in urinary dopamine in sodium-replete states may be caused, in part, by increased AADC activity. Patients with AADC deficiency have normal or increased levels of urinary dopamine that may be explained, in part, via tyramine hydroxylation by renal CYP2D6. Hypertonic saline can increase the expression of AADC, that is mediated, in part, by TonEBP.
Dopamine Transporters
The accumulation of monoamines from the cytoplasm to storage organelles and their secretion are dependent on vesicular monoamine transporters 1 and 2 (VMAT-1, VMAT-2). VMAT-2 is expressed in neurons and certain neuroendocrine cells, while VMAT-1 is expressed in endocrine and rat renal proximal tubule cells. VMAT-1 may participate in the dopaminergic regulation of renal ion transport, because renal tubular VMAT-1 mRNA and protein expression are increased by a high sodium diet. However, dopamine synthesized in renal tubules is not stored, but is transported both to the basolateral membrane and into the tubular lumen, where it acts on its receptors locally. Dopamine is secreted into the basolateral space via organic acid cationic transporters, while dopamine probably diffuses into the lumen where it is concentrated, reaching high nanomolar to low micromolar concentrations (see above), as a result of water reabsorption and can stimulate its receptors in more distal nephron segments.
Dopamine Metabolism
Dopamine that is synthesized in the renal tubule cells is probably not converted to norepinephrine or epinephrine. Dopamine β-hydroxylase (DBH) mRNA is not expressed in bovine or rat kidneys. However, DBH is expressed in adrenergic nerves supplying the kidney, and inhibition of DBH increases renal dopamine concentration three-fold. In tissues expressing phenylethanolamine- N -methyl transferase (PNMT) (e.g., adrenal medulla, heart, and some areas of the brain) norepinephrine is converted to epinephrine ( Figure 19.1 ). PNMT is also not expressed in human renal proximal tubules, but is expressed in glomeruli, renal distal tubules, and endothelial cells. Non-specific NMT, which is expressed in non-neuronal tissues including the kidney, enables the synthesis of epinephrine even after denervation. More recent studies have also shown that TH and DBH are expressed in mesangial and renal proximal tubule cells in culture, in a pig renal tubular cell line (LLCPK) with proximal tubule and distal tubule characteristics, in a canine distal tubular cell line, MDCK, and mouse inner medullary collecting duct cells. Because TH is not expressed in renal proximal tubules, the presence of TH in LLCPK could be indicative of its distal tubular characteristics. Although dopamine may have access to non-specific NMT, the increase in urinary dopamine with salt loading is not accompanied by an increase in urinary norepinephrine or epinephrine.
In renal tubules, dopamine is degraded both by deamination via monoamine oxidase (MAO) to 3,4-dihydroxyphenylacetic acid (DOPAC), and by methylation via COMT to 3-methoxytyramine. Newly formed dopamine is metabolized predominantly by MAO-A rather than MAO-B, especially in the proximal tubule. In contrast, dopamine is metabolized by COMT in more distal nephron segments. A novel flavin adenine dinucleotide-dependent amine oxidase named renalase or MAO-C has been identified in the kidney, but its role in the intrarenal metabolism of renal dopamine is unknown. Acute inhibition of COMT activity may be more important than MAO in the short-term, while the converse is true for chronic inhibition. The inhibition of dopamine degrading enzymes can increase its concentration. However, the increase in urinary dopamine with high salt intake is probably not related to any decrease in dopamine degradation.
Dietary Influence Including Salt Intake
Feeding increases urinary dopamine that have been related to the amount of sodium in the diet. Most studies have shown that a low sodium intake is associated with low urinary dopamine, while the converse occurs with a high sodium diet. This could be due to an increased spillover of L-DOPA into the arterial blood, as ingestion of food/protein which contains sodium causes an increase in circulating L-DOPA. However, plasma L-DOPA levels may not be increased by a high sodium diet. Even if circulating L-DOPA is not increased by salt intake, the increase in urinary dopamine with salt loading is associated with an increase in urinary excretion of L-DOPA. Therefore, the increase in urinary excretion of dopamine appears to be secondary to an increase in the uptake of L-DOPA by renal proximal tubules, presumably from the glomerular filtrate and circulation. There may also be a mechanism by which L-DOPA is delivered to the kidney independent of circulating L-DOPA (e.g., via the adrenal gland).
Chloride may be more important than sodium in the regulation of renal dopamine production, because an increase in the intake of chloride without sodium increases urinary dopamine, while sodium bicarbonate does not. An increase in the intake of phosphate or calcium also increases renal dopamine production. Blockade of N-type, but not L-type, calcium channels can decrease urinary dopamine excretion.
An important factor in the increase in urinary dopamine caused by sodium loading is the preferential egress of dopamine into the renal tubular lumen, rather than into the interstitium. In LLC-PK1 cells dopamine egresses at the apical surface by a non-saturable process, while its egress at the basolateral surface is saturable, with the latter 5–7-fold higher than the former. The egress of dopamine in LLC-PK1 is probably not related to VMAT-1, because the egress of dopamine is not inhibited by dopamine transporter inhibitors. However, chronic sodium loading increases the expression of the VMAT-1 in the renal proximal tubule, which may contribute to the modification of the polarity of dopamine secretion during sodium loading. Inhibition of sodium hydrogen exchanger (NHE) activity decreases efflux of dopamine into the peritubular space, which presumably favors luminal outflow. Thus, dopamine, by inhibiting proximal tubular luminal NHE activity, may facilitate its own egress into the tubular lumen. The preferential luminal efflux and tubular fluid reabsorption result in high nanomolar to low micromolar concentrations of dopamine in the tubular lumen, concentrations that approach the EC 50 of dopamine and D 1 -like receptor agonists to stimulate adenylyl cyclase and phospholipase C activity. This mechanism, and the fact that dopamine can also be synthesized in more distal nephron segments, albeit to a lower extent relative to the proximal tubule, enables dopamine to stimulate its receptors in more distal nephron segments.
There may not always be a relationship among dietary sodium, urinary dopamine, and sodium excretion. Mühlbauer and colleagues have claimed that food intake rather than sodium is responsible for the increase in urinary dopamine. However, all the acute saline loading studies were performed in rats in which food, but not water, was withheld for 24 hours. Differences in the amount and duration of the sodium load in the food, as well as differences in the strains of rats studied (see below) are possible explanations. In humans, increasing sodium intake from 20 to >200 mmol/day increases renal dopamine production that peaks by the second day, followed by a gradual decline to 50% of the peak value by the 5th day. In rats, sodium chloride loading maximally increases dopamine excretion on the first few days, with the excretion decreasing close to control levels at 1 week, only to gradually increase again from 2 to 4 weeks. Renal dopamine concentrations are not higher in non-salt loaded rats than in rats loaded with salt for 6 weeks. There may also be an interaction between sodium and other substances in food, e.g., protein. Ingestion of food with very low sodium content (<0.02% sodium) is not associated with an increase in sodium excretion (unpublished data).
The renal production of dopamine is strain-dependent in rodents. Urine dopamine is lower in Sprague-Dawley than in Dahl salt-sensitive or -resistant rats or in WKY and SHRs. A strain of WKY rat increases urinary dopamine after 24 hours of salt loading at 4 weeks, but not at 12 weeks of age. Uninephrectomy increases urinary dopamine in Wistar-Han from Harlan, but not from Charles-Rivers or WKY rats from Harlan. The increase in urinary dopamine with chronic sodium chloride loading is less in the salt-sensitive C57BL/6 than the salt-resistant SJL mice from Jackson Laboratory.
The renal production of dopamine is also age-dependent. Renal dopamine synthesis increases with development. However, in contrast to the stimulatory effect of sodium intake on dopamine excretion in normal adult humans, in pre-term infants sodium supplementation prevents, while sodium restriction increases, urinary dopamine ; the significance of this in terms of sodium balance remains to be determined. The stimulatory effect of increased dietary sodium on renal dopamine production and ability to excrete salt load are diminished with aging. Urinary dopamine excretion is also decreased in patients with chronic renal failure, but due to loss of nephron number rather than an inability to synthesize dopamine.
Gender may influence the urinary dopaminergic response to a sodium load. For example, sodium loading has been reported to increase urinary dopamine excretion in Chinese females, but not Chinese males. Ethnic differences in the relationship between urine dopamine and sodium have been reported. The blunted increase in urinary dopamine in response to acute volume expansion in blacks may be caused by reduced decarboxylation of L-DOPA.
Patients with type 1 or type 2 diabetes have decreased basal urinary dopamine, as well as a deficient response to high sodium chloride diet or infusion. Decreased dopamine production in human adults and children with type 2 diabetes may affect the ability to excrete a sodium load. Rats with type 1 diabetes also have decreased renal dopamine production.
Patients with salt-sensitive, low renin or non-modulating hypertension, and some with normal renin hypertension have a blunted increase in urinary dopamine in response to salt loading, despite higher rates of L-DOPA excretion. Protein intake or high dietary sodium intake may not increase urinary dopamine in essential hypertensive patients or even normotensive subjects with a family history of hypertension. However, salt-sensitive and normal renin essential hypertensives have normal basal urinary dopamine. Mild exercise has been reported to increase renal dopamine production in Stage 1 hypertensive subjects. Borderline and young hypertensives have higher basal urinary dopamine than control subjects or stable hypertensive patients, suggesting that the renal dopaminergic system may act as an early defense against hypertension that fails during its progression.
There are also abnormalities in renal dopamine production in animal models of genetic hypertension. Renal dopamine production may be decreased in adult spontaneously hypertensive and Dahl salt-sensitive rats relative to their normotensive controls, the WKY and Dahl salt-resistant rat, respectively. However, urinary free dopamine and renal tissue dopamine are actually increased in young SHRs in comparison with WKY rats, but the difference disappears at age 16 weeks. In the young Dahl salt-sensitive rat, urinary dopamine is normal and increases with salt intake, as in Dahl salt-resistant rats, although kidney levels of dopamine are lower in Dahl salt-sensitive than in salt-resistant rats on a high salt diet. In adult Dahl salt-sensitive rats, the urinary excretion of dopamine is not increased or may even be decreased in response to acute volume expansion or salt loading. Mice deficient of D 2 dopamine receptors have salt-sensitive hypertension and decreased renal dopamine production.
Dopamine Receptor Subtypes
Dopamine receptors probably evolved from a common ancient ancestor over 750 million years ago, before the divergence of vertebrates and invertebrates. Dopamine receptors belong to the α group of the rhodopsin-like family of GPCRs, characterized by seven transmembrane domains. Based on their ability to stimulate or inhibit adenylyl cyclase, dopamine receptors have been classified into two families ( Table 19.1 ): D 1 -like receptors stimulate adenylyl cyclases, while D 2 -like receptors inhibit adenylyl cyclases. There are two D 1 -like receptors in eutherian mammals, D 1A and D 1B , also known as D 1 and D 5 in humans; archosaurs have four D 1 -like receptors, D 1A, D 1B , D 1C , and D 1D ; all other jawed vertebrates have three D1-like receptors, D 1A , D 1B , and D 1C . The D 2 -like receptors include D 2 (D 2 R), D 3 (D 3 R), and D 4 (D 4 R). The rank order affinity of dopamine to its receptors is: D 3 R≥D 4 R>D 5 R≥D 2 R>D 1 R.
Dopamine Receptor Subtype | Species | Glomerulus e | Proximal Tubule | TDL, ATL | TAL | DCT | Collecting Duct | Juxtaglomerular Apparatus | Arterial Vessel | |||||
---|---|---|---|---|---|---|---|---|---|---|---|---|---|---|
MC | Podocytes | PCT | PST | CCD | OMCD | IMCD | JGC | MD | ||||||
D 1 R | ||||||||||||||
mRNA | mouse | |||||||||||||
rat | No | No | Yes a ,367 | No | No | Yes | Yes | No | No | Yes | No | Yes | ||
human | ||||||||||||||
Protein | mouse | Yes * | Yes b | No b | Yes b | Yes b | Yes b | Yes b | No b | Yes b | Yes b | Yes b | ||
rat | Yes c No | No | Yes | No | No Yes | Yes | Yes d | No Yes d | No Yes | Yes a , 361 | Yes | Yes | ||
human | No | No | Yes | Yes | Yes | Yes | Yes d | No | No | Yes | ||||
D 2 R | ||||||||||||||
mRNA | mouse | |||||||||||||
rat | Yes | Yes | Yes | Yes | ||||||||||
human | ||||||||||||||
Protein | mouse | No b | No b | Yes b | Yes b | No b | Yes b | Yes b | Yes b | No b | No b | No b | No b | No b |
rat | Yes | No | Yes | No | Yes b | Yes b | Yes | Yes 357, f | No b | Yes b | ||||
human | No b | Yes b | Yes b | Yes b | No b | Yes b | Yes b | Yes b | Yes b | No b | No b | No b | Yes b | |
D 3 R | ||||||||||||||
mRNA | mouse | |||||||||||||
rat | Yes | Yes | Yes | Yes | ||||||||||
human | ||||||||||||||
Protein | mouse | Yes b | Yes b | Yes b | Yes b | Yes b | No b | No b | No b | Yes b | Yes b | |||
rat | Yes g ,375 No | Yes No | Yes | No | No | No | Yes No | Yes No | No | Yes No | No Yes 391, i | Yes No | Yes No | |
human | ||||||||||||||
D 4 R | ||||||||||||||
mRNA | mouse | |||||||||||||
rat | Yes | |||||||||||||
human | ||||||||||||||
Protein | mouse | Yes b | Yes b | No b | No b | Yes b | Yes b | Yes b | Yes b | No b | Yes b | Yes b | ||
rat | No | Yes | No | Yes | Yes | Yes | Yes | No | No | Yes h | ||||
human | ||||||||||||||
D 5 R | ||||||||||||||
mRNA | mouse | Yes * | ||||||||||||
rat | Yes b | Yes b | Yes b | Yes b | ||||||||||
human | ||||||||||||||
Protein | mouse | No | No | Yes | Yes | No | Yes | Yes | Yes | Yes | No | No | No | Yes |
rat | No | Yes | Yes | Yes | Yes d | Yes d | Yes | Yes b | Yes b | |||||
human |
* did not distinguish D1R from D5R
a Yamaguchi I, Jose PA, Mouradian MM, et al. Expression of dopamine D1A receptor gene in proximal tubule of rat kidneys. Am J Physiol. 1993 Feb;264(2 Pt 2):F280–5.
c Not universally expressed in the WKY rat, not expressed in the SHR.
d Not indicated as to location in outer or inner medulla.
e Expressed in the WKY, not expressed in the SHR.
f Expressed in intercalated cells.
h Disappeared with renal denervation.
Based on site-directed mutagenesis and protein modeling of some GPCRs, including D 2 R, the binding of their respective agonist has been suggested to occur at the hydrophobic transmembrane domains, i.e., aspartate residues in transmembrane domains II and III, two serine residues in transmembrane V, and a phenylalanine residue in transmembrane VI. Dopamine receptors have not been crystallized, but studies of the crystallized β2-adrenergic receptor suggest that agonist binding occurs at the extracellular ends of helices III, IV, V, and VII, with the cytosolic ends mediating G-protein activation. Nuclear magnetic resonance spectroscopy has revealed three distinct conformations of the β 2 AR: unliganded receptor or neutral antagonist, inverse agonist, and agonist. The binding pocket of the A 2A adenosine receptor is slightly different, with the binding pocket closer to helices VI and VII, suggesting GPCR specificity.
Dopamine Receptor Signaling
Receptor activation, at least for rhodopsin, occurs by a disruption of the Arg135/Glu134 ionic bond in transmembrane VI resulting in the formation of a new Arg135/Tyr223 interaction in transmembrane V261. This causes the GPCR to function as a guanine nucleotide exchange factor promoting the exchange of GDP with GTP on the Gα-subunit, resulting in the dissociation and release of the Gα-subunit from the Gβγ-subunit. The dissociated G-protein-subunits then initiate the activation or inhibition of downstream effectors. Hydrolysis of GTP by the intrinsic GTPase activity of Gα promotes the reassociation of the G-protein-subunits. RGS (regulators of G-protein signaling) acting as a GTPase-activating protein (GAP), stimulate the hydrolysis of GTP and, therefore, the reassociation of the G-protein-subunits. RGS act as a GAP for GαS, Gαi and Gαq. While a primary function of RGS is to suppress G-protein signaling via GAP activity, RGS have non GAP actions such as direct antagonism of Gα and binding of Gβ/γ. Specific RGS have been reported to regulate dopamine receptors. For example, RGS7 with Gβ5 can regulate dopamine receptor signaling and RGS9-2, but not RGS4, inhibits D 2 R internalization, while RGS19 inhibits D 2 R signaling. RGS4 may be involved in D 1 R signaling. In contrast, RGS2 may be regulated by both D 1 R and D 2 R.
In some instances, agonist specific ligand binding does not cause dissociation of the protein subunits, but rather a conformational change. Heterotrimeric G-proteins can also interact with proteins other than GPCRs to regulate physiological processes. These include activators of G-protein signaling (AGS) which promote guanine nucleotide exchange independent of GPCRs and activate or inhibit adenylyl cyclase or phospholipase C. Dopamine receptors have been reported to decrease AGS1 expression. In addition, GPCR signaling can be independent of G-protein-subunits. For example, the inotropic effect of angiotensin II with occupation of AT 1a R in cardiomyocytes occurs via a Gαq/PKC-independent, but GRK6/β-arrestin 2-dependent, mechanism.
Both D 1 -like receptors, D 1 R and D 5 R couple to Gα S and Gαq. There are also differences in G-protein linkage between D 1 R and D 5 R. For example, the D 1 R, but not D 5 R, also couples to Go and Gα Olf , which is expressed in renal tubules and macula densa. In contrast, D 5 R, but not D 1 R, couples to Gz and Gα 12/13 . Gα 12/13 proteins may have functions different from those ascribed to GPCR activation, including cell movement, actin cytoskeleton, and protein phosphatase 2A activity. Because the recycling of D 1 R is partly under the control of protein phosphatase 2A or a protein phosphatase 2-like enzyme, this may be a mechanism for D 5 R and D 1 R cross-talk.
The linkage of G-protein-subunits to the specific D 1 -like receptor may be tissue-specific. This apparently discordant linkage of D 1 -like receptors to G-protein-subunits can be explained by recent observations that a specific ligand acting on the same receptor recruits specific signaling proteins that elicit a specific phenotype. For example, most D 1 -like receptor agonists stimulate both adenylyl cyclase and phospholipase C. However, there are exceptions; SKF83822 stimulates adenylyl cyclase but not phospholipase C, whereas SKF83959 stimulates phospholipase C but actually inhibits adenylyl cyclase.
Adenylyl Cyclase and Protein Kinase A (PKA)
As indicated above, D 1 -like receptors are linked to stimulation of adenylyl cyclase which leads to the formation of cAMP. There are at least 10 isoforms of adenylyl cyclase (adenylyl cyclases I-IX and adenylyl cyclase short). In the neostriatum, the D 1 R is linked to adenylyl cyclase V. However, adenylyl cyclase V is not expressed in the rat renal proximal tubules (but may be expressed in humans, unpublished observations). In kidney tubule cells, D 1 R is linked to adenylyl cyclase VI, while D 5 R is linked to adenylyl cyclase IV. cAMP binds to the regulatory subunits of PKA resulting in the activation (disinhibition) of its catalytic subunits. There are two PKA isoforms, but D 1 -like receptors, specifically D 1 R, may utilize PKA II rather than PKA I. There are several PKA substrates, among which are dopamine and cAMP-regulated 32 kDa phosphoprotein (DARPP-32) which inhibits protein phosphatase 1. In contrast to the stimulatory effect of the D 1 R/PKA pathway on DARPP-32, the D 1 R/phospholipase C pathway inhibits DARPP-32. The roles of PKA, DARPP-32, and protein-phosphatases in renal sodium transport are discussed below.
Phospholipase C
Phospholipase C catalyzes the formation of diacylglycerol and inositol phosphates. D 1 -like receptors are linked to phospholipase C, via Gαq, independent of adenylyl cyclase, a phenomenon that was initially described in renal cortical tubules. There are several isoforms of phospholipase C; the D 1 R directly stimulates phospholipase Cβ1 in renal cortical but not in medullary membranes, and indirectly stimulates phospholipase Cγ via PKA and PKC, in fibroblasts.
In neuroblastoma cells, the D 1 R is not linked to G q and in neurons, D 1 R-mediated stimulation of phospholipase C may require the presence of D 2 R while D 5 R, by itself, can increase phospholipase C-mediated calcium mobilization that is inhibited by D 2 R. However, in a pituitary adenoma rat cell line (GH4C1) transfected with the D 5 R, the D 5 R activation actually decreases inositol phosphate production. The report that D 1 -like receptor-stimulated phospholipase C persists in D 1 −/− mice has been taken to indicate that there is a D 1 -like receptor other than D 1 R that can stimulate phospholipase C. This is indeed the case, as the D 5 R is also linked to phospholipase C activation in neural tissue (hippocampus, cortex, and striatum).
Phospholipase D
Phospholipase D (PLD) hydrolyzes phospholipids, such as phosphatidylcholine, to form phosphatidic acid and the free polar head group of the phospholipid substrate. Phosphatidic acid can be cleaved by phosphatidic acid phosphohydrolase to produce diacylglycerol; both are important second messengers in the “late” response of cells to certain stimuli. The two mammalian isoforms of PLD (PLD1 and PLD2) have ~50% identity, and are distributed widely in mammalian tissues and cells. They are believed to play an important role in the regulation of cell function and cell fate by a variety of extracellular signals. D 5 R inhibits PLD2 activity in renal proximal tubule cells, while both D 1 R and D 5 R can inhibit phospholipase D activity in vascular smooth muscle cells. These actions lead to inhibition of the production of reactive oxygen species (ROS). The D 3 R heterologously expressed in HEK293 cells has been reported to activate PLD, via Rho and independent of Gi/Go. The relationship between PLD and endogenously expressed D 3 R remains to be determined.
Protein Kinase C
Protein kinase C (PKC) isoforms are classified into three groups: (1) conventional PKCs (cPKC-α, -βI, -βII, and -γ); (2) novel PKCs (nPKC-δ, -ε, -θ, and -η/G); and (3) atypical PKCs (aPKC-λ(ι) and -ξ) and PKCμ, also called PKD which consists of PKD1, PKD2, and PKD3. In renal tubule cells, D 1 -like receptors can stimulate PKCθ and PKCξ, and inhibit PKCδ. The D 1 R increases the phosphorylation of PKCθ S676 in human embryonic kidney (HEK293) cells. D 1 -like receptors can also translocate specific PKC isoforms. Thus, D 1 -like receptors translocate conventional (α, β) and novel (ε) PKCs from renal tubule cytosol into membranes, and the novel PKC-δ from membranes to cytosol. Some studies have shown that the stimulation of PKC by dopamine may be secondary to PKA activation.
While PKA and PKC mediate the actions of D 1 R, both can also regulate D 1 R signaling. PKC activation can produce differential D 1 R and D 5 R signaling. PKC can increase the membrane expression of D 1 R in vascular smooth muscle cells. In HEK293 cells, novel PKC isoforms stimulate D 1 R signaling, but inhibit D 5 R signaling. However, in HEK293T cells, PKCα, β1, γ, δ, and ε constitutively phosphorylate and dampen D 1 R signaling. Ethanol enhances D 1 R signaling by inhibiting PKCγ and PKCδ. PKA and PKC have been reported to phosphorylate D 1 R, modulating the rate of agonist-induced D 1 R desensitization and intracellular trafficking. RanBP9 and RanBP10 interact with both PKCδ/γ to increase the phosphorylation and desensitization of D 1 R in neural and renal proximal tubule cells. RanBP9 and RanBP10 may function as scaffolding proteins to regulate the spatial and temporal organization of D 1 R and PKC. In renal proximal tubule cells, NHERF-1 is necessary for the D 1 R-mediated stimulation of cAMP accumulation and PKC activity.
D 2 -Like Receptors
The D 2 -like receptors couple to G-proteins Gα i and G o , and inhibit adenylyl cyclases.
There are two isoforms of D 2 R; postsynaptic D 2 R effects are mediated by the long isoform, D 2L R, while the presynaptic D 2 R effects are mediated by the short isoform, D 2S R. The D 2S R is localized at the plasma membrane, while the D 2L R is localized in the perinuclear region in several cell lines heterologously expressing D 2S R and D 2L R. However, in renal proximal tubule cells that express the D 2L R rather than the D 2S R, the D 2L R is expressed mainly at the plasma membrane. The particular G-protein-subunit that interacts specifically with either D 2 R isoform is not clear. The D 2 R can couple to the same extent to Gαi (Gαi2 and Gαi3) and Gz, but Gαo may be the major G-protein that is activated by D 2S R and D 2L R ; neither D 2S R nor D 2L R subtype is linked to Gq11 or Gα 12/13 . A splice variant of Gαi2, sGα i2 , is important in the intracellular localization of D 2 R, and its dissociation from D 2 R following D 2 R agonist stimulation increases cell surface D 2 R expression.
The D 4 R, like the D 2 R, couples to Gαi (Gα i2 and Gα i3 ), Gαo, Gz, and Gαt2. Different numbers of 16 amino acid repeats in the third cytoplasmic loop cause several human D 4 R isoforms (e.g., D4-2, D4-4, and D4-7). The role of these D 4 R isoforms in cell function remains to be determined. However, the D 4 R long (at least one 7 to 10 repeat) has been reported to be associated with higher diastolic and systolic blood pressure.
The D 3 R linkage to Gα i is not robust, in contrast to that observed for D 2 R and D 4 R. In rat renal proximal tubule cells the D 3 R stimulation of phospholipase C is via Gα i3 . The signaling of D 3 R, like the D 2 R and D 4 R, may be mediated by Gαo. The utilization of G-proteins in D 2 R and D 3 R-mediated stimulation of extracellular signal-regulated kinase differs, D 2 R utilizes Gα i while D 3 R utilizes Gα/ο and the βγ-subunit of G i . The D 3 R also couples to Gz and, in the presence of pertussis toxin, the D 3 R can also couple to Gαs. The D 3 R can also couple to Gq11. The linkage of the D 3 R to other effectors, such as inhibition of K + and Ca 2+ , may be more sensitive than its weak linkage to G-proteins. The D 3 R is also insensitive to GTP, relative to the other dopamine receptor subtypes. There could be seven distinct alternatively spliced D 3 R variants. The full-length D 3 R and a shorter receptor isoform, the D 3 S, bind to dopamine. The five other alternatively spliced D 3 R variants do not bind dopamine, but one of them, D 3 Rnf, regulates receptor dimerization.
Adenylyl Cyclase
Although D 2 -like receptors inhibit adenylyl cyclase activity, in many cell lines heterologously expressing the D 3 R, its linkage to Gα i is not robust. However, the D 3 R robustly inhibits adenylyl cyclase isoform V, but because this adenylyl cyclase isoform is not expressed in the rat renal proximal tubule, it is expressed in human renal proximal tubule cells (unpublished data). D 3 R action in this rat nephron segment is not due to Gα i signaling. Because D 3 R can couple to Gαs in the presence of pertussis toxin, the D 3 R could increase adenylyl cyclase activity in this nephron segment in the rat. This has not been directly tested, but D 1 R and D 3 R dimerization increases the ability of dopamine to stimulate adenylyl cyclase activity. In contrast, the co-expression of D 2 R with D 3 R increases the ability of D 2 -like agonists to inhibit adenylyl cyclase activity. This becomes a conundrum in cells expressing these dopamine receptor subtypes, but at least in renal proximal tubules there is no evidence of D 1 R and D 2 R or D 2 R and D 3 R heterodimerization (unpublished data). However, in the kidney and vascular smooth muscle D 1 R and D 3 R do interact, and this interaction probably predominates over the other possible D 1 -like and D 2 -like interactions. While D 2 R, by itself, stimulates Na + K + -ATPase activity, simultaneous stimulation of D 1 -like and other D 2 -like receptors inhibits Na + K + -ATPase activity. Furthermore, D 2L R and D 4.4 R can potentiate PKC-stimulated adenylyl cyclase II activity through the release of β/γ-subunits, and D 2L R can sensitize adenylyl cyclase VI. Although these adenylyl cyclase isoforms are expressed in renal proximal tubule cells, this interaction probably does not occur in renal proximal tubule cells because quinpirole, a D 2 -like receptor agonist with preference for the D 3 R and D 4 R over the D 2 R, does not stimulate adenylyl cyclase activity (unpublished studies). As stated above, there are species differences because adenylyl cyclase V is expressed in the human (unpublished data) but not in the rat renal proximal tubule.
Phospholipase C
In neural cells, D 2 R, via Gβ/γ, stimulates phospholipase C and specifically phospholipase Cβ4 in the intermediate lobe of the pituitary gland . D 1 R and D 2 R can interact to stimulate phospholipase C in neural cells, but as stated above there is no evidence for D 2 R and D 3 R interaction in renal proximal tubule cells. D 2 R and D 5 R can also heterodimerize to stimulate phospholipase C in neural tissue and HEK-293 cells. The D 2S R can also stimulate phospholipase D, but the latter enzyme is inhibited by D 5 R. These effects need not be counter-regulatory, because as previously mentioned the D 2S R is presynaptic, while the D 5 R inhibition of phospholipase D is postsynaptic in renal proximal tubule cells (in particular). In addition, presynaptic inhibition of adrenergic neurotransmitters by D 2S R facilitates the inhibitory effects of dopamine on ion transport.
Dopamine Regulation of Ion Channels
D 1 -like receptors inhibit voltage-gated K + channels and G-protein-regulated inward rectifying channels, but stimulate L-type Ca 2+ channels. D 1 -like receptors inhibit G-protein-dependent inward rectifier K-like channels in medial prefrontal cortex via PKC. In contrast, D 2 -like receptors stimulate voltage-gated K + channels and G-protein-regulated inward rectifying channels, but inhibit L-type Ca 2+ channels. Both D 1 -like and D 2 -like receptors inhibit N-, P-, and Q-type Ca 2+ channels and transient sodium channels, but stimulate persistent P sodium channels. We have reported that the D 3 R-mediated vasorelaxation of rat mesenteric arteries is due to stimulation of small- and/or large-Ca 2+ -activated K + channels. In human coronary vascular smooth muscle cells, D 5 R stimulates Ca 2+ -activated big K + channel, via cAMP-mediated stimulation of PKG.
Dopamine Regulation of Mitogen-Activated Protein (MAP) Kinase and other Kinases
D 1 -like receptors may activate MAP kinases, including p38MAP kinase, and c-jun amino-terminal kinase. In the brain, D 1 -like receptors can also activate ERK, but ERK1/2 is actually inhibited by D 5 R but not by D 1 R in human renal proximal tubule cells. D 2 -like receptors can also activate MAP kinase via Gβ/γ. D 2 -like receptor-mediated stimulation of Na + K + -ATPase in the kidney is linked to the p44/42 MAP kinase pathway. Aberrant D 1 R activation of ERK1/2/MAP kinase has been reported in D 1 R supersensitivity. However, in the kidney, MAP kinase reduces D 1 R affinity and G-protein coupling.
Dopamine inhibits DNA synthesis of cultured human mesangial cells. In rat vascular smooth muscle cells, D 1 R or D 3 R does not affect vascular smooth muscle cell proliferation, but inhibits the proliferative effect of insulin and norepinephrine. D 2 R, via inhibition of MAP kinase, inhibits angiotensin II-induced hypertrophy of cultured neonatal rat ventricular myocytes. However, dopamine increases the phosphorylation of and activates p44/42 MAP kinases in rabbit renal proximal tubule cells in primary culture. In human renal proximal tubule cells and Chinese hamster ovary cells, the D 3 R promotes mitogenesis or cell proliferation through the activation of MAP kinases. The variable effects of dopamine on MAP kinase in smooth muscle and epithelial cells may be related to tissue- or cell-specificity.
DARPP-32
As stated above, DARPP-32 was identified initially as a major target for dopamine-activated adenylyl cyclase in striatum. Since the mid-1980s, DARPP-32 has been acknowledged as a crucial mediator of the biochemical, electrophysiological, transcriptional, and behavioral effects of dopamine. The state of DARPP-32 phosphorylation has been shown to provide a mechanism for integrating information arriving at dopaminoceptive neurons, in multiple brain regions, via a variety of neurotransmitters, neuromodulators, neuropeptides, and steroid hormones. Activation of PKA or PKG stimulates DARPP-32 phosphorylation at Thr, and thereby converts DARPP-32 into a potent inhibitor of protein phosphatase-1 (PP1). Protein phosphatase-2B (PP2B) is the most effective protein phosphatase in dephosphorylating DARPP-32 at Thr. Thus, DARPP-32 acts as an amplifier of PKA and PKG-mediated signaling when it is phosphorylated on Thr, which converts it into an inhibitor of PP1. The role of DARPP-32 in signaling has turned out to be very complex. Under basal conditions, DARPP-32 is phosphorylated at Thr and inhibits PKA. Thus, DARPP-32 has the unique property of acting either as an inhibitor of PP1 or an inhibitor of PKA. However, under hyperdopaminergic conditions, the phosphorylated state of Thr is reduced, allowing increased phosphorylation at Thr. This positive feedback loop acts as a switch to potentiate dopaminergic signaling. Cdk5, a cyclin-dependent kinase family member, also phosphorylates DARPP-32 at Thr. Furthermore, protein phosphatase 2A dephosphorylates DARPP-32 both at Thr and Thr, and the state of phosphorylation of DARPP-32 at Thr depends on the phosphorylation state of two serine residues, Ser and Ser. DARPP-32 has been demonstrated with biochemical, immunohistochemical, and in situ hybridization techniques to have an anatomical distribution similar to that of dopaminoceptive cells possessing D 1 Rs in the central nervous system. DARPP-32 is also expressed in several peripheral tissues, including brown fat cells, parathyroid, retina, and renal tissue. The ability of D 1 R to stimulate protein phosphatase 2A may serve as a negative feedback in the D 1 R and DARPP-32 relationship, and prevent the inhibitory effect of DARPP-32 on PKA.
Regulation of Dopamine Receptor Function
Effect of Sodium on Dopamine Receptor Expression
While acute sodium loading generally increases renal dopamine production, it may decrease brain and renal D 1 -like and D 2 -like receptor density. However, the decrease in renal D 1 -like receptor expression occurs after 7–28 days of increased sodium intake during peak renal dopamine production. In C57BL/6 mice, increasing NaCl diet to 6% for 5–7 days decreases renal D 1 R expression, but increases renal D 3 R expression without affecting the renal expression of D 2 R, D 4 R or D 5 R (unpublished observations).
Dopamine Receptor Recycling
As with other GPCRs, dopamine receptor signal transduction is precisely regulated. In the basal state, in human renal tubules, human D 1 Rs exist as homo-oligomers in lipid raft microdomains. Occupation of D 1 Rs increases the amount of D 1 R monomers associated with a transient increase in D 1 R function, as a result of the recruitment of D 1 R from an intracellular pool of D 1 R to the lipid rafts of plasma membranes. 215, Following the transduction of the GPCR signals, there is a transient loss of receptor responsiveness (desensitization). This mechanism dampens short-term agonist effects following repeated agonist exposure. At least three families of regulatory molecules contribute to GPCR desensitization: second messenger-dependent protein kinase; G-protein-coupled receptor kinases (GRKs); and arrestins. Homologous desensitization, in response to agonist stimulation, occurs via action of a member(s) of the GRK family. Heterologous desensitization, mediated by second messenger-dependent kinases, occurs when a decrease in receptor responsiveness is induced by a ligand other than its own specific ligand. The phosphorylation of GPCRs, including the D 1 R, leads to the binding of a member(s) of the arrestin family, uncoupling of the receptor from its G-protein complex, and a decrease in its functional response. Desensitization of GPCRs involves phosphorylation, sequestration/internalization, and degradation of receptors. The D 1 R (but not D 5 R), expressed endogenously in renal proximal tubule cells, is regulated to a lesser extent by GRK2 and to a greater extent by GRK4 in human kidneys, but the converse may be true in rat kidneys. Moreover, GRK4 may constitutively phosphorylate D 1 R. GRK3 regulates rat D 1 R overexpressed in HEK293 cells. It is not clear whether or not GRK5 regulates the rat D 1 R. GRK6 is not important in the regulation of D 1 R in the kidney (unpublished), but it is important in the desensitization of the D 1 R in intestinal crypt cells, emphasizing the importance of cell type in D 1 R regulation. The desensitization of D 5 R, in contrast, does not involve GRKs, but rather sorting nexin 1. Similarly, GRK is not involved in the first 20 minutes of D 1 R desensitization in human renal proximal tubule cells, but could be related to sorting nexins (unpublished data). It has also been suggested that GRK2 may negatively regulate D 2 R signaling by a phosphorylation-independent mechanism.
Heterologously expressed D 1 Rs, after ligand stimulation, rapidly (but not completely) internalize (5–12 minutes) in cell lines (HEK-293 and Neuro2A neuroblastoma cells). In HEK-293 cells heterologously expressing rat D 1 R, 15 minutes of agonist stimulation does not completely desensitize D 1 R. Presumably, the internalized D 1 R continues to function until it is completely desensitized. Phosphorylation, desensitization, and internalization cannot be directly equated. GPCR desensitization cannot be completely explained by phosphorylation; ubiquitination may also play a role. AT 1 R, D 1 R, and D 5 R (unpublished data) are ubiquitinated within the first 15 minutes following ligand stimulation; AT 1 R and D 5 R, but not D 1 R, are targeted for degradation following ligand occupation. Thus, desensitization may also occur at the plasma membrane. Cell membrane invagination and endocytosis are important in D 1 R trafficking; prevention of endocytosis with hypertonic sucrose abrogates ligand-induced D 1 R desensitization in human renal proximal tubule cells. The phosphorylated GPCR/β-arrestin complex undergoes endocytosis/internalization via clathrin-coated pits into a series of endosomal units, where it is dephosphorylated and recycled back to the plasma membrane or degraded. Dopamine receptors belong to Class A receptors that are rapidly recycled to the plasma membrane. In contrast, AT 1 R receptors belong to Class B receptors that are slowly recycled to the plasma membrane, and some are actually degraded. Protein phosphatases are involved in the resensitization of GPCRs in endosomes and at the plasma membrane. Protein phosphatases, including protein phosphatase 2A (PP2A), a GPCR phosphatase, and PP2B (calcineurin) have been reported to resensitize D 1 R, but PP2A is probably the major protein phosphatase involved in D 1 R function and/or resensitization in renal tubule cells.
The D 2 R, like some GPCRs, is not desensitized by phosphorylation but rather by association with β-arrestin, but phosphorylation is required for its recycling. The GRK regulating D 2 R in renal tubule cells is not known, but D 2 R in other cells is regulated by GRK2, GRK3, GRK5, and GRK6, and constitutively desensitized by GRK2. GRK2 and GRK3 preferentially sequester D 2S R over D 2L R, while β-arrestin 2 has the converse effect. GRK2 or GRK3, but not GRK5 or GRK6, is involved in the desensitization of the calcium signal mediated by the interaction of D 1 R/D 2 R heterologously expressed in HEK293TSA cells. The desensitization of D 3 R is weakly regulated by GRK2 and GRK3, but robustly by GRK4 (GRK4γ>GRK4α); GRK4 and D 3 R colocalize and interact in human renal proximal tubule cells. D 4 R, like some GPCRs, e.g., AT 2 R and β 1 R, may not undergo internalization and is resistant to desensitization. The GRK regulating D 4 R is not clear, but does not seem to involve either GRK2 or GRK3. The GRK regulating D 5 R is also not clear, but does not seem to involve GRK4.
Membrane Microdomains
Cholesterol rich domains in membranes are called lipid rafts and the rest are called non-lipid rafts. Lipid rafts serve as signaling platforms for several signaling molecules such as G-protein-subunits, enzymes, and adaptor proteins. Lipid rafts can affect the function of GPCRs by inducing a conformational change and/or alteration of the physical properties of the membrane in which the GPCR is embedded. We have reported that angiotensin II does not affect the differential expression of AT 1 R in lipid and non-lipid rafts in renal proximal tubule cells from normotensive rats, but increases the amount of glycosylated AT 1 R in lipid rafts in renal proximal tubule cells from SHRs; this may lead to an increased ability of angiotensin II to stimulate the Cl − /HCO 3 − exchanger. D 1 -like receptors also regulate NADPH oxidase activity and subunit expression in lipid microdomains of renal proximal tubule cells. The D 2 -like receptor antagonist, haloperidol, has been reported to disrupt lipid rafts. Interestingly, high doses of simvastatin, a cholesterol depleting reagent, increase dopamine receptor expression in the rat prefrontal cortex. In human renal tubule cells, heterologously or endogenously expressed D 1 R exists as homo-oligomers; agonist stimulation increases the amount of D 1 R monomers consistent with other GPCRs. D 1 R oligomers exist in caveolae-like microdomains; decreasing caveolin expression or depleting cholesterol impairs D 1 R-mediated generation of cAMP and oligomer formation. Renal inhibition of caveolin-1 expression, an important component of lipid rafts, via caveolin-1 siRNA in the kidney impairs D 1 -like receptor-mediated natriuresis in salt-loaded rats and produces hypertension. Thus, the function of GPCRs, including dopamine receptors, can be regulated by cholesterol membrane microdomains.
Regulation of GPCR Membrane Expression
Plasma membrane expression of GPCRs requires proper folding that is aided by chaperones. Homodimerization has been suggested to help in GPCR folding. Indeed, folding efficiency has been suggested to regulate the expression of D 4 R. A proper amount of calnexin, an endoplasmic reticulum chaperone, is important in the proper trafficking of both D 1 R and D 2 R to the plasma membrane.
D 1 R
Dopamine receptor interacting protein 78 (DRiP78) is an endoplasmic reticulum protein that regulates the transport of D 1 R and other GPCRs, including AT 1 R, and the assembly of G-protein β- and γ-subunits. Increased expression of DRiP78 impairs the transport of D 1 R from the endoplasmic reticulum to the plasma membrane. In contrast, DRiP78 increases the plasma membrane expression of AT 1 R, another example of how certain proteins have contrasting effects on AT 1 R, and dopamine receptor trafficking and expression. Neurofilament-M specifically decreases the cell surface membrane expression of D 1 R (not with D 2 -like receptors and weakly with D 5 R). Calcyon is another D 1 R interacting protein that may be important in D 1 R-calcium-mediated signaling in neural tissue. Whether these proteins regulate D 1 R endogenously expressed in renal tissue remains to be determined. However, RanBP9/10 which colocalizes with D 1 R in renal and neural tissues, phosphorylates and impairs D 1 R function.
D 2 R
Cbl-interacting protein of 85 kDa (CIN85) may be important in the trafficking of D 2 R, at least in striatal tissue. Absence of striatal CIN85 is associated with decreased endocytosis of D 2 R and implicated in the hyperactivity of CIN85-deficient mice. A Ca 2+ -binding protein, S100B, increases the ability of D 2 R to inhibit adenylyl cyclase and stimulate ERK activity. Two D 2 R interacting proteins decrease the expression and/or function of D 2 R, including neuronal calcium sensor 1 and protein kinase Cξ-interacting protein. In neural cells, a spliced variant of the Gαi2 may also be important in the plasma membrane expression of the D 2 R and protein 4.1N for both D 2 R and D 3 R.
D 3 R
The D 3 R has been reported to interact with proteins that affect plasma membrane localization. Filamin-A interacts with D 2 R and D 3 R, linking them to the cytoskeleton and increasing their plasma membrane localization. In contrast, paralemmin specifically interacts with the D 3 R decreasing its localization to the plasma membrane. The role of these interacting proteins on D 3 R function in renal proximal tubules or blood vessels remains to be determined.
D 4 R
KLHL12, which can act as a ubiquitin ligase, interacts with the D 4 R, but its ubiquitination does not result in its degradation. Indeed, the D 4 R is resistant to agonist-induced internalization and degradation.
D 5 R
The D 5 R, but not the D 1 R, requires N-glycosylation for membrane localization in transfected HEK-293 cells. However, it does not contribute to the radioligand-binding properties of D 5 R.
Renal Distribution of Dopamine Receptors
The expression of the dopamine receptors in the kidney ( Table 19.1 ) of several mammals has been reported.
Tubular Distribution
The dopamine receptor subtypes are differentially expressed along the mammalian nephron. However, their expression in the medulla is species-dependent. In the rat, the D 2 R and D 4 R are expressed in the inner medulla ( Table 19.1 ). The mouse kidney has no dopamine receptors in the inner medulla (unpublished observations). High concentration of dopamine (100 μM) stimulates prostaglandin E2 production in the inner medulla and thin limb of Henle. The dopamine receptor subtype expressed in the thin limb of Henle is not known, although dopamine (100 μM) stimulates prostaglandin E2 production in rat thin ascending limb cells .
Immunohistochemical studies in human and rodent kidneys have shown expression of D 1 R in the apical and basolateral membranes, as well as in the cytoplasm of proximal tubules (mouse:S3>S1=S2), distal convoluted tubules, medullary thick ascending limb (mTAL) of Henle, macula densa, and cortical collecting duct. The presence of D 1 R in the rat outer or inner medulla has not been shown consistently using immunohistochemistry or in situ mRNA amplification, and dopamine does not stimulate cAMP production via dopamine receptors, in this nephron segment. Moreover, there is no D 1 -like receptor radioligand-binding in the rat inner or outer medulla. The human and mouse kidney (unpublished observations), do not express dopamine receptors in the inner medulla.
D 2L R mRNA is expressed in the rat cortex, and outer and inner medulla. Immunoreactive D 2 R is present in rat and human (unpublished observations) proximal tubule (mouse:S2>S1/S3), distal convoluted tubule, and cortical and outer medullary collecting duct. The D 2 R is expressed in the intercalated cells of the medullary collecting duct in the rat, but not in the mouse (unpublished data). D 2 R protein is also expressed in the OK cell, an opossum proximal tubule cell line that also exhibits distal tubular cell characteristics.
D 3 R mRNA is expressed in the rat cortex, and outer medulla and inner medulla. Immunoreactive D 3 R is expressed in rat apical and subapical areas, but not in the basolateral membrane of proximal tubules (S1>>S2/S3) in three reports ; one study also showed immunoreactive D 3 R in the distal convoluted tubule, and two studies showed D 3 R protein in the cortical collecting duct. One of three reports found D 3 R immunostaining in the inner medulla. Immunoreactive D 3 R is present in mouse proximal tubule, thick ascending limb of Henle, and distal convoluted tubule, but not in the cortical collecting or outer and inner medullary collecting ducts ( Table 19.1 ).
D 4 R is present in the rat proximal tubule (S1>S2>S3), distal convoluted tubule, and especially in the cortical and outer and inner medullary collecting ducts, where it is more abundant at the luminal than at the basolateral areas. In the mouse, D 4 R is present in the proximal convoluted tubule (but not proximal straight tubule), thick ascending limb of Henle, and cortical and outer medullary collecting duct (unpublished observations). D 4 R mRNA is expressed in the human kidney, but its expression along the nephron has not been reported.
D 5 R is expressed in the rat and mouse proximal tubule (S1>S2/S3), thick ascending limb, and distal convoluted tubule and cortical and outer medullary collecting duct. The expression of D 5 R protein has not been reported in human kidney, but is expressed in human renal proximal tubule cells in culture, and D 5 R may be expressed preferentially over the D 1 R in the thick ascending limb of Henle and the cortical collecting duct, while the D 1 R is preferentially expressed in the proximal tubule (unpublished data).
Vascular Receptors
D 1 -Like Receptors
The D 5 R may have chemo- and mechano sensory roles in primary cilia of mouse vascular endothelial cells. D 1 Rs, and to a greater extent D 5 Rs, are expressed in the tunica media of rat mesenteric, pial, and renal arteries. They are mainly located postjunctionally, which has been confirmed by insensitivity to chemical sympathectomy. In the rat kidney, D 1 R mRNA is also found in smooth muscle cells of large and small arteries, and afferent and efferent arterioles. In humans D 1 R protein is also present in vascular smooth muscle cells in the large intrarenal arteries, but not in renal veins.
D 2 -Like Receptors
The D 2 -like receptors are expressed in the adventitia and adventitia–media border of arteries. D 2 R and D 4 R, but not D 3 R, are expressed in rat mesenteric and pial arteries. Rat renal arterial branches express D 2 R and D 3 R. Although renal arteries and arterioles in Wistar rats were not found to express D 4 R, a subsequent study in Sprague-Dawley rats showed expression of D 4 R in large segmental, arcuate, interlobar, interlobular, and afferent and efferent arterioles. Bilateral superior cervical ganglionectomy and renal denervation confirmed the prejunctional localization of the D 2 -like receptors, and the location of D 2 -like receptors in the renal artery suggest their prejunctional localization. However, we have reported that D 3 R stimulation relaxes mesenteric arterial rings, independent of the endothelium. The failure of the endothelium to influence the vasorelaxant effect of D 3 R stimulation is in agreement with the absence of D 2 -like receptor expression in the intima. One study could not find D 3 R expression in renal vessels, although another study found D 3 R immunostaining in medial and adventitial layers of renal arteries in agreement with the report of D 3 R mRNA in renal microvessels.
Glomerular Receptors
Radioligand binding and autoradiographic studies using D 1 -like receptor ligands that recognize both D 1 R and D 5 R have failed to detect specific binding in rat and human glomeruli. D 1 R mRNA studied by in situ mRNA amplification is not detected in rat renal glomeruli ( Table 19.1 ). Immunoreactive D 1 R or D 5 R has not been demonstrated in rat or human glomeruli. In rat glomeruli isolated by sieving, two of three studies showed that dopamine, via an uncharacterized dopamine receptor, increased cAMP production. Dopamine increases cGMP, but not cAMP production in isolated dog glomeruli, also obtained by sieving. However, because afferent and efferent arterioles express D 1 R and D 5 R ( Table 19.1 ) it is possible that glomerular preparations that contain arterial vessels may confound the results. Nevertheless, rat glomerular mesangial cells and mouse glomerular podocytes, in culture, express D 1 -like receptors, assessed by an increase in cAMP production in response to dopamine or D 1 -like receptor agonist. Rat glomerular epithelial cells in culture do not increase cAMP production in response to dopamine.
D 2 -like receptors, D 2L R, D 3 R, and D 4 R, are present in rat glomeruli ( Table 19.1 ). Specifically, immunoreactive D 2 R and D 3 R have been reported in rat mesangial cells in one study and in podocytes in another study. However, there are three reports of the absence of D 4 R receptors in rat glomeruli. D 2 -like receptor stimulation decreases cAMP production in rat glomeruli. Dopamine attenuates the contractile response to angiotensin II in rat isolated glomeruli, but the receptor mediating this effect is not known.
Receptors in Juxtaglomerular Apparatus
Rat and mouse (unpublished observations) but not human juxtaglomerular cells, in situ , and rat juxtaglomerular cells in culture express D 1 R ( Table 19.1 ). Rat and mouse (unpublished studies) but not human macula densa cells express D 1 R. Rat but not mouse juxtaglomerular or macula cells express D 5 R. Rat but not mouse (unpublished studies) macula densa cells express D 1 R. D 5 R expression in human juxtaglomerular or macula cells has not been reported.
Rat, mouse, and human (unpublished studies) juxtaglomerular cells, in situ , do not express D 2 R. Mouse juxtaglomerular cells, in situ , express D 3 R ( Table 19.1 ). In contrast, rat juxtaglomerular cells, in situ , also do not express D 3 R or D 4 R, but express D 3 R and D 4 R in culture. Rat macula densa cells were found to express D 3 R in one of two reports. Mouse macula densa cells express D 3 R and D 4 R (unpublished studies). D 2 R is not expressed in human or mouse macula densa cells, but is expressed in rat macula densa (unpublished studies; Table 19.1 ); D 3 R and D 4 R expression has not been studied in human macula densa.
Dopamine and Renal Hemodynamics
D 1 -Like Receptors
Dopamine, administered in low doses intravenously or directly into the renal artery, increases renal blood flow and decreases renal vascular resistance. The renal vasodilatory effect of dopamine is mimicked by D 1 -like receptor agonists and blocked by the D 1 -like receptor antagonists. The contribution of D 1 R and D 5 R in this action remains to be determined. Dopamine and D 1 -like receptor agonists dilate afferent and efferent arterioles to the same extent. However, afferent arterioles are preferentially dilated in the hydronephrotic kidney. Dopamine induces a greater vasodilatory effect in the renal artery than in the coronary artery, mesenteric, in agreement with the receptor density data.
cAMP/PKA is the primary signaling pathway of the renal vasodilatory effect of dopamine via D 1 -like receptors. However, a dopamine-mediated stimulation of PKC is associated with increased expression of D 1 -like receptors that enhances cAMP production in renal vascular smooth muscle cells. However, as stated earlier in HEK293 cells, novel PKC isoforms stimulate D 1 R but inhibit D 5 R signaling, while conventional PKCs and two of the novel PKCs (δ and ε) impair D 1 R signaling. In coronary arteries, the vasorelaxant effect of D 1 -like receptors has also been attributed to PKA/PKG-dependent activation of K + -ATP, calcium-, and voltage-activated K + channels. Prostacyclins may also contribute to dopamine- and D 1 -like receptor-mediated renal vasodilation.
The effect of D 1 -like receptors in the rat aorta and tail artery is complex. Dopamine has been reported to decrease both sodium influx and efflux by inhibition of NHE and Na + /K + -ATPase activity, respectively, in part via PKA, in rat aorta smooth muscle cells. A predominant inhibitory effect on NHE activity would result in a decrease in intracellular sodium and a decrease in vessel tone, while a predominant inhibitory effect on Na + /K + -ATPase activity would result in an increase in intracellular sodium and an increase in vascular tone. The latter situation may explain the apparent vasoconstrictor action of a D 1 -like receptor agonist in the rat tail artery. In the rat tail artery, dopamine and SKF 38393, a D 1 -like receptor agonist, inhibited Na + /K + -ATPase and increased vascular tone, an effect that was associated with activation of phospholipase C. Since the effects were abolished by pertussis toxin, the D 1 -like receptor in the rat tail artery is must be different from the D 1 -like receptor in renal proximal tubules, because in this tissue phospholipase C is pertussis-toxin resistant and linked to Gq. When phospholipase Cβ1 is not expressed, D 1 -like agonists can be indirectly linked to phospholipase Cγ1 via PKA. The effect of dopamine in resistance vessels may not be the same as that in conduit vessels (e.g., aorta) and in the rat tail artery, which may subserve a thermoregulatory function.
D 2 -Like Receptors
Both the D 2S R and D 2L R may act as autoreceptors in certain nerves (GABA transmission), while the D 2S R but not the D 2L R regulates glutamate release. However, the D 2S R but not the D 2L R is involved in presynaptic dopamine transmission, while postsynaptic dopamine transmission is via the D 2L R. The D 3 R but not the D 4 R can also function as an autoreceptor (presumably located in prejunctional areas) and inhibit catecholamine release. Prejunctional D 2 -like receptors in the kidney inhibit norepinephrine release. This effect may explain the ability of bromocriptine (D 2 R and D 3 R agonist), but also with D 1 -like antagonistic properties, to increase renal blood flow in the anesthetized rat, and the renal vasodilatory effect of endogenous dopamine in humans on a low-sodium diet.
The effect of postjunctional D 2 -like receptors on the renal vasculature is inconsistent. When both α- and β-adrenergic receptors are blocked in the dog kidney, the renal vasodilatory effect of dopamine is antagonized by D 1 -like but not by D 2 -like receptor blockade. However, in a similar preparation, bromocriptine has been reported to decrease renal blood flow. In the conscious, chronically instrumented dog on a moderate sodium intake (40 mmol/day), low (picomolar) concentrations of quinpirole, a D 2 -like receptor agonist with selectivity for the D 3 R and D 4 R over the D 2 R, also produces vasoconstriction. This result agrees with the report that D 3 R stimulation constricts renal vessels in volume-loaded rats. In humans, D 2 -like receptor blockade with L-sulpiride does not affect renal plasma flow but potentiates the renal vasoconstrictor effect of NO inhibition with L-NAME. D 4 R stimulation also enhances the contractile response of guinea pig vas deferens.
In contrast to the above observations that D 2 -like receptors induce vasoconstriction, in the preconstricted isolated perfused rat kidney bromocriptine (D 2 R/D 3 R agonist) induces vasodilation via postjunctional D 2 -like receptors. In the norepinephrine- or high-potassium-preconstricted rat mesenteric arterial rings, D 3 R stimulation induces vasorelaxation. The vasodilatory effect of D 3 R is caused by activation of potassium channels (small- and/or large-conductance calcium-activated potassium channels). Although the D 3 R is present in the intima, the vasodilatory effects of the D 1 R and D 3 R agonists are endothelium independent; D 1 R is not expressed in the intima. In humans, the renal vasodilatory effect of D 2 -like receptors is reduced during sodium loading and increased during sodium restriction. This is in contrast to the lack of effect of sodium loading on the renal vasodilatory effect of D 1 -like receptors.
The D 3 R can augment the vasodilatory effect of the D 1 R by mechanisms independent of signal transduction. In embryonic thoracic aortic smooth muscle cells and coronary artery smooth muscle cells, stimulation of the D 1 R increases D 1 R and D 3 R expression. However, the additive vasorelaxant effects of D 1 R and D 3 R could not be explained by the increased expression of those two receptors, because the vasorelaxant effect occurs within minutes following agonist stimulation. Rather, the D 1 R and D 3 R may acutely and physically interact with each other; D 1 R and D 3 R co-immunoprecipitate within minutes after agonist stimulation, consistent with the timeframe of the additive vasodilation effect of D 1 R and D 3 R.
The published studies show that D 1 -like receptors cause renal vasodilation. Stimulation of pre-junctional D 2 -like receptors should cause vasorelaxation, while stimulation of post-junctional D 2 -like receptors can result in either vasodilation or vasoconstriction. With chronic sodium chloride loading, basal reactivity of renal vessels may be enhanced by an increase in levels of endogenous Na + /K + -ATPase inhibitor, e.g., ouabain, and an increase in intracellular sodium and calcium. Ouabain, per se , can decrease renal D 1 R expression and function. Under these conditions, dopamine can further increase intracellular sodium by stimulating NHE1 activity via D 2 -like receptors. The increase in intracellular sodium increases sodium calcium exchanger activity. The increase in intracellular calcium increases vascular reactivity and, thus, dopamine, via D 2 -like receptors which can then elicit vasoconstriction. When renal nerve activity is increased, as seen in renal nerve stimulation, low-sodium diet, hypovolemia or during anesthesia, the vasodilator effect of dopamine occurs via prejunctional D 2 -like receptors, presumably of the D 3 R subtype. In addition, when renal vascular resistance is increased, the D 2 -like receptor effect at postjunctional sites would be that of vasodilation, since D 2 -like receptors inhibit Ca 2+ channels and stimulate K + channels, both of which can lead to vasorelaxation. Under these conditions, a synergistic effect between D 1 – and D 2 -like receptors may become evident. The effect of dopamine on vascular tone may differ between conduit (e.g., aorta) and resistance (e.g., mesenteric and renal arterioles) vessels. The increase in vascular tone produced by D 1 -like receptor agonists in conduit vessels may serve to increase perfusion in downstream vessels dilated by D 1 -like receptors.
Glomerular Filtration
Although low concentrations (nM) of dopamine consistently increase renal blood flow, this is not the case for glomerular filtration rate. Normally, low concentrations of dopamine dilate afferent and efferent arterioles to the same degree, and therefore transglomerular pressure remains unchanged. However, dopamine can ameliorate the reduced glomerular filtration rate caused by amphotericin B, radiocontrast material, and hypovolemic states. This could be a direct effect on glomerular cells, since dopamine can attenuate the contractile effect of angiotensin II in isolated glomeruli. This effect is probably exerted via D 2 -like receptors, because D 1 -like receptors are not expressed in glomerular mesangial cells, in situ . It is only after culture that glomeruli express D 1 -like receptors and their stimulation increases cAMP production. In isolated dog glomeruli, dopamine increases cGMP formation and in isolated rat glomeruli, dopamine decreases adenylyl activity, in keeping with the presence of D 2 -like receptors ( Table 19.1 ). In vivo , D 2 -like receptors can decrease or increase glomerular filtration rate, depending upon the state of renal vascular D 2 -like receptor activation. When the interaction of D 1 – and D 2 -like receptors results in a greater vasodilatory effect on afferent than efferent arterioles, glomerular filtration rate can increase. D 2 -like receptors are involved in the increase in glomerular filtration rate associated with amino acid infusion, an effect that is mediated by renal nerves. The D 2 -like receptor-mediated decrease in renal blood flow is associated with greater constriction of afferent than efferent arterioles, resulting in a greater decrease in glomerular filtration rate than renal blood flow and, therefore, a fall in filtration fraction.
Tubuloglomerular Feedback
Tubuloglomerular feedback describes a mechanism by which changes in distal tubular sodium chloride delivery induce changes in afferent glomerular arteriolar resistance mediated by the adenosine A 1 receptor. Tubuloglomerular feedback is initiated by transport of sodium via sodium potassium 2 chloride transporters type A (NKCC2A) and type B (NKCC2B) at the macula densa. Because dopamine stimulates NKCC2 activity, it would be expected that dopamine should promote the tubuloglomerular feedback. However, dopamine inhibits tubuloglomerular feedback by occupation of luminal D 1 R but not D 2 R on macula densa cells that may be more evident during sodium surfeit. How can this be done when D 1 R stimulates NKCC2? There are several possible explanations. D 1 -like receptors counteract the effect of adenosine A 1 receptors on the tubuloglomerular feedback. The heterodimerization of the D 1 R and adenosine A 1 receptor and D 2 -like and adenosine A 2A receptors inhibit dopamine receptor function (see adenosine and dopamine receptor interaction). Adenosine A 2A receptors counteract the effect of adenosine A 1 receptors on tubuloglomerular feedback and dopamine (probably via D 2 -like receptors) counters the effects of adenosine A 2A receptors. Superoxide anion activates 5′-nucleotidase, thereby increasing adenosine generation in the kidney. Dopamine also induces ecto-5′nucleotidase in glomeruli and because ecto-5′-nucleotidase catalyzes the formation of adenosine, dopamine should enhance tubuloglomerular feedback. However, the ability of dopamine receptors to inhibit ROS production may also play a role, because ROS is also involved in tubuloglomerular feedback. These counteracting effects of dopamine on adenosine formation, adenosine receptor action, NKCC2, and ROS may explain the variable effect of dopamine on tubuloglomerular feedback.
Renin, Angiotensinogen, and Aldosterone Secretion
Dopamine can increase, decrease or have no effect on renin secretion, in vivo . Dopamine can alter renin secretion directly or indirectly by effects on blood pressure and renal tubular ion transport. As indicated above, rat and mouse juxtaglomerular cells express the D 1 R ( Table 19.1 ). The D 1 R stimulates secretion of renin in rat primary cultured cells. In pithed rats and anesthetized dogs, dopamine and D 1 -like receptor agonists increase renin release. However, in non-pithed rats, dopamine or a D 1 -like receptor agonist inhibits renin secretion on a low or normal salt diet, by inhibiting cyclooxygenase 2 expression. However, when cyclooxygenase 2 expression is already inhibited, as is the case with salt-loading, D 1 -like receptor stimulation increases renin secretion. In humans on low or high sodium (300 mmol/day) intake D 1 -like receptor stimulation does not affect renin secretion, in agreement with the absence of D 1 R in human juxtaglomerular cells. D 1 -like receptor stimulation also does not affect plasma renin activity in subjects with mild essential hypertension on an unmonitored sodium intake, but increases it in hypertensive humans on a high (150 mmol Na) or normal sodium intake. The role of D 5 R on renin secretion in the rat remains to be determined; D 5 R is expressed in rat but not in mouse juxtaglomerular cells and deletion of Drd5 does not affect plasma or renal renin levels.
In salt replete humans, D 2 -like receptor blockade with metoclopramide does not affect plasma renin activity but another D 2 -like receptor antagonist, domperidone, inhibits the fall in plasma renin activity induced by intravenous γ-L-glutamyl-L-dopa in humans on an unmonitored salt intake. Disruption of the Drd3 in mice leads to increased renin release and renin-dependent hypertension. In contrast, deletion of Drd4 does not affect plasma or renal renin levels. D 2 R also probably do not play a role in renin secretion, because they are not expressed in the juxtaglomerular cell in rat, mouse or human kidney (unpublished observations). Whether or not the reported effects of dopamine and D 1 -like and D 2 -like receptors on renin secretion are exerted at the macula densa remains to be confirmed, but all the dopamine receptor subtypes have been found in macula densa cells, albeit with species variability ( Table 19.1 ). These studies would suggest that in humans, the predominant effect of dopamine receptors on renin secretion is inhibitory, via D 2 -like receptors. A stimulatory effect could occur in hypertensive humans (see above), similar to that suggested in Sprague-Dawley rats , which are salt-sensitive.
D 1 R is expressed in the zona glomerulosa of rat adrenal glands and increases aldosterone secretion in isolated and cultured rat adrenal glomerulosa cells. In humans, dopamine does not affect aldosterone secretion in the salt-replete state but dopamine inhibits angiotensin II-induced aldosterone secretion. This would be in keeping with the attenuation of angiotensin II-induced aldosterone secretion by bromocriptine (D 2 R/D 3 R agonist) in human adrenal cortical and adenocarcinoma cells. These observations are confounded by the report that stimulation of D 4 R enhances angiotensin II-stimulated aldosterone secretion in the same human adrenal cortical and adenocarcinoma cells. However, in hypertensive subjects, the D 2 -like receptor agonist bromocriptine (D 2 R/D 3 R agonist) decreased renin and aldosterone secretion which was reversed by metoclopramide, a non-selective D 2 -like receptor antagonist. Metoclopramide did not affect aldosterone levels in saline-loaded normal and heart failure subjects. Because saline loading normally decreases aldosterone levels, this study suggests that dopamine does not normally stimulate aldosterone secretion. However, the inhibitory effect of D 2 R on aldosterone secretion is consistent with the increase in urinary aldosterone in D 2 −/− mice. In contrast, urinary aldosterone is not different between D 4 −/− and D 4 +/+ mice, D 3 −/− and D 3 +/+ mice (unpublished studies) or D 5 −/− and D 5 +/+ mice. Thus, dopamine probably normally is inhibitory of aldosterone secretion, mainly via the D 2 R.
Autocrine/Paracrine Regulation of Renal Function by Dopamine
The autocrine/paracrine regulation of renal tubular sodium transport, via D 1 -like receptors, is mediated by tubular and not by hemodynamic mechanisms (also see below). Systemically administered dopaminergic drugs may not mimic the autocrine/paracrine function of dopamine. The quantitative contribution of each dopamine receptor subtype on renal sodium transport remains to be determined. However, the D 1 R is responsible for ≈80% of D 1 -like receptor activity in renal proximal tubule cells ; D 5 R may be more important in the distal nephron, while the D 3 R may regulate glomerular dynamics. Each of the dopamine receptor subtypes, alone or via interaction with the other dopamine receptor subtypes or other GPCRs, regulates sodium transport in a unique fashion. Indeed, disruption of any of the dopamine receptor genes results in hypertension, the pathogenesis of which is specific for each subtype.
Regulation of Ion and Water Transport
Ion Transport
Euvolemia and Moderate Volume Expansion
Dopamine and its receptors are important in the regulation of ion transport during euvolemia or moderate volume expansion, but have a minor role under marked volume expansion. Inhibition of renal dopamine production by pharmacological inhibition of DOPA decarboxylase activity or silencing of Ddc in mouse proximal tubule impairs the ability to excrete a sodium load. In contrast, inhibition of the breakdown of renal tubular dopamine by inhibition of COMT but not MAO activity increases sodium excretion. Deletion of Comt in mice, however, decreases the natriuretic response to an acute sodium load, apparently because in these mice, basal renal dopamine production is increased and is not futher increased by saline loading. The increase in ion excretion caused by dopamine or D 1 -like receptor agonists cannot be entirely ascribed to its ability to increase renal blood flow or glomerular filtration rate. Reduction in renal blood flow to control values decreases but does not abolish the natriuretic effect of the D 1 -like receptor agonist fenoldopam administered into the renal artery. Rather, dopamine can directly inhibit renal tubular ion transport by inhibition of ion transporters, sodium channels, and sodium pump activity. The short-term inhibition of ion transport by dopamine involves alteration in enzyme kinetics and internalization of ion transporters/pump. The long-term inhibition of sodium transport by dopamine may involve regulation of protein expression by decreasing gene transcription and translation, and increasing their degradation.
The inhibitory effect of dopamine on ion transport is not simply a direct effect, but is modulated by its regulation of the release or secretion of other hormones/humoral substances. Hormones which directly inhibit ion transport interact with dopamine to increase (e.g., angiotensin III/AT 2 R, ANP/ANPA, eicosanoids, nitric oxide, prolactin, and urodilatin ) their inhibition of ion and water transport. In addition, dopamine negatively interacts with hormones that increase ion transport (e.g., angiotensin II, insulin, renin, angiotensin II/AT 1 R, and aldosterone ) and water transport (e.g., vasopressin ). The natriuretic effect of D 1 -like receptor stimulation persists even after renal denervation.
The inhibitory effects of dopamine, via D 1 -like receptors, on renal ion transport in vivo is well-established ( vide infra ). In contrast, the effect of D 2 -like receptor agonists on ion transport in vivo has not been consistent; reports have found no effect, a decrease or an increase in sodium excretion. The effect of blockade of D 2 -like receptors may not become evident unless the nitric oxide mechanism is impaired. The D 2 R and D 2 -like receptors have been reported to stimulate Na + K + -ATPase. The D 2 R/D 3 R agonist, bromocriptine, transiently increases basolateral chloride transport in the isolated mTAL. However, D 2 -like receptor stimulation inhibits the stimulatory effect of angiotensin II on Na + K + -ATPase.
Stimulation of D 3 R also inhibits NHE3 activity. The apparent discrepancies in these studies may be related, in part, to the status of the extracellular fluid volume. For example, a two-day administration of quinpirole, a D 2 R/D 3 R agonist, increased while a D 2 R antagonist decreased sodium excretion in D 2 +/+ mice fed high but not normal sodium diet. Because D 1 -like receptor effect is increased with moderate sodium loading, this would indicate the need of D 1 -like receptors for D 2 -like receptors to inhibit Na + transport. A selective D 3 R agonist, 7-OH-DPAT, increases sodium excretion in rats on a normal and high salt diet. The natriuresis in rats with streptozotocin-induced diabetes is decreased by D 3 R antagonist. However, the natriuretic effect of another D 3 R agonist, pramipexole, is blocked by a D 1 -like receptor antagonist. The natriuretic effect of dopamine has also been shown to depend on the activation of both D 1 -like and D 2 -like receptors. Therefore, the inhibitory effect of D 2 -like receptors on sodium transport may not be observed, unless the animals are salt-loaded, a state that enhances the natriuretic effect of D 1 -like receptors.
In hypovolemic or euvolemic states where vasopressin levels are elevated, the ability of D 2 -like receptor agonists, probably acting via the D 4 R, to inhibit the effects of vasopressin may obscure any stimulatory effect of D 2 -like receptors, probably via the D 2 R, on water and ion transport in more proximal parts of the nephron. However, preliminary studies suggest that D 4 R stimulation inhibits NCC activity in mouse renal distal convoluted tubule cells.
Dopamine inhibits ion and water transport in the proximal and distal nephron, as estimated from lithium clearance studies. It must be noted that the renal tubular site of action of endogenous dopamine and its agonists on ion transport may not always match that given exogenously. Most of the renal dopamine is produced in the proximal tubule, and so its effects in the distal nephron could be limited while exogenously administered dopamine would reach all nephron segments equally.
Luminal Membrane
Proximal Tubule
As stated earlier, all the dopamine receptor subtypes are expressed in the proximal tubule. The stimulation of D 1 -like receptors decreases the activity of several ion transporters, including the sodium hydrogen exchanger 3 (NHE3, SLC9A3), sodium phosphate co-transporter (NaPi-IIa/SLC34A1 and NaPi-IIc/SLC34A3) and Cl − /HCO 3 − exchanger (SLC26A6) at the luminal membrane. G-protein-dependent, cAMP/PKA-, PKC-, NHERF-1-, and phosphatase-dependent and -independent mechanisms are involved in the dopamine or D 1 -like receptor inhibition of NHE3, Na + /Pi2, Na + /HCO 3, and Cl − /HCO 3 − exchanger activity, including their translocation out of the brush border membranes into the cytosol. The signaling pathways by which D 1 -like receptors inhibit NHE3 activity may be species-specific, involving PKA only in rat and human renal proximal tubule cells ( Figure 19.2 ), but involving both PKA and PKC in renal opossum kidney cells. Specific PDZ domains of Pals-associated tight junction protein (PDZ 2, 4 or 5) or NHERF-1 (PDZ 2) may be important in organizing the signaling pathways by which dopamine inhibits sodium transporter/pump activity. In contrast, NHERF3/4 regulates the internalization of NaPiIIa.

The D 3 R and D 4 R may also regulate NHE3 ( Table 19.2 ) because its expression is increased in D 3 −/− and D 4 −/− mice on normal salt intake. However, the inhibitory effect of the D 3 R on NHE3 activity in renal proximal tubules is not linked to Gαs because pretreatment of the cells with cholera toxin did not prevent the D 3 R effect. Rather, the D 3 R-mediated inhibition of NHE3 activity in renal proximal tubules is related to Gα i3 , stimulation of PKC, and modulation of intracellular calcium. The effect of the D 3 R, in the rat, is probably independent of changes in intracellular cAMP because renal proximal tubules do not express adenylyl cyclase V. In mesenteric arteries, D 3 R causes vasodilation that is mediated by inhibition of Ca 2+ channels and modulation of K + channels, in agreement with the suggestion that the linkage of the D 3 R to other effectors, such as inhibition of K + and Ca 2+ may be more sensitive than the weak linkage to G-proteins.
Variable | D 1 -/- | D 2 -/- | D 3 -/- | D 4 -/- | D 5 -/- |
---|---|---|---|---|---|
Blood Pressure | High | High | High , Normal 560* | High | High |
Salt Sensitivity (chronic NaCl load) | Yes# | BP increased only with sodium load | Yes # , No 560* | Yes # | Yes |
Increased Na + transporter/exchanger, channel | ND | NHE3, NCC # | NHE3, NCC # | NHE3, NKCC2, NCC # | NKCC2, NCC, α & β ENaC subunits |
Na + K + ATPase, a subunit | ND | Low activity and protein # | Normal # | High # | Normal |
GPCR (kidney) | ND | Normal AT 1 R # , normal response to ARB, increased ETBR | High AT 1 R , | High AT 1 R | High AT 1 R a,46, 360 |
Renin-Angiotensin | ND | ND | High | Normal | Normal |
Aldosterone | ND | High | Normal # | Normal # | Normal |
Reactive Oxygen Species | ND | High | Normal # | Normal # | High a, 234 |
Inflammation | ND | High # | Normal | Normal # | ND |
Body Weight | Low | Normal , Low b | Normal , Fat load increases body weight in males; increased body fat in males and females c | Normal | Normal |
a Asico L, Zhang X, Jiang J, et al. Lack of renal dopamine D5 receptors promotes hypertension. J Am Soc Nephrol. 20;22(1):82–89.
b Kim KS, Yoon YR, Lee HJ, et al. Enhanced hypothalamic leptin signaling in mice lacking dopamine D2 receptors. J Biol Chem. 2010;285(12):8905–8917.
c McQuade JA, Benoit SC, Xu M, Woods SC, Seeley RJ. High-fat diet induced adiposity in mice with targeted disruption of the dopamine-3 receptor gene. 2004;151(1–2):313–319.
Loop of Henle
The mTAL expresses the D 1 R, D 3 R (in the mouse but not in the rat), D 4 R, and D 5 R. D 1 -like and D 2 -like receptor agonists administered to the apical membrane of the rat mTAL do not affect chloride transport. However, their application to the basolateral membrane decreases chloride transport via D 1 -like receptors that is unrelated to PKA or PKC, but rather to PLA2-mediated formation of 20-HETE. Incubation of mTAL suspensions with dopamine increases sodium potassium 2 chloride co-transporter (NKCC2, SLC12A1) activity. Indeed, NKCC2 activity is stimulated by PKA and PKC, but inhibited by 20-HETE. The PKA-mediated stimulation of Na + /K + -ATPase activity is observed only under oxygenated conditions, but is overridden by PLA2 inhibitory pathways under hypoxic conditions. Therefore, overall ion transport is inhibited, probably because of inhibition of basolateral chloride transport and Na + /K + -ATPase activity. The D 1 -like receptor-mediated stimulation of NKCC2 may be important in K + recycling in the mTAL. Bromocriptine (D 2 R/D 3 R agonist) increases chloride transport, albeit transiently, reminiscent of the ability of this agonist to increase Na + /K + -ATPase activity in renal proximal tubules. However, the D 4 R and D 5 R may negatively regulate NKCC2 expression because its expression is increased in D 4 −/− (unpublished) and D 5 −/− mice. NKCC2 expression is not altered in D 2 −/− and D 3 −/− mice and has not been reported in D 1 −/− mice ( Table 19.2 ).
Distal Convoluted Tubule
The distal convoluted tubule expresses all the dopamine receptor subtypes, but their effect on ion transport in this nephron segment has not been reported, except for D 4 R which inhibits NCC. However, the D 2 R, D 3 R, D 4 R, and D 5 R may regulate NCC because its expression is increased in D 2 −/− , D 3 −/− , D 4 −/− and D 5 −/− mice (unpublished data). NCC expression has not been reported in D 1 −/− mice ( Table 19.2 ).
Cortical Collecting Duct
D 1 -like receptor stimulation of the rabbit cortical collecting duct depolarizes the luminal membrane and increases cAMP production. D 5 R may regulate ENaC because α- and γ-subunit expressions are increased in D 5 −/− mice ; sodium transporters in D 1 −/− mice have not yet been quantified. Dopamine, via D 4 R, at the basolateral membrane decreases sodium transport in the rabbit cortical collecting duct. The D 4 R may also inhibit the increase in sodium transport mediated by vasopressin, via a decrease in cAMP. αENaC is increased in D 4 −/− mice but only following a high salt diet (unpublished observations). ENaC expression is not altered in D 2 −/− and D 3 −/− mice. ENaC expression has not been reported in D 1 −/− mice ( Table 19.2 ).
Medullary Collecting Duct
The D 1 R is expressed in the collecting duct or in the outer but probably not in the inner medulla, while the D 5 R is also expressed in the outer but not in the inner medullary collecting duct in the mouse kidney. Indeed, dopamine does not stimulate cAMP production in the rat inner medullary collecting duct. D 2 -like receptors are expressed in the medullary collecting duct; the D 2 R is expressed in the intercalated cells, while D 3 R and D 4 R are not differentially expressed between intercalated and principal cells. A D 2 -like receptor has been shown to stimulate PGE2 production in rat inner medullary collecting duct cells. The specific D 2 -like receptor involved is not known, but only the D 4 R is expressed in the inner medullary collecting duct; the expression of the D 2 R has not been consistently shown ( Table 19.2 ). It is not clear if dopamine regulates ion transport in the medullary collecting duct. Indeed, the inhibitory effect of dopamine on vasopressin-induced water permeability and cAMP accumulation in the rat inner medullary collecting duct is mediated by the α 2 adrenergic receptor.
Basolateral Membrane
D 1 -like receptors inhibit the electrogenic Na + /HCO 3 − co-transporter (NBCe1A, SLC4A4) expressed in the basolateral membrane of the proximal tubule and Na + /K + -ATPase expressed at the basolateral membrane in all nephron segments studied (proximal tubule, thick ascending limb of Henle, cortical collecting duct). Although stimulation of D 2 -like receptors may increase Na + /K + -ATPase activity, the inhibitory effect of dopamine on Na + /K + -ATPase activity may require simultaneous stimulation of D 1 -like and D 2 -like receptors, and could result in a potentially greater inhibition of the sodium pump caused by D 1 -like receptors. For example, in the rat proximal tubule (but not in the mTAL or cortical collecting duct) D 2 -like receptors act in conjunction with D 1 -like receptors to inhibit Na + /K + -ATPase activity and decrease sodium transport, similar to that proposed for NaPiII and NHE3. Under normal circumstances, dopamine receptors probably do not regulate the expression of the α-subunit of Na + /K + ATPase, because its expression is not altered in D 2 −/− , D 3 −/− , D 4 −/− , and D 5 −/− mice on a normal salt intake although this has not been studied in D 1 −/− mice.
The inhibitory effect of D 1 -like receptors on Na + /K + -ATPase is mediated by cAMP/PKA, certain PKC isoforms, and 20-HETE. The overall consequence of D 1 -like receptor stimulation is internalization of Na + / K + -ATPase subunits. The inhibitory effect of D 1 -like receptors on Na + /K + -ATPase involves PKC in the proximal convoluted tubule ( Figure 19.2 ) and PKA in the distal convoluted tubule and cortical collecting duct, while the eicosanoids are involved in all nephron segments, including the mTAL, PKC may also be involved in the inhibition of Na + /K + -ATPase in the mTAL. 20-HETE is important in the ability of dopamine to inhibit Na + /K + -ATPase activity in rat renal cortex, because it overrides the stimulatory effect of PKA. In the renal medulla, but not in the renal cortex, PKA and phosphatase inhibition also contribute to the inhibition of Na + ,K + -ATPase activity that may be related to DARPP-32 inhibition of PP-1, in the case of dopamine.
The effect of dopamine on Na + /K + -ATPase is tissue-specific. As stated above, dopamine inhibits Na + /K + -ATPase activity in renal cortical and medullary tubules. However, the regulation of the phosphorylation of Na + /K + -ATPase at Ser-23 is different between the proximal convoluted tubule and thick ascending limb of Henle; PKC phosphorylates Na + /K + -ATPase at Ser-23 in the proximal tubule but not in the mTAL. D 1 -like receptors may actually stimulate Na + /K + -ATPase activity in human ciliary nonpigmented epithelial and pulmonary alveolar cells. D 2L R also stimulates Na + /K + -ATPase in murine fibroblasts. D 1 R and D 2 R, on the one hand, and Na + /K + -ATPase, on the other, can also negatively regulate each other in HEK293T cell by direct protein–protein interaction. While the inhibition of Na + /K + -ATPase in the kidney by dopamine under conditions of sodium chloride excess is beneficial, inhibition of Na + /K + -ATPase activity in neuronal cells by high concentrations of dopamine can lead to cell death. Inhibition of Na + /K + -ATPase activity in vascular smooth muscle cells would increase vascular resistance, as has been reported in the rat tail. Low concentrations of dopamine, however, decrease systemic vascular resistance probably by mechanisms other than via regulation of sodium transporter or pump activity, e.g., opening of potassium channels ( vide supra , “Dopamine and Renal Hemodynamics”).
Water Transport
Dopamine may also regulate water transport. Dopamine inhibits arginine vasopressin-mediated increase in water transport, via the D 4 R in the rat cortical collecting duct. Dopamine has been reported also to inhibit vasopressin-stimulated increase in water permeability in inner medullary collecting duct cells. Although it has been claimed to be exerted at a 2 -adrenergic receptors, the effect could be inhibited by clozapine, a D 4 R antagonist. The dopamine-mediated reversal of vasopressin-mediated increase in water transport has been related to a decrease in expression, and increase in phosphorylation, of aquaporin 2 at the apical plasma membrane. In addition, dopamine, via D 4 R, increases the ubiquitin-mediated degradation of aquaporin 2 in lysosomes and decreases the aquaporin 2 transcription by decreasing vasopressin-induced increase in cAMP production. D 1 -like receptors probably do not play a role in the vasopressin-mediated transport, at least in the rat inner medullary collecting duct, because dopamine does not increase cAMP levels in this nephron segment. Aquaporin 4-mediated increase in basolateral permeability is also impaired by dopamine, via a yet to be determined receptor subtype that is linked to PKC. The D 2 R can regulate aquaporin 4 in glial cells, but is probably not the dopamine receptor involved in the medullary collecting duct because the D 2 R is not expressed in principal cells of this nephron segment. The diuresis associated with hypotonic saline loading has been related to the ability of D 1 -like receptor antagonist to decrease vasopressin release. However, dopamine is known to increase vasopressin secretion from the hypophysis. This discrepancy could be taken to indicate that the dopamine-mediated release of vasopressin may be influenced by the state of volume expansion.
Hypovolemia
In contrast to the natriuretic effect of endogenous renal dopamine in euvolemic and in moderately volume-expanded states, in sodium-depleted states the D 1 -like agonist, fenoldopam, does not affect sodium excretion, while dopamine actually decreases sodium excretion. A limited number of studies have assessed the dopamine receptor subtype that may increase renal sodium reabsorption in hypovolemic states. In conscious, chronically instrumented dogs on a sodium intake of 40 mmol/day, quinpirole (D 3 R and D 4 R agonist) decreased sodium excretion as a consequence of both a decrease in renal blood flow and an increase in tubular sodium reabsorption. Dopamine has also been reported to stimulate NHE3 and Na + /K + -ATPase activity in rabbit renal proximal tubule cells and NKCC2 in mTAL ; bromocriptine (D 2 R/D 3 R agonist) stimulates Na + /K + -ATPase activity in rat renal proximal tubule cells and increases chloride transport in the mTAL. Stimulation of the D 2S R or D 2L R heterologously expressed in murine LTK-cells increased Na + /K + -ATPase and NHE1 activity. These may be the mechanisms by which dopamine increases sodium transport during hypovolemia.
Dopamine Receptor Subtype Mutant Mice
The deletion of dopamine receptor subtype in mice has helped to determine the role of each dopamine receptor subtype in the regulation of renal function and blood pressure. Each dopamine receptor subtype participates in the regulation of blood pressure by mechanisms specific for the subtype. As described above, some receptors influence epithelial transport. Others, as described below, regulate blood pressure by influencing the central and/or peripheral nervous system and regulating the secretion and receptors of several humoral agents ( Table 19.2 ).
D 1 R Mutant Mice
D 1 −/− mice are growth retarded without obvious neurological defects, but exhibit a decrease in rearing behavior. The low survival after weaning is caused by decreased feeding ability. D 1 −/− mice and D 1 −/+ mice (C57BL/6 background) on a normal NaCl diet have higher systolic and diastolic blood pressures than D 1 R wild-type (D 1 +/+ ) mice. Homozygous D 1 −/− mice do not increase renal tubular cAMP accumulation in response to dopamine stimulation, but the response to parathyroid hormone is intact. These data indicate D 1 R specificity in the increased blood pressure in mice. However, it is not clear why the other D 1 -like receptor, D 5 R, is unable to compensate for the lack of D 1 R; D 5 R expression is not altered in D 1 −/− mice and D 1 R expression is not altered in D 5 −/− mice. The hypertension of D 1 −/− mice is aggravated by an increase in salt intake ( Table 19.2 ). The hypertension of D 1 −/− mice is aggravated by an increase in salt intake ( Table 19.2 ).
D 2 R Mutant Mice
D 2 −/− mice in C57BL/6J background have decreased motor activity but rotarod performance is not impaired. D 1 -like receptor binding may be decreased, while D 3 R is transiently increased after birth in D 2 −/− mice. D 2 −/− and D 2 −/+ mice (C57BL/6J) on a normal NaCl diet have higher systolic and diastolic blood pressures than D 2 wild-type (D 2 +/+ ) mice. α-Adrenergic blockade decreases blood pressure to a greater extent in D 2 −/− mice than in D 2 +/+ mice, but acute adrenalectomy decreases blood pressure to a similar level in D 2 −/− and D 2 +/+ mice. ETB receptor expression is greater in D 2 −/− mice than in D 2 +/+ mice, and ETB receptor blocker decreases blood pressure in D 2 −/− mice but not D 2 +/+ mice. D 2 −/− mice also have increased production of ROS; increasing antioxidant activity with hemin normalizes the increased blood pressure. These data indicate that D 2 −/− mice may have enhanced vascular reactivity caused by increased sympathetic and ETB receptor activities, and oxidative stress. The D 2 −/− mice also have increased production of aldosterone, and treatment with a mineralocorticoid receptor blocker normalizes blood pressure but not the increased oxidative stress in these mice, indicating that the increased mineralocorticoid activity is distal to the increased oxidative stress. In an unspecified strain of D 2 −/− mice, blood pressure is increased only when the mice are fed a high-salt diet; this is associated with a decrease in renal AADC activity and renal dopamine production. Sympathetic activity is not increased in these D 2 −/− mice. The differences between the two strains of D 2 −/− mice could be related to differences in the genetic background, similar to the differences in behavior of D 2 −/− mice from different genetic backgrounds.
D 3 R Mutant Mice
The locomotor phenotype of D 3 −/− mice does not resemble that of D 2 −/− mice. D 3 −/− mice may show a transient locomotor hyperactivity in a novel environment. The D 3 R may be involved in seeking behavior for natural reinforcers such as food in rodent models of obesity ; D 3 −/− mice fed a high fat diet become obese. D 3 −/− and D 3 −/+ mice (C57BL/6J) on a normal NaCl diet have both higher systolic and diastolic blood pressure than their wild-type (D 3 +/+ ) littermates. These D 3 −/− and D 3 −/+ mice have increased renal renin production, but sustained decrease in blood pressure with AT 1 R blockade is observed in the D 3 −/− but not D 3 −/+ or D 3 +/+ mice. An unspecified strain of D 3 −/− mice has normal blood pressure regardless of salt intake. Nevertheless, these two strains (C57BL/6 and an unspecified strain) of D 3 −/− mice have decreased sodium excretion after an acute or chronic sodium chloride load. Differences in phenotypes can occur depending on the genetic background, and even the same mouse strain from different commercial sources. For example, the blood pressure of C57BL/6 from Jackson Laboratories is salt-sensitive while the blood pressure of C57BL/6 mice from Taconic is salt-resistant. The salt-sensitive hypertensive phenotype of human G-protein-coupled receptor kinase type 4 (GRK4) 486V transgenic mice is dependent on the percentage of genetic background from salt-resistant SJL mice.
D 4 R Mutant Mice
D 4 R deficient (D 4 −/− ) mice have impaired photoreceptor response, reduced response to novelty, but attenuated locomotor response to amphetamine, but not to methylphenidate or cocaine. Congenic D 4 −/− but not D 4 −/+ ( Table 19.2 ) mice have increased systolic and diastolic blood pressures. The blood pressure is increased further with increased sodium intake in D 4 −/− mice ( Table 19.2 ) but the effect of sodium intake on blood pressure in D 4 −/+ has not been tested. D 4 −/− mice do not have altered circulating or renal renin or aldosterone levels. Renal and brain, but not cardiac, AT 1 R expression is increased in D 4 −/− mice. The hypotensive effect of a bolus intravenous injection of the angiotensin type 1 receptor blocker, losartan, persists longer in D 4 −/− than in D 4 +/+ mice. Thus, the hypertension brought about by the absence of the D 4 R is mediated, in part, by increased AT 1 R expression , similar seen in D 3 −/− and D 5 −/− ( vide infra ).
D 5 R Mutant Mice
D 5 −/− mice have no impairment in learning and memory. D 5 −/− mice have normal expression of the other dopamine receptors, including the D 1 R, a feature found in the other dopamine receptor subtype knockout mice except for the D 2 −/− mice. D 5 −/− and D 5 −/+ mice are hypertensive. As with the D 4 −/− mice ( Table 19.2 ), the blood pressure is increased further with increased sodium intake in D 5 −/− mice. Epinephrine/norepinephrine ratio and the hypotensive response to the acute administration of an α-adrenergic blocker are greater in D 5 −/− mice than their D 5 +/+ littermates, indicating that increased sympathetic activity plays a role in the elevated blood pressure observed with deletion of the Drd5 gene. Central nervous system pathways involving glutaminergic, oxytocin, vasopressin, and adrenergic receptors are important in the pathogenesis of hypertension in D 5 −/− mice. Besides these central nervous system mechanisms, renal AT 1 R and increased production of ROS are also involved in the hypertension of D 5 −/− mice.
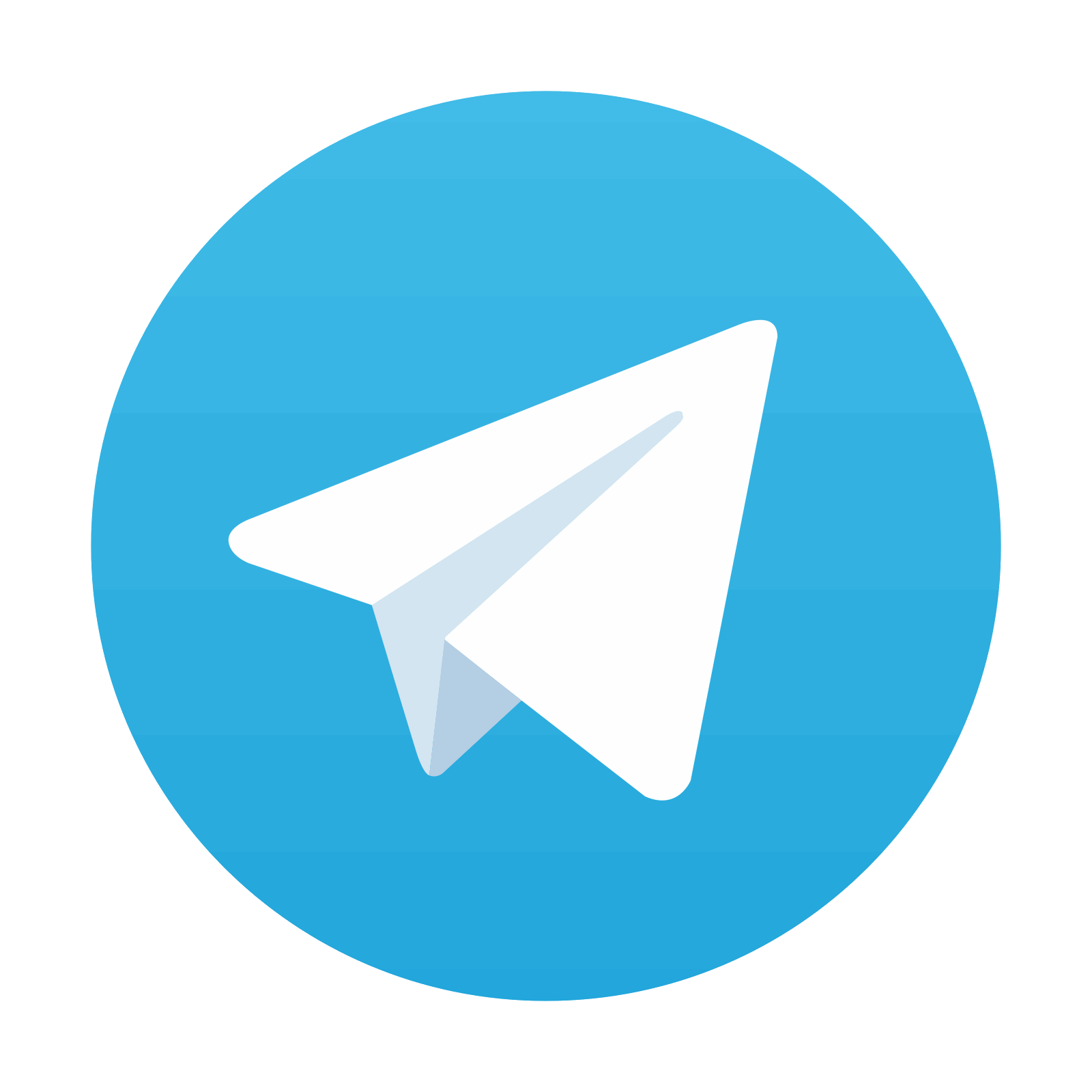
Stay updated, free articles. Join our Telegram channel

Full access? Get Clinical Tree
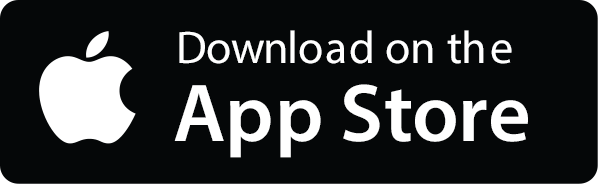
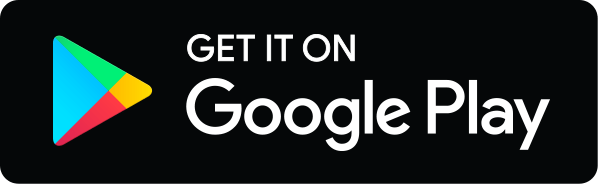