Chapter 6 Normal Fertilization and Implantation
HISTORICAL PROSPECTIVE
Before the initiation of modern reproductive and developmental biology in the 17th century, the theory of “seeds” belonging to the pluralistic current of the Pythagorean School led by Anaxagoras of Clazomenae and Empedocles of Akragas was the most believed (5th century B.C.).1 In the field of human reproduction, pluralism means that a fetus results from the mixing of two parental seeds. Hippocrates (c. 460–370 B.C.) believed that “seeds” are produced in all parts of the body, each containing both the masculine and feminine principle; when transmitted to offspring at the time of conception, they cause certain parts of the offspring to resemble their parents. A century later, Aristotle (384–322 B.C.) rejected Hippocrates’ theory. For him, only the male’s seed contributes to form the fetus; the female’s role in reproduction is to contribute menstrual blood. He had noticed that offspring sometimes resemble their grandparents rather than their parents. It was difficult to understand how seed of tissue and blood could be maintained unnoticed in the parents only to be revealed again in the children. Aristotle proposed that male semen was a mix of ingredients that was not blended perfectly so that materials from previous generations could sometimes get through. Most of Aristotle’s ideas were presented in his treatise, On Generation of Animals. This was the very first complete embryologic work ever written. Moreover, he was the first to illustrate his treatises, which significantly helped to clarify his writings.
Galen (130–201 A.D.), who is considered the greatest Greek physician after Hippocrates and founder of experimental physiology, supported Hippocrates’ theory that the seeds of both man and woman contribute to reproduction, but that each contains only one principle.1 In the 17th century, several outstanding findings fueled new scientific developments in modern reproductive biology. William Harvey (1578–1657) was the first to suggest that humans and other mammals reproduced via the fertilization of an oocyte by sperm. However, many authors see Regnier de Graaf (1641–1673) as the founder of modern reproductive biology.2 De Graaf is the one who discovered (1672) the source of oocytes in the testes of women, which we now call ovaries. Five years later the initial discovery of spermatozoa was made by a medical student named John Ham, who told Anton van Leeuwenhoek of the presence of what was termed animalcules in human seminal fluid, thought to have arisen from putrefaction. Leeuwenhoek (1632–1723) was the first to make detailed and accurate identification of sperm as a normal constituent of semen.3 Van Leeuwenhoek proposed that fertilization occurs when the sperm enters the oocyte, but this could not actually be observed for another 100 years because of the quality of microscopes available.
Another discovery that revolutionized scientific thinking was by the Italian priest and physiologist Lazaro Spallanzani in 1779. Until that time, our understanding of reproduction was based on our knowledge of how plants grow. It was believed that the embryo was the “product of male seed, nurtured in the soil of the female.” Spallanzani’s experiment established for the first time that for an embryo to develop there must be actual physical contact between the oocyte and the sperm. Spallanzani successfully inseminated frogs, fish, and dogs. The first successful artificial insemination of a woman was recorded just 11 years after Spallanzani’s experiment. In 1790, the renowned Scottish anatomist and surgeon, Dr. John Hunter, reported successful insemination of the wife of a linen draper using her husband’s sperm. These discoveries led to the emergence of modern reproductive technologies, by which the world’s first IVF baby was born just before midnight on 25 July 1978 as a result of continued efforts of Drs. Edwards and Steptoe.
SPERM TRANSPORT IN THE MALE REPRODUCTIVE TRACT
Spermatogenesis refers to the complex process of transformation of germline stem cells into sperm cells within the seminiferous tubules of the testes. Spermatogenesis is regulated through endocrine interactions between the pituitary gland and Sertoli cells. This endocrine system is referred to as the hypothalamic-pituitary-gonadal axis and involves a series of signaling mechanisms. Two hormones, follicle-stimulating hormone (FSH) secreted by the pituitary, and androgens (i.e., testosterone) produced by the Leydig (interstitial) cells in the testis, control Sertoli cell functions. Once formed within the seminiferous tubules, the immotile spermatozoa are released into the luminal fluid and transported to the epididymis, where they gain the ability to move and fertilize the oocyte.4 The testicular spermatozoa are transported passively to the rete testis, which is a branched reservoir of the openings of the seminiferous tubules. From the rete testis, the spermatozoa are transported to the epididymis via the efferent ductules.5 In mammals, the transit of spermatozoa through the epididymis usually takes 10 to 13 days; in humans the estimated transit time is 2 to 6 days.6 The epididymal segment where most spermatozoa attain their full fertilizing capacity appears to be the proximal cauda. The spermatozoa from that region are capable of moving progressively, which is characteristic of spermatozoa preceding fertilization, and bind to zona-free hamster ova in vitro at a higher percentage than spermatozoa obtained from more proximal locations.7
To attain the capacity to fertilize an oocyte, sperm undergo many maturational changes during its transit in the epididymal duct.4 These include, for instance, changes in plasma membrane lipids, proteins, and glycosylation; alterations in the outer acrosomal membrane; gross morphologic changes in acrosome in some species; and cross-linking of nuclear protamines and proteins of the outer dense fiber and fibrous sheath. The cauda epididymidis (and proximal ductus deferens) are the regions where spermatozoa are stored before ejaculation.7 When ejaculation occurs, the stored spermatozoa with the surrounding fluid are mixed with the alkaline secretions of the male accessory sex glands and deposited in the vagina.
SPERM TRANSPORT IN THE FEMALE REPRODUCTIVE TRACT
Spermatozoa are actively transported from the vagina via the cervical canal and the uterine cavity to the ampulla of the oviducts, where fertilization occurs. In the human vagina, the ejaculated semen is deposited near the external cervical opening, where the environment is very acidic due to lactic acid and thus is hostile to spermatozoa.8 The alkaline pH of the ejaculate protects spermatozoa in this acidic environment.9 This protection is, however, temporary, and most spermatozoa only remain motile in the vagina for a few hours. Thus, the human vagina does not serve as an effective reservoir for spermatozoa and the role of the vagina in sperm transport is transitory at best. There is significant sperm loss in the vagina, and the proportion of ejaculated sperm that enter the cervical mucus in vivo is not known. The spermatozoa are transported into the cervical canal by pressure alterations in the vagina due to the female orgasm, assisted by the normal motility of sperm. Migration of spermatozoa through the cervical canal is thought to be dependent at least in part on sperm concentration, motility, and morphology and is modulated by the physiochemical characteristics of cervical mucus. The interactions between sperm and mucus and the motility of spermatozoa during transport are important; one cause of infertility is presumably impaired sperm movement through the cervical mucus.9 The change in the composition of cervical mucus at midcycle also affects the passage of sperm.10 In ovulatory mucus, sperm penetration and forward progression occur in an efficient and directional manner. In this phase of the cycle, mucin molecules are arranged in a parallel position, directing the spermatozoa into the uterus and also to the cervical crypts where they are stored.11
It is not clear whether the human female reproductive tract has the capacity to establish sperm reservoirs as do the resproductive tracts of other mammals. However, it has been shown that the release of spermatozoa from human cervical crypts may continue for several days.4 In a small percentage of women displaying no sperm in the cervical mucus, however, motile sperm can be recovered from the uterine cavity.12 The uterus seems to be a conduit to sperm transport. The transport of spermatozoa from the cervix to the uterotubal junction is mainly attributable to the myometrial contractility, ciliary movements on the surface of the endometrial cells, and intrinsic sperm motility, although the latter has been shown not to be critical.4 The human endometrium prepares for ovulation by secreting a unique kind of fluid into the uterine lumen. The fluid has a different protein pattern, ionic composition, and volume than at other stages of the cycle.13 This fluid serves to suspend spermatozoa and to keep them viable during the transport process and to remove the coating from the sperm surface as one facet of capacitation. It also contains macrophages that remove dead and nonviable spermatozoa, which in mice is probably the most important physiologic mechanism for the disposal of sperm from the uterus.14 The passage of sperm from the uterus to the fallopian tube is apparently modulated by the uterotubal junction. This segment prevents the entry of nonmotile cells and therefore acts as a selective barrier. The isthmus functions as a sperm reservoir and only a few sperm pass along the fallopian tube to the site of fertilization at any given time.11 The ampulla of the oviduct is the site of fertilization, and motile spermatozoa can be found there up to 85 hours after intercourse. The transport of spermatozoa through the oviducts is a combination of sperm motility, fluid flow, and the contractive movements of the oviduct walls.8
Sperm Capacitation
Immediately after deposition in the female genital tract, besides active motility, the sperm are not able to fertilize the oocyte. The physiologic changes that occur to sperm during their transport in the female reproductive tract are collectively referred as capacitation. Capacitation, first described in 1951 (independently by Chang in the United States and Austin in Australia), was later shown to be a requirement for fertilization. It seems that the initiation and completion of capacitation in human spermatozoa takes place in the cervix.4,9 The molecular events that initiate capacitation include removal of cholesterol from the sperm plasma membrane, increase in membrane fluidity, ion influxes resulting in alteration of sperm membrane potential, hyperpolarization of sperm membrane, increase in tyrosine phosphorylation, and changes in the adenylate cyclase-cAMP system, nucleus, and acrosome.4,15 In humans, capacitation can be mimicked in vitro in defined culture media, the composition of which is based on the electrolyte concentration of oviductal fluid. In most cases capacitation media contain energy substrates such as pyruvate, lactate, and glucose; a cholesterol receptor (such as serum albumin); NaHCO3, calcium, potassium, and physiologic sodium concentrations.
Hyperactivation and Acrosome Reaction
Capacitation, as a molecular event, occurs both in the sperm head (i.e., the acrosome reaction) and in the tail (i.e., changes in the sperm motility pattern designated as sperm hyperactivation). Sperm hyperactivation occurs before the acrosome reaction.4 The vigorous pattern of movement observed in hyperactivation is a result of physiologic changes in spermatozoa. Compared to sperm in seminal plasma, the speed, velocity, rate of flagellar beating or beat frequency, and mean beat width (lateral head displacement) are increased in capacitated sperm. This pattern of movement enables the spermatozoa to swim in the viscous oviduct fluid and to overcome the physical resistance of the three vestments—cumulus oophorus, corona radiata, and the zona pellucida—which surround the oocyte.16,17 Hyperactivation is a prognostic indicator for IVF; low levels are associated with male infertility and decreased binding to the zona pellucida.
The endpoint of capacitation is the acrosome reaction, which occurs when spermatozoa come into close contact with the oocyte in the ampullae of the oviduct. The acrosome reaction is an exocytotic event and enables spermatozoa to penetrate through the zona pellucida and fuse with the oocyte plasma membrane. Many artificial stimuli are reported to trigger the acrosome reaction, either by driving extracellular Ca2+ into the sperm cell (Ca2+ ionophores) or by acting through intracellular second messengers that are involved in the cascade leading to acrosomal exocytosis. The anterior region of the sperm head is covered by the acrosome, containing several hydrolytic enzymes, including proteases, phosphatases, arylsulfatases, and phospholipases. Hyaluronidase and acrosin have been the most extensively studied of these enzymes. The membranes surrounding the nucleus of spermatozoa are the nuclear membrane, the inner acrosomal membrane, the outer acrosomal membrane, and the plasma membrane. In this reaction, the plasma membrane and the outer acrosomal membrane fuse, enabling release of the acrosomal contents important for the events preceding fertilization.4 The acrosome reaction may facilitate recognition, adhesion, and fusion with oocytes by at least three different mechanisms: externalization of ligand proteins (e.g., CD46 on the inner acrosomal membrane); protein migration through the fluid membrane to reach binding sites, such as that for PH-20; or conformational changes of preexisting membrane proteins.18
OOCYTE TRANSPORT
The transport of oocytes from the ovarian follicles to the site of implantation has been long recognized as a fundamental step in the reproductive process in the female.19 The fallopian tubes provide the path and means of transport for the ovum from the ovary to the uterus; the duration of ovum transport is the time from its discharge from the follicle until it reaches the normal site of implantation.20 At midmenstrual cycle, approximately day 14 of an idealized 28-day cycle, the surge of pituitary luteinizing hormone (LH) results in the maturation of the oocyte by resumption of meiosis and completion of the first meiotic division. The oocyte nucleus or germinal vesicle undergoes a series of changes that involve germinal vesicle breakdown. The oocyte then enters into the second meiotic division and arrests in the second metaphase or first polar body stage. Meiosis will proceed no further unless the oocyte is fertilized. Meiotic maturation is a vital event in ovulation because it is obligatory for normal fertilization. During the process of meiotic maturation, the cumulus granulosa cells undergo mucification followed by expansion.21 The preovulatory surge of FSH appears to initiate the process of cumulus expansion. The onset of mucification is marked by a dramatic increase in the secretion of mucopolysaccharides into the extracellular spaces. This leads to the dispersal of the cumulus cells and causes the oocyte–cumulus complex to expand tremendously.
After ovulation and discharge of the follicular fluid, the oocyte–cumulus complex, consisting of an oocyte surrounded by a zona pellucida, noncellular porous layers of glycoprotein secreted by the oocyte, and granulosa cells (cumulus oophorus), is ovulated from ovarian follicles into the peritoneal cavity and is picked up by the infundibulum of the oviduct.8 The infundibulum is a highly specialized, funnel-shaped portion of oviduct (up to 10 mm in diameter) that bears long, fingerlike extensions of the mucosa (i.e., fimbriae), which sweep over the surface of the ovary; the oocyte–cumulus complex is transported by means of ciliary action along the surface of the fimbriae toward the opening of the oviduct. Cilia that cover the exterior surface of the infundibulum beat in the direction of the ostium and are important in moving the oocyte–cumulus complex into the oviduct. Oocyte pickup is not dependent on a suction effect secondary to muscle contractions, and ligation of the tube just proximal to the fimbriae in the rabbit does not interfere with pickup.22 The mucosal layer of the fallopian tube is lined with a single layer of columnar epithelium that undergoes cyclic changes in response to hormonal changes of the menstrual cycle. There are two distinct types of oviductal epithelium—ciliated and nonciliated secretory epithelium—and the ratio of these two fluctuate in response to ovarian steroids. There are fewer ciliated cells in the isthmus than in the ampullary portion of the tube, whereas they are most prominent in the fimbriated infundibulum. Ciliary activity is responsible for the pickup of oocytes by the fimbrial ostium and for movement through the ampulla, as well as the distribution of the tubal fluid, which supports gamete maturation and fertilization and facilitates gamete and embryo transport. Under the influence of the estrogens of the follicular phase of the menstrual cycle, the cilia of the isthmus beat in the direction of the ovary. At the same time, the cilia of the fimbria and the cilia of the ampulla next to the fimbria beat in the direction of the uterus. In this fashion, the ovulated oocyte will be sequestered within the middle of the ampulla. If sperm enter the uterus at this time, they will be directed into the oviduct and propelled to the awaiting oocyte.
It is within the ampulla that fertilization takes place; in humans the oocyte spends about 90% of its stay in the ampulla. After surgical reversal of tubal ligation where a large part of the ampulla was removed, excellent pregnancy rates result along with mildly increased rates of ectopic pregnancies. Pregnancy is possible after reversal of tubal ligation where complete excision of the entire ampula or fimbria was performed, but pregnancy rates are poor. After ovulation and in the presence of an increasing concentration of progesterone, the cilia and myosalpingeal contractions all pulse from the ovary toward the uterus.23 In most species, transport of the fertilized oocyte through the tube requires approximately 3 days.19 Tubal fluid is rich in mucoproteins, electrolytes, and enzymes. This fluid is abundant in midcycle when gametes or embryos are present and may play an important role during fertilization and early cleavage. Fluid in the tubes is believed to be formed by selective transudation from the blood and active secretion from the epithelial lining.
SUCCESSIVE FERTILIZATION STEPS
Fertilization is a series of steps that require immense coordination and communication between the two principal participants, the oocyte and sperm. Each gamete must undergo a series of changes before the final event of union can occur (Figs. 6-1 and 6-2).
Adhesion of Spermatozoa to Oocyte
Sperm Penetration of the Cumulus Oophorus
The cumulus oophorus is a specialized layer of granulosa cells that surrounds the ovulated mammalian oocyte. During oocyte maturation, the cumulus cell mass undergoes expansion and mucification, resulting in a well-expanded mass with an extensive amount of extracellular matrix material binding the cells together. The cumulus cells and their matrix are probably involved in several reproductive processes, including pickup of the oocyte–cumulus complex by the oviduct and increasing the chances of an encounter with one of the few sperm that have reached the ampulla of the tube.24 The extracellular matrix consists of a variety of glycoproteins and proteoglycans, with hyaluronic acid being a major component. The mechanisms of sperm penetration through the cumulus mass may involve both mechanical and chemical forces. Hyperactivated sperm and membrane-bound hyaluronidase are necessary, and perhaps sufficient, to digest a path through the extracellular matrix of the cumulus cells. The role of hyaluronidase in cumulus mass penetration is controversial because sperm remain largely acrosome-intact during in vitro penetration of the cumulus; sperm with no hyaluronidase can penetrate the cumulus mass and reach the zona pellucida.25 The role of the cumulus cell mass in fertilization is not fully understood. In human fertilization, however, a body of evidence has shown that the presence of the cumulus oophorus is beneficial for fertilization, partly by stimulation of proacrosin conversion to acrosin and initiation of the acrosome reaction. However, removal of the cumulus does not prevent sperm penetration and fertilization.
Sperm Interaction with the Zona Pellucida
Eventually after a long journey and penetration of the cumulus mass, the sperm encounters the zona pellucida, the cell type-specific extracellular matrix or coat of the oocyte where species-specific gamete recognition is believed to occur. The mammalian zona pellucida is composed of the transcripts of three highly conserved gene families named ZPA, ZPB, and ZPC. The mature products of the genes based on their deduced amino acid sequence are classified as ZP1, ZP2, and ZP3. They are synthesized during oogenesis as a product of the oocyte itself and exhibit heterogeneity because of extensive post-translational modification, including glycosylation.26 Determining the function of individual ZP proteins in humans has been difficult because of the limited availability of native human ZP, and much of the research on the zona pellucida and sperm recognition of the zona pellucida has been carried out using the mouse as a model system. In this species, the ZP3 protein serves as a sperm receptor molecule, mediating binding of the sperm to the intact zona pellucida. The currently favored model is that O-linked oligosaccharide chains on ZP3 act as a binding epitope for sperm. ZP3 is the primary ligand for sperm, whereas ZP2 in the mouse acts as a secondary sperm receptor that binds acrosome-reacted sperm and ZP1 is considered as a scaffold-like protein that appears to cross-link the ZP2 and ZP3 proteins.27 The complexity of the sperm membrane architecture, along with the difficulty of isolating and purifying it, have made the identification of the spermatozoon counterpart of the oocyte coat ligand a difficult task.
Sperm proteins thought to participate in zona binding have been identified by a range of approaches, including inhibitory monoclonal antibodies. Some of these sperm proteins have been specifically implicated as primary sperm receptors for ZP3, whereas others are thought to function as generalized adhesive proteins for the zona matrix. Thus far, among the sperm proteins thought to bind ZP3 oligosaccharides in particular, the sperm surface enzyme β1,4-galactosyltransferase-I (GalT) and two sperm membrane proteins called sp56 and sp95 are the main candidates; GalT satisfies virtually all the criteria expected of a ZP3 receptor.25,28 It presumably recognizes and binds specifically to N-acetylglucosamine residues on ZP3, an enzyme–substrate reaction in which ZP3 serves as substrate. The location of GalT, on the plasma membrane covering the dorsal aspects of the anterior region of the sperm head, is consistent with the inability of acrosome-reacted sperm to bind the zona pellucida because the plasma membrane bearing GalT is lost during the acrosome reaction.29 The binding of ZP3 to sperm activates a range of intracellular signal cascades that culminate in fusion of the plasma membrane and underlying outer acrosomal membrane (i.e., the acrosome reaction).30 The ability of ZP3 to act as an acrosome reaction inducer depends on its polypeptide chain, whereas its ability to act as a sperm receptor rests entirely on its oligosaccharide side chains. ZP3-induced exocytosis of the acrosomal contents proceeds through two sperm-signaling pathways. In the first, ZP3 binding to GalT and other potential receptors results in activation of a heterotrimeric GTP-binding protein and phospholipase C, thus elevating the concentration of cytoplasmic calcium. In the second pathway, ZP3 binding to the same receptor(s) stimulates a transient influx of calcium through T-type channels. In a later phase of the signaling, these initial ZP3-induced events produce additional calcium entry through transient receptor potential proteins, candidate subunits of the ion channels, resulting in a sustained increase in cytoplasmic calcium concentration that triggers exocytosis.24,31
Sperm–Oocyte Membrane Fusion
After sperm bind to the zona pellucida and the acrosome reacts, they penetrate the zona and enter the perivitelline space, the extracellular region between the zona and the oocyte plasma membrane where the final adhesion in the fertilization process occurs (i.e., adhesion of the sperm plasma membrane to the oocyte plasma membrane). Spermatozoa entering the perivitelline space approach the oocyte surface at an angle that lies somewhere between the vertical and the horizontal. Mammalian oocytes are spherical cells covered with microvilli before fertilization. The sperm head is like a flat dish, and the thickness of the head (∼0.2 μm) is a little less than the average distance between oocyte microvilli.32 Spermatozoa, therefore, just pass between the microvilli and make contact with the oocyte close to the cortex through the equatorial segment of the head. In the search for sperm surface proteins that function in this process, most attention has recently been given to the cysteine-rich secretory protein 1 (Crisp1) and members of the ADAM family of metallopeptidase domains (fertilin α [ADAM1], fertilin β [ADAM2], and cyritestin [ADAM3]) that function as cell adhesion molecules. The ADAM family molecules have an adhesion module, the disintegrin domain, which leads directly to the idea that oocytes have an appropriate plasma membrane adhesion partner (i.e., an integrin).33 To better assess the requirement for specific members of the ADAM family in binding (and possibly fusion), knockout mice were constructed that were null for fertilin β, cyritestin, or both (double knockout). Sperm from each of these mouse lines showed dramatically (90%) reduced binding to zona-free oocytes.34,35 Because other sperm proteins may be lost in these knockouts, this reduction in total sperm binding could possibly be caused by loss of an unidentified protein. Initial attachment of the sperm to the oocyte membrane are reversible and appear to require sperm motility. Sperm tail movement decreases or stops within a few seconds of sperm–oocyte fusion. Electron microscopy shows that the inner acrosomal membrane is later engulfed by the oocyte through a process that appears similar to phagocytosis.33 The sperm tail is also eventually incorporated into the oocyte. The adhesion of sperm to oocyte is frequently cited as likely to be analogous to the mechanism by which leukocytes interact with endothelial cells in a stepwise fashion; sperm–oocyte fusion shares common features with another classical, well-known membrane fusion event between cells and virus particles. Fusion of the sperm and oocyte membrane is followed by the cortical reaction and metabolic activation of the oocyte.
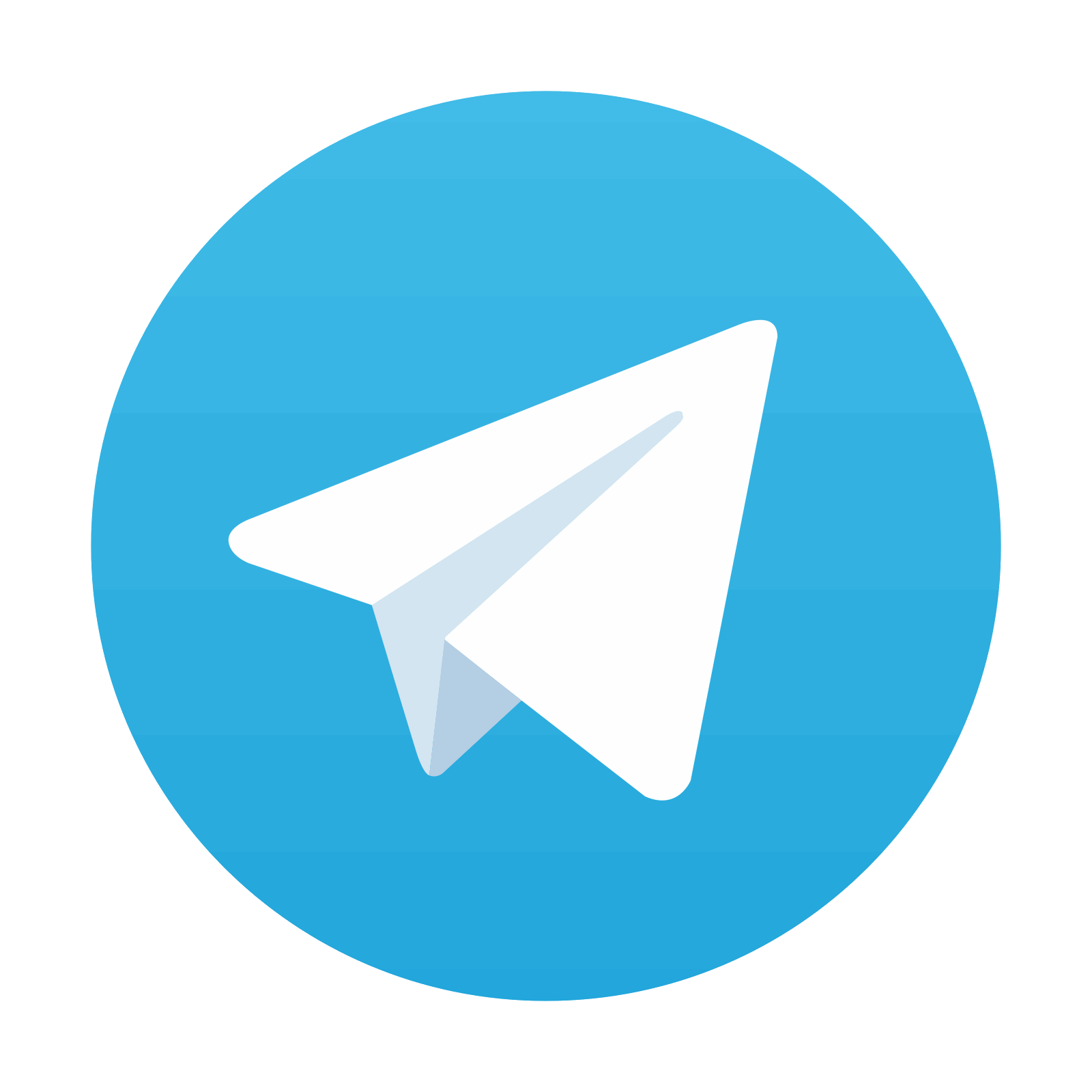
Stay updated, free articles. Join our Telegram channel

Full access? Get Clinical Tree
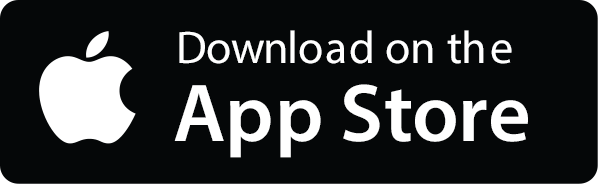
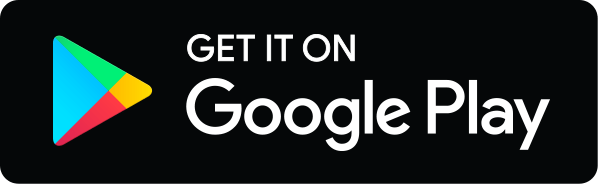