Radiopharmaceutical agent
Indication
99Tcm Diethylene Triamine-pentaacetic acid (DTPA)
Measurement of GFR, renography
99Tcm Mercaptoacetylgly-cylglycylglycine (MAG3)
Clearance studies, renography
99Tcm dimercaptosuccinic acid (DMSA)
Divided renal function, characterization of renal space occupying lesion
99Tcm methyl di-phosphonate (MDP)
Assessment of bone metastases, renography
99Tcm labelled red cells
Assessment of cardiac function (pre and post chemotherapy)
Measurement of Glomerular Filtration Rate
For in vitro testing of renal physiology, a long living isotope is preferable, attached to a product, which is excreted via a single part of the nephron. For this Chromium-51 (51Cr) is ideal when attached to ethylenediaminetetracetic acid (EDTA) which is filtered by the glomerulus and not re-absorbed. The gamma irradiation is not good for imaging but in small quantities such as 3–4 MBq will be measured efficiently in a well counter and therefore can be used via the radio-activity in sequential blood specimens to calculate the glomerular filtration rate (GFR). This is used in assessment of renal function during chemotherapy but in combination with 99Tcm DMSA can be used to predict residual renal function after nephrectomy.
Positron Emitters
The second main class of imaging radiopharmaceuticals are those that are positron emitters. Positrons are positively charged electrons emitted during decay of proton rich nuclei. To obtain these isotopes means adding protons or proton rich nuclei to the nucleus of the target atom. This cannot be done in a reactor instead a machine called a cyclotron accelerates the These positively charged particles, such as protons or alpha particles to close to the speed of light and then bombards them into a precise target. The resulting positron emitting isotopes tend to be of low atomic number such as oxygen nitrogen or fluorine. These have short half-lives so have to be used close to the site of production. For example, the most commonly used of these positron emitters is fluorine-18 (18F). This has a 2-h half-life and as after production some time will be taken up combining the positron emitter with a physiologically useful organic molecule such as glucose to make FDG. It is generally considered practical if the images are performed within a 2-h radius of production. This might mean 30 miles by road transport in a big city but 600 miles when the product can be moved by air.
Imaging with Positrons
All positron emitters produce two 511 kEV gamma rays after their annihilation with an electron. The two gamma rays travel at 180° and can be identified by a paired detection system. Though traditional gamma cameras can be used the 511 jeV energy is a little high for them to work efficiently so a special crystal such as Beryllium-Germanium-Oxide (BGO) is used. These are set in a ring to collect the pairs of 511 keV photons, called co-incidence events. This enabled a 3 dimensional map of positron emission to be produced. This type of machine is a positron emission tomography (PET) camera. The most common form available at present is a combination of a PET machine and CT. The CT component allows accurate localisation of any site of abnormal uptake and is also used to correct the PET image for any attenuation of the gamma signal through the patient. In the last 12 months PET combined with MRI has also been launched. This technique must be considered experimental and there has been no evidence that it will be of use in urological cancers.
Therapy Isotopes
The third type of radiopharmaceutical has a particulate form of emission such as a beta or alpha particle. These have mass and therefore can deliver a higher radiation dose to any tissue they interact with but cannot be used for imaging unless there is an accompanying gamma emission. In urological cancers there use until recently has been limited to pain relief from bone metastases, however new agents which directly target renal cancer are entering phase II and phase III clinical trials.
Imaging Devices
To be able to image radiopharmaceuticals efficiently, a range of imaging devices have been produced. They all relay on the same principle. They use a scintillator, which is a type of crystal, which is temporarily energised by being hit by a gamma ray resulting in the production of visible light. This is picked up by a photo-multiplier tube (PMT), which converts this signal into the electrical energy, which is amplified and then passed on to a computer. The simplest form of such a system is found in a well gamma counter which can be used to measure small amounts of radioactivity in blood.
The Anger Camera
Invented in 1959 by Hal Anger, the gamma camera uses a large single scintillation crystal often 40 × 60 cm and about 2 cm thick. To pick up the light signal as array of 30–70 PMTs are needed and are normally hexagonal so they fit together in a honeycomb fashion to ensure the surface is covered. As the amount of light produced by the scintillation crystal is small both the scintillation crystal and PMTs are held in a light proof box. Gamma rays cannot be focused like light so any scattered photos would degrade the image and make it look more “fuzzy”. To remove these scattered photons the gamma rays pass through a series of parallel lead lined tubes called a collimator. The width of the septa in the collimator is determined by the energy of the gamma rays and divided into “low energy” (0–180 keV), medium energy (180–300 keV) and high energy (>300 keV). The computer that is attached to the gamma camera system then determines where on the crystal a gamma ray arrived and its energy. These camera cameras can be mounted on gantries that can move along the patient to perform whole body images and rotate around the patient producing a three-dimensional image called single photon emission computed tomography (SPECT). The associated computer system can detect a series of images (every 0.5 s upwards) of a particular part of the body. This is called a dynamic image then the computer can calculate the activity of a radiopharmaceutical at any area of interest to perform a time activity curve as seen in renography.
Positron Emission Tomography (PET) Imaging
PET imaging does not need collimation, as a scattered gamma ray will be deflected much like a billiard ball hitting one another. Therefore it will not arrive 180° from its partner gamma ray released at the same time and will not make a co-incidence event and will not be recorded. As no lead collimation is needed in PET less gamma rays are stopped and the system is more efficient than a standard gamma camera.
Tomography
Both SPECT and PET are excellent methods to look at functional images of the body and are finding increasing roles in the imaging of urological cancers. However spatial resolution is limited to about 7 mm for SPECT and 3 mm for PET, though these figures are optimistic and describe the best attainable. Also identifying the site of any abnormal uptake can be difficult due to the lack of anatomical markers. Therefore both SPECT and PET machines have been added to CT scanners to allow for simultaneous SPECT/CT and PET/CT a technique called image registration.
Radiation Dosimetry
The radiation burden for nuclear medicine tests tends to lie in the low to medium band compared to other radiological studies The unit of measurement for radiation is the Sievert (Sv) which equals a joule of received energy per kg of tissue Clearly as this is a lot of energy normally a mSv is used.
Residents of London receive on average 2–5 mSv of radiation per year. Residents of Cornwall or Aberdeen who live on granite may receive an annual radiation dose of 5–10 mSv due to Radon gas seeping into their home. The area of the world with the highest annual background radiation dose is the Southern Caucasus with an annual radiation rate of 25 mSv. The radiation dose from X-rays studies may vary depending on the number of views taken, energy of the X-ray beam and exposure time. In Nuclear Medicine the radiation dose is determined by the activity of radioisotope given. In the UK this is itself governed by a statuary instrument and administered through a committee of the Department of Health called the Administration of Radioactive Substances Advisory Committee (ARSAC) who publish typical maximum activities and radiation doses received (Table 6.2) [1]. A few radiological investigations are included for comparison. However, it can be seen that the excess radiation of a single nuclear medicine test is not great compared to background radiation.
Table 6.2
Radiation dose for investigations commonly used in urological cancer
Investigation | Radiation dose measured as effective dose equivalent (mSv) |
---|---|
3 MBq 51Cr-EDTA for GFR | 0.006 |
100 MBq 99Tcm MAG3 renogram | 0.7 |
400 MBq 18F FDG PET scan | 10 |
KUB plane X-ray | 1–3 |
Spiral CT of kidneys with contrast | 3–24 |
IVU-6 image | 1–6 |
Assessment of Renal Function
The most commonly used isotopic test for the assessment of renal function is the measurement of GFR with a radiopharmaceutical, which is exclusively filtered in the glomerulus. There are several agents which can do this including 99Tcm-DTPA. However the short half-life of 99Tcm at 6 h means that any counts must be decay corrected and correct timing of samples taken is vital. Also in patients with impaired GFR (that is below 30 ml/min), a 24-h sample is needed, which is not practical with 99Tcm-labeled pharmaceuticals. The method of choice then is to use 51Cr-EDTA, which has a half-life of 27 days. This helps make counting simple with no real need to decay correct and samples may be counted 2–3 days after the test has been performed. In addition taking a late sample at 24 h is simple.
The standard method is to take a background sample and then three samples 2, 3 and 4 h after injection of 2–4 MBq of 51Cr-EDTA. The activity in a millilitre of plasma is then plotted on a graph and the rate of reduction of counts gives the GFR. However, a simpler 1 blood sample method and some mathematical modelling by Martenssen et al. [2] has shown that if the GFR is more than 50 ml/min then it is just as accurate as a three sample method. Likewise if the GFR is less than 30 ml/min, a 24 sample should be added. As it may not be possible to pre-predict the exact GFR before a test is done then more than one method may be needed. The creatinine clearance or estimated GFR can be used as a guide but may be inaccurate if the patient is in a catabolic state from malnutrition (the anorexia of cancer or post-chemotherapy), or has impaired renal function or has suffered a recent muscle injury including an operation. Also the GFR is more accurate for sequential measurements especially if potentially nephrotoxic treatment is being given [3, 4].
The main use of a GFR in urological cancer management is the correct dosing of nephrotoxic chemotherapy (especially platinum based drugs) before and during treatment. The combination of the GFR and divided renal function as determined by a 99Tcm DMSA study can be used to predict post nephrectomy renal function. The EPRF may be a better measure of early drug induced renal toxicity than GFR but it has not been accepted into standard clinical practice.
Cardiac Assessment
Though not directly connected to the urological system, a well functioning heart it is required for the efficient treatment of some patients. Many nephrologists think that the heart is there merely to perfuse the kidneys and there is some element of truth in this. However, there are two specific areas in which assessment of the heart using radionuclide techniques may be of particular value. The first is a general assessment of myocardial perfusion before any major surgery such as nephrectomy as not only is cancer more common in the elderly but so is heart disease. Therefore the high negative predictive value of stress and rest myocardial perfusion scintigraphy using 99Tcm-methyliso-butyliso nitrile (MIBI), 99Tcm-tetrofosmin or thallous-201 chloride (201TlCl) will determine, if normal with no evidence for myocardial ischaemia that a major operation can proceed without risk to the patient.
In addition gated blood pool imaging using 99Tcm-labeled red blood cells allow an accurate determination of left ventricular ejection fraction (LVEF) which is less dependent of left ventricular geometry than stress echocardiography and may be more accurate in serial studies [5]. This is used to assess the LVEF before and after chemotherapy with cardiotoxic drugs of doxorubicin type and may be of particular use if the patient has co-existent hypertension and or diabetes for which the commonly used nomograms for maximal tolerated drug may not apply.
Renography
Renography, acquired by dynamic gamma camera imaging normally with 300 MBq 99Tcm-DTPA or preferably with 100 MBq 99Tcm-MAG3 again are not primary methods used to assess urological cancers. However, they have two main roles. Firstly, in combination with a GFR it may be used to determine the expected result of a nephrectomy on residual renal function. For example, if a renogram demonstrates that each kidney contributes 50 % of renal function and the total GFR is 80 ml/min, removal of one kidney will result in a residual GFR of 40 ml/min. Interestingly as the most common agent used for bone imaging 99Tcm-MDP is up to 80 % excreted in the kidneys during the first 20 min post injection it may be possible to combine a 99Tcm-MDP renogram with a staging bone scan, which if combined with a GFR may provide all the staging required for the patient, in nuclear medicine terms, quickly and efficiently with reduced cost and radiation dose to the patient.
The second use of renography is to assess renal function if there is pelvic tumor, which is suspected of blocking one or both ureters, in which case a high urine flow rate needs to be achieved to prevent false positive studies due to ureteric dilatation form old obstruction. Frusemide can be given at any time during the renogram but if ureteric obstruction is suspected giving the Frusemide 15 min before the imaging agent (the Manchester protocol or F-15 protocol) means that the maximum diuresis occurs when the imaging agent in injected [6]. A flat or rising excretion phase curve suggests partial or complete obstruction of the ureter on that side (Fig. 6.1). If the ureter is stented or a nephrostomy placed the F-15 renogram should be repeated about 48 h later to determine if the kidney is now clearing. The divided function on the affected side will give some idea of the age of the obstruction, but if it is within a few days, the function of the affected kidney would not have been reduced by a huge margin and relief of the obstruction should result is a rapid return to full function of that kidney. If the function of the obstructed kidney is poor then a full recovery becomes less likely.
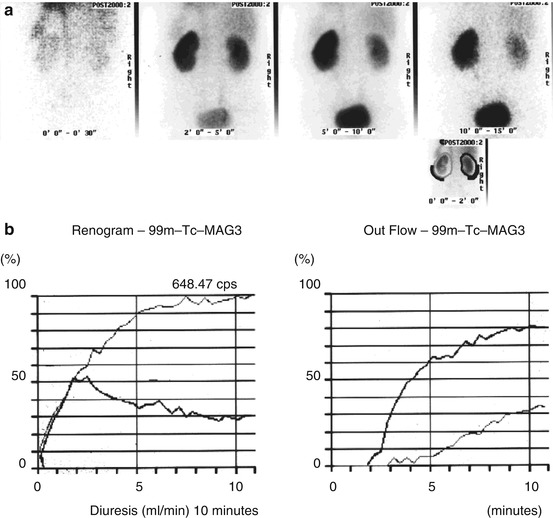
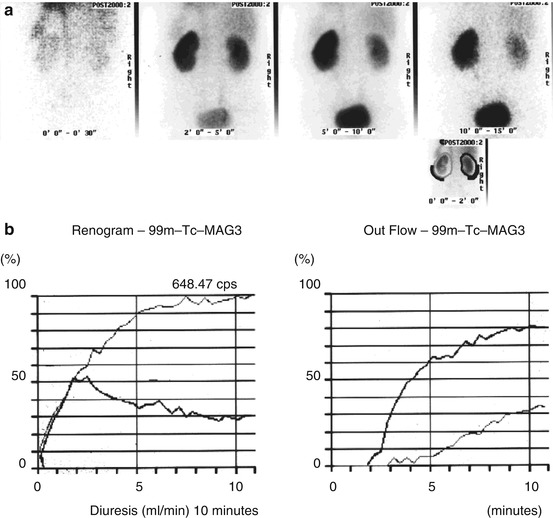
Fig. 6.1
(a) A series of static images of two kidneys imaged after injection of Tc-99m MAG3. The right kidney clears but the left kidney retains activity. (b) The renogram curves shows the left kidney (unbold line) confirms a rising curve and low output confirming obstruction on the left
Ureteric Obstruction
In very acute obstruction, which is complete, the renogram with 99Tcm-MAG3 demonstrates not hold up in the renal pelvis but in the kidney parenchyma-a sort of “shock kidney” picture. The clues are divided function is normal, the other kidney drains and ultrasound suggests a dilated collecting system. If this pattern is seen early drainage procedures work well, often without any residual loss of function on the affected side.
< div class='tao-gold-member'>
Only gold members can continue reading. Log In or Register a > to continue
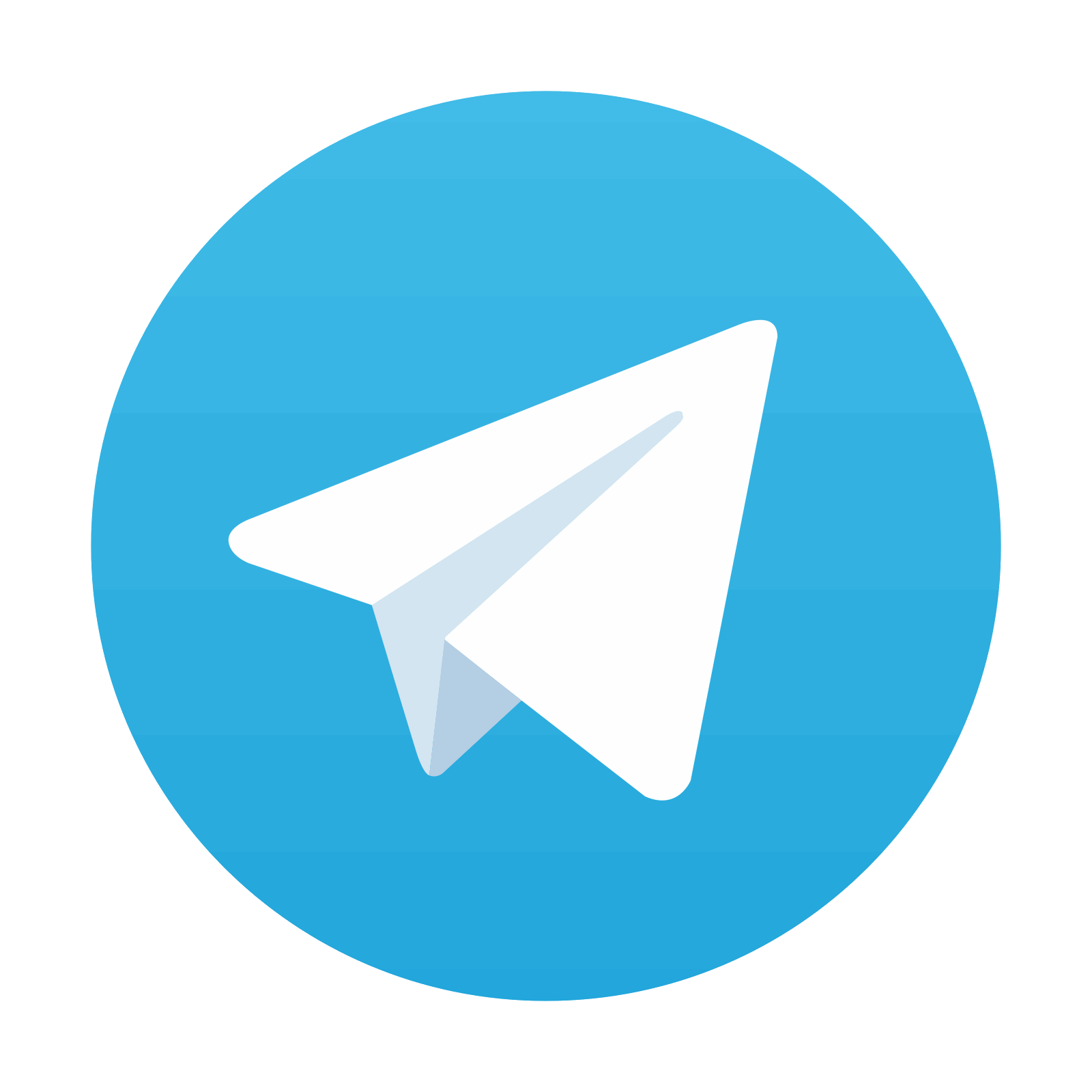
Stay updated, free articles. Join our Telegram channel

Full access? Get Clinical Tree
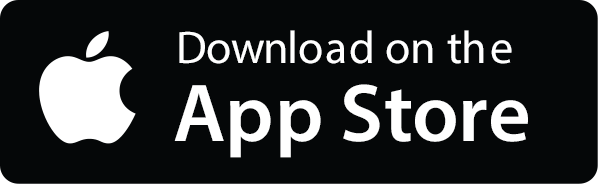
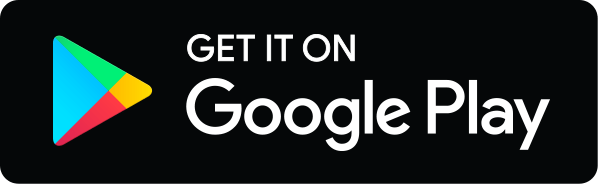